Abstract
This study aimed to investigate if glycated hemoglobin (HgbA1C) as compared to fasting blood glucose is better for reflecting cardiometabolic risk in non-diabetic Korean women. Fasting glucose, HgbA1C and lipid profiles were measured in non-diabetic women without disease (n = 91). The relationships of fasting glucose or HgbA1C with anthropometric parameters, lipid profiles, and liver and kidney functions were analyzed. Both fasting glucose and HgbA1C were negatively correlated with HDL-cholesterol (r = -0.287, p = 0.006; r = -0.261, p = 0.012), and positively correlated with age (r = 0.202, p = 0.008; r = 0.221, p = 0.035), waist circumference (r = 0.296, p = 0.005; r = 0.304, p = 0.004), diastolic blood pressure (DBP) (r = 0.206, p = 0.050; r = 0.225, p = 0.032), aspartate transaminase (AST) (r = 0.237, p = 0.024; r = 0.368, p < 0.0001), alanine transaminase (ALT) (r = 0.296, p = 0.004; r = 0.356, p = 0.001), lipid profiles including triglyceride (r = 0.372, p < 0.001; r = 0.208, p = 0.008), LDL-cholesterol (r = 0.315, p = 0.002; r = 0.373, p < 0.0001) and total cholesterol (r = 0.310, p = 0.003; r = 0.284, p = 0.006). When adjusted for age and body mass index, significant relationships of DBP (r = 0.190, p = 0.049), AST (r = 0.262, p = 0.018), ALT (r = 0.277, p = 0.012), and HDL-cholesterol (r = -0.202, p = 0.049) with HgbA1C were still retained, but those with fasting glucose disappeared. In addition, the adjusted relationships of LDL-cholesterol and total cholesterol with HgbA1C were much greater than those with fasting glucose. These results suggest that glycated hemoglobin may be a better predictor than fasting glucose for cardiometabolic risk in non-diabetic Korean women.
Diabetes mellitus (DM) is not only an important and dependent risk factor, but also a predictor of adverse outcomes for cardio-cerebrovascular diseases (i.e. coronary heart disease, stroke, heart failure, and peripheral artery disease) [1,2,3]. Glycemic measures, such as fasting glucose, 2-hour glucose, and glycated hemoglobin (HgbA1C) have been recommended for the diagnosis of diabetes and glycemic control [4,5]. Classically, fasting glucose is considered the standard measure used for the diagnosis of diabetes, and HgbA1C is recommended for the determination of long-term glycemic control in patients with DM [4,5]. In diabetic patients, increased values of HgbA1C were associated with a marked excess in cardiovascular disease (CVD) risk independent of classical CVD risk factors [6,7]. However, increased levels of HgbA1C and fasting glucose were associated with coronary artery calcification in general population without DM or cardio-cerebrovascular diseases [8].
Recently, evidences from epidemiological and clinical studies have reported that as compared to fasting blood glucose, HgbA1C was more strongly associated with the risk of atherosclerosis and CVD [9,10]. According to the earlier cross-sectional investigation within the Framingham Heart Study, normoglycemic HgbA1C (< 6.5%) may serve as a useful CVD risk marker in non-diabetic individuals [11]. Subsequent prospective studies also reported the associations of high-normal HgbA1C values with the increased risk of incident cardio-cerebrovascular diseases [3,9,10,12,13], indicating an independent relationship of HgbA1C with CVD risk in non-diabetic individuals [3,8,12,13]. In a study of 307 non-diabetic, stroke Korean male adults, the highest quartile of HgbA1C in normal range was significantly associated with the increased risk for ischemic stroke as compared to the lowest HgbA1C (≤ 5.3%) [3].
However, there were few studies comparing HgbA1C and fasting glucose as a marker reflecting cardiometabolic risk in non-diabetic Korean women. Therefore, this study aimed to investigate if HgbA1C as compared to fasting blood glucose is a better marker for reflecting cardiometabolic risk in nondiabetic Korean women.
Study participant (women) were recruited from the Health Promotion Center at Dong-A University Hospital between Januarys 2014 and March 2014. They do not have any history of diseases. Exclusion criteria were following: 1) patients diagnosed for diabetic mellitus and stroke; 2) any diagnosis of vascular disease, cancer (clinically or by anamnesis), renal disease, liver disease, thyroid disease, and acute or chronic inflammatory disease. Totally ninety-one subjects (n = 91) were recruited and agreed to the participation consent form. The written informed consent proved before participation was approved by the Institutional Review Board of Dong-a University.
Body mass index (BMI, kg/m2) was calculated using body height and weight. Blood pressure was measured in the arm of seated patients with an automatic blood pressure monitor (HEM-7220, OMRON, Matsusaka, Japan) after a rest. After overnight fasting, venous blood samples were collected in ethylenediaminetetraacetic acid (EDTA) - treated and plain tubes, separated into plasma and serum, and then stored at -80℃ until analysis.
Fasting total cholesterol and triglyceride levels were measured using commercially available kits on a Hitachi 7150 Autoanalyzer (Hitachi Ltd., Tokyo, Japan). After precipitation of serum chylomicrons with dextran sulfate magnesium, the concentrations of low-density lipoprotein (LDL) and high-density lipoprotein (HDL) cholesterol in the supernatants were enzymatically measured.
Fasting glucose levels were measured using a glucose oxidase method with a Beckman Glucose Analyzer (Beckman Instruments, Irvine, CA, USA). Glycated hemoglobin (HgbA1C) was measured using Variant II Turbo HgbA1C kit-2.0 (Bio-Rad, Hercules, CA, USA) on Variant analyzer (Variant II TURBO, Bio-Rad, Hercules, CA, USA). Serum aspartate aminotransferase (AST), alanine aminotransferase (ALT) were measured by a Modified IFCC UV method.
Statistical analyses were performed using SPSS version 21.0 for Windows (SPSS Inc., Chicago, IL, USA). Spearman and partial correlation analyses were performed for the relationships of fasting glucose or HgbA1C with basic and biochemical parameters. Skewed variables were log transformed for statistical analysis. For descriptive purposes, mean values were presented using untransformed values. Results were expressed as means ± SE or percentages. A two-tailed p value < 0.05 was considered statistically significant.
Table 1 shows general characteristics and baseline parameters such as age, body mass index (BMI), waist circumference, blood pressures, liver functions, fasting glucose, HgbA1C and lipid profiles of entire study subjects. Mean value of fasting glucose and HgbA1C were in the normal ranges (89.1 ± 1.19 and 5.26 ± 0.04, respectively).
Both fasting glucose and HgbA1C were positively correlated with age (r = 0.202, p = 0.008; r = 0.221, p = 0.035, respectively), waist circumference (r0 = 0.296, p = 0.005; r0 = 0.304, p = 0.004, respectively). As shown in Figure 1, diastolic blood pressure (DBP) was positively correlated with fasting glucose (r0 = 0.206, p = 0.050) and HgbA1C (r0 = 0.225, p = 0.032). AST and ALT, the liver function markers, were also positively correlated with fasting glucose (r0 = 0.237, p = 0.024; r0 = 0.296, p = 0.004, respectively) and HgbA1C (r0 = 0.368, p < 0.0001; r0 = 0.356, p = 0.001, respectively). When adjusted for age and BMI, significant relationships of DBP (r1 = 0.190, p = 0.049), AST (r1 = 0.262, p = 0.0018), ALT (r1 = 0.277, p = 0.012) with HgbA1C were still retained, but those with fasting glucose disappeared.
Figure 2 presents relationship between lipid profiles and fasting glucose or HgbA1C. Both fasting glucose and HgbA1C were positively correlated with triglyceride (r0 = 0.372, p < 0.001; r0 = 0.208, p = 0.008, respectively), LDL-cholesterol (r0 = 0.315, p = 0.002; r0 = 0.373, p < 0.0001, respectively) and total cholesterol (r0 = 0.310, p = 0.003; r0 = 0.284, p = 0.006, respectively), and negatively correlated with HDL-cholesterol (r0 = -0.287, p = 0.006; r0 = -0.261, p = 0.012, respectively). Further adjustment for age and BMI still maintained the significant positive correlation of fasting glucose or HgbA1C with total cholesterol (r1 = 0.232, p = 0.038; r1 = 0.265, p = 0.017, respectively) and LDL-cholesterol (r1 = 0.247, p = 0.026; r1 = 0.400, p < 0.0001, respectively), but not with triglyceride (r1 = 0.117, p = 0.115; r1 = 0.181, p = 0.115, respectively). In addition, the negative relationship between HDL-cholesterol with HgbA1C (r1 = -0.202, p = 0.029) were still maintained but those with fasting glucose disappeared (Figure 2).
The aim of this study was to investigate if HgbA1C as compared to fasting blood glucose is better for reflecting cardiometabolic risk in non-diabetic Korean women. Study result shows that both fasting glucose and HgbA1C were negatively correlated with HDL-cholesterol and positively correlated with DBP, AST, ALT, triglyceride, LDL-cholesterol and total cholesterol. When adjusted for age and BMI, significant relationships of DBP, AST, ALT, and HDL-cholesterol with HgbA1C were still maintained, but those with fasting glucose disappeared. In addition, the adjusted relationships of LDL-cholesterol and total cholesterol with HgbA1C were much greater than those with fasting glucose. These results may suggest that HgbA1C as compared to fasting glucose is more sensitive for reflecting cardiometabolic risk in non-diabetic Korean women, thereby being a more predictable parameter for cardiometabolic risk in non-diabetic condition.
Many of epidemiological and clinical studies have reported increased lipid abnormalities in uncontrolled diabetic individuals, which contribute to higher rates of CVD [9,10]. Therefore, lipid management aimed at lowering LDL cholesterol and triglycerides, and raising HDL cholesterol has been applied to reduce CVD risk and mortality in type 2 diabetes [14]. As reported, insulin is a potent anti-lipolytic hormone and restrains lypolysis (release of free fatty acids, FFA) from the adipocytes by inhibiting the enzyme lipase. Fat cells in patients with type 2 DM or with metabolic syndrome (MetS, i.e. impaired glucose status, dyslipidemia, higher blood pressure, obesity) are resistant to the inhibitory effect of insulin on lipolysis [15]. This leads to a release of large amounts of FFA into the blood, thereby the increased FFAs producing lipid abnormalities mainly such as increased triglycerides and decreased HDL which causes atherogenic dyslipidemia [16]. In our study, both fasting glucose and HgbA1C were negatively correlated with HDL-cholesterol and positively correlated with triglyceride, LDL-cholesterol and total cholesterol. When adjusted for age and BMI, the significant relationships of LDL-cholesterol and total cholesterol with fasting glucose or HgbA1C were still retained, but the relationship between HDL-cholesterol and fasting glucose disappeared. This may indicate that HgbA1C as compared to fasting glucose is more sensitive to reflect cardiometabolic risk in non-diabetic Korean women.
In our study, DBP was positively associated with HgbA1C and the relationship was still maintained after adjusted for age and BMI. It may be partly explained by the report that dyslipidemia and hyerpinsulinemia in DM are associated with vascular dysfunction (i.e. increased vascular smooth muscle cell proliferation, arterial stiffness and vascular tone, and decreased vasodilation) and sodium retention which cause hypertension [17].
In addition, our study shows that AST and ALT the liver function markers were significantly associated with fasting glucose and HgbA1C levels. Particularly, the relationships with HgbA1C were still retained after adjusted for age and BMI. Our result is partly in accordance with the report by Fraser et al. [18] and Kim et al. [19]: when ALT and gamma-glutamyltransferase (GGT) levels were subdivided into tertiles, the levels of fasting insulin, fasting glucose and HgbA1C were higher in the highest terile of ATL and GGT levels in both non-diabetic and diabetic, although the associations of ALT and GGT with fasting glucose and HgbA1C were stronger in women with DM compared to those without DM [18]. Kim et al. also reported that the significant association between serum levels of GGT and the presence of the MetS even after adjusted for the presence of fatty liver, suggests that another mechanism governs the relationship between serum GGT and MetS [19].
This study has limitations. First, it did not allow for the sequential assessment of a direct association between fasting glucose or HgbA1C and cardiometabolic risk in non-diabetic individuals, because the exposure and outcome data were collected at a single time point. Second, only age and BMI (one of representative parameters for degree of obesity) were used as the adjustment parameters in partial correlation analysis. If other confounding factors (i.e. body fat percentage or distribution etc.) were additionally measured and adjusted, the conclusion would be more strongly supported.
Figures and Tables
Figure 1
Relationships of blood pressure and liver function markers with fasting glucose or glycated Hemoglobin (HgbA1C) tested by Spearsman and partial correlation analysis, ø: log-transformed r0: correlation coefficient (unadjusted), r1: correlation coefficient (adjusted for age and body mass index); (A) presents corrleation between diastolic BP and fasting glucose or HgbA1C, (B) presents corrleation between AST and fasting glucose or HgbA1C, and (C) presents corrleation between ALT and fasting glucose or HgbA1C; AST: aspartate aminotransferase, ALT: alanine aminotransferase, BP: blood pressure.
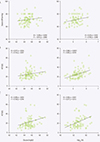
Figure 2
Relationships of serum fasting lipid profiles with fasting glucose or glycated hemoglobin (HgbA1C) tested by Pearson and partial correlation analysis, ø: log-transformed r0: correlation coefficient (unadjusted), r1: correlation coefficient (adjusted for age and body mass index); (A) presents corrleation between triglyceride and fasting glucose or HgbA1C, (B) presents corrleation between total cholesterol and fasting glucose or HgbA1C, (C) presents corrleation between LDL-cholesterol and fasting glucose or HgbA1C, and (D) presents corrleation between HDL-cholesterol and fasting glucose or HgbA1C.

Table 1
General characteristics and biochemical parameters of study women
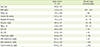
Acknowledgements
We thank the research volunteers who participated in the studies described in this manuscript. This study was supported by the Basic Science Research Program through the National Research Foundation of Korea funded by the Ministry of Science, ICT and Future Planning (2013R1A1A3011535), and fellowships for students were partly supported by the Brain Busan 21 project.
References
1. Swinburn BA, Sacks G, Hall KD, McPherson K, Finegood DT, Moodie ML, Gortmaker SL. The global obesity pandemic: shaped by global drivers and local environments. Lancet. 2011; 378:804–814.


2. Malmberg K, Norhammar A, Wedel H, Rydén L. Glycometabolic state at admission: important risk marker of mortality in conventionally treated patients with diabetes mellitus and acute myocardial infarction: long-term results from the Diabetes and Insulin-Glucose Infusion in Acute Myocardial Infarction (DIGAMI) study. Circulation. 1999; 99:2626–2632.


3. Oh HG, Rhee EJ, Kim TW, Lee KB, Park JH, Yang KI, Jeong D, Park HK. Higher glycated hemoglobin level is associated with increased risk for ischemic stroke in non-diabetic Korean male adults. Diabetes Metab J. 2011; 35:551–557.


4. American Diabetes Association. Standards of medical care in diabetes--2014. Diabetes Care. 2014; 37:Suppl 1. S14–S80.
5. Selvin E, Crainiceanu CM, Brancati FL, Coresh J. Short-term variability in measures of glycemia and implications for the classification of diabetes. Arch Intern Med. 2007; 167:1545–1551.


6. Greenland P, Alpert JS, Beller GA, Benjamin EJ, Budoff MJ, Fayad ZA, Foster E, Hlatky MA, Hodgson JM, Kushner FG, Lauer MS, Shaw LJ, Smith SC Jr, Taylor AJ, Weintraub WS, Wenger NK, Jacobs AK, Smith SC Jr, Anderson JL, Albert N, Buller CE, Creager MA, Ettinger SM, Guyton RA, Halperin JL, Hochman JS, Kushner FG, Nishimura R, Ohman EM, Page RL, Stevenson WG, Tarkington LG, Yancy CW. American College of Cardiology Foundation. American Heart Association. 2010 ACCF/AHA guideline for assessment of cardiovascular risk in asymptomatic adults: a report of the American College of Cardiology Foundation/American Heart Association Task Force on Practice Guidelines. J Am Coll Cardiol. 2010; 56:e50–e103.
7. International Expert Committee. International Expert Committee report on the role of the A1C assay in the diagnosis of diabetes. Diabetes Care. 2009; 32:1327–1334.
8. Jung CH, Rhee EJ, Kim KJ, Kim BY, Park SE, Chang Y, Ryu S, Park CY, Mok JO, Oh KW, Kim CH, Park SW, Kang SK, Lee WY. Relationship of glycated hemoglobin a1c, coronary artery calcification and insulin resistance in males without diabetes. Arch Med Res. 2015; 46:71–77.


9. Levitan EB, Liu S, Stampfer MJ, Cook NR, Rexrode KM, Ridker PM, Buring JE, Manson JE. HbA1c measured in stored erythrocytes and mortality rate among middle-aged and older women. Diabetologia. 2008; 51:267–275.


10. American Diabetes Association. Diagnosis and classification of diabetes mellitus. Diabetes Care. 2004; 27:S5–S10.
11. Thanopoulou A, Karamanos B, Archimandritis A. Glycated hemoglobin, diabetes, and cardiovascular risk in nondiabetic adults. N Engl J Med. 2010; 362:2030–2031.


12. Haring R, Baumeister SE, Lieb W, von Sarnowski B, Völzke H, Felix SB, Nauck M, Wallaschofski H. Glycated hemoglobin as a marker of subclinical atherosclerosis and cardiac remodeling among non-diabetic adults from the general population. Diabetes Res Clin Pract. 2014; 105:416–423.


13. Cai A, Li G, Chen J, Li X, Wei X, Li L, Zhou Y. Glycated hemoglobin level is significantly associated with the severity of coronary artery disease in non-diabetic adults. Lipids Health Dis. 2014; 13:181.


15. Smith U. Impaired ('diabetic') insulin signaling and action occur in fat cells long before glucose intolerance--is insulin resistance initiated in the adipose tissue. Int J Obes Relat Metab Disord. 2002; 26:897–904.


16. Mooradian AD. Dyslipidemia in type 2 diabetes mellitus. Nat Clin Pract Endocrinol Metab. 2009; 5:150–159.


17. Mugo MN, Stump CS, Rao PG, Sower JR. Hypertension and diabetes mellitus. In : Black HR, Elliott WJ, editors. Hypertension: a companion to Braunwald's heart disease. Philadelphia (PA): Elsevier Saunders;2007. p. 409.