Abstract
Background
The purpose of this study is to compare the efficacy and safety of multisession radiosurgery to those of single dose radiosurgery for metastatic brain tumors.
Methods
Between February 2008 and February 2012, 90 patients with 196 metastatic brain tumors were treated with cyberknife radiosurgery, and we reviewed these patients retrospectively. Among them, 57 patients underwent single dose radiosurgery, and 33 patients multisession radiosurgery. Tumors involving the eloquent area and large tumors (>5 cc) were treated with multisession radiosurgery. The median tumor volume and the median treatment dose of single dose radiosurgery were 2.05±0.72 cc and 19.76±1.54 Gy respectively, and in the case of multisession radiosurgery, 5.30±1.70 cc and 29.6±1.70 Gy respectively. The frequency of multisession dose was 3 to 5 times, on average 3.55 times, and 8.91 Gy were given per 1 session on average.
Results
The overall survival (OS) of multisession radiosurgery was 16.0 months, whereas that of single dose radiosurgery was 11.5 months. The radiologic tumor response rates were 90% in single dose radiosurgery and 95.4% in multisession radiosurgery, respectively. Over 6-month and 1-year periods, the OS rates of single dose radiosurgery were 71.4% and 44.9%, whereas those of multisession radiosurgery were 69.1% and 58.3%, respectively (p=0.83). Toxicities were seen in 18.1% in the single dose radiosurgery group versus 4% in the multisession radiosurgery group. The difference was significant (p<0.05).
Conclusion
In this study, the multisession radiosurgery group, despite the location and size constraints, did not differ from the single dose radiosurgery group when comparing the survival and recurrence rates, but complications and toxicity were lower. Thus, multisession radiosurgery is thought to be beneficial for treatment of large tumors and tumors located in the eloquent area.
Metastatic brain tumors are the most common intracranial tumors; they account for 25% to 35% of all cancer patients and are a common cause of morbidity and mortality in patients with solid tumors [1]. Various treatment options have been developed for metastatic tumors, such as surgical resection, whole brain radiation therapy (WBRT), stereotactic radiosurgery such as Gamma Knife and Cyberknife, and intensity modulated radiation therapy [2]. One such option is Cyber-knife (Accuracy, Sunnyvale, CA, USA), an image-guided frameless, real-time robotic radiosurgery system that has proven its efficacy in prospective and randomized trials [3]. It has been increasingly used to treat brain metastases as it avoids many of the side effects of whole brain radiotherapy as well as the need for invasive surgery [45]. The benefits of Cyberknife include more accurate target localization and better dose delivery for the management of metastatic brain tumors [6]. In addition, Cyberknife is highly recommend in certain situations since it is minimally invasive, and therefore has lower risk. It can be combined with other methods, and can also be an alternative to other methods depending on the patient status and tumor location. Cyberknife is available on an outpatient basis [78], is capable of treating multiple lesions simultaneously, and can be used for repeated treatments in cases of regional or local metastasis [91011]. To date, the local malignant tumor control rate for Cyberknife is approximately 83%, which is comparable to other radiosurgery systems [12]. Although non-invasive frameless stereotactic radiosurgery such as Cyberknife has become increasingly popular, the majority of papers about radiosurgery have been on frame-based stereotactic radiosurgery. The therapeutic impact of Cyberknife is still unclear since there are few published papers on this treatment method. There are few papers that compare single dose and multisession radiosurgery. We compared the efficacy and complications of single radiosurgery and multisession radiosurgery using Cyberknife in a single institute.
Between February 2008 and February 2012, we retrospectively analyzed 196 lesions from 90 patients with metastatic brain tumors that had been treated with Cyberknife. Patient data of this study was approved by the Institutional Review Board (IRB No.: SCHUH 2015-05-001). The numbers of patients who had been treated by single dose radiosurgery and multisession radiosurgery were 57 (63.3%) and 33 (36.7%), and the numbers of those with multiple tumors were 29 (50.9%) and 9 (27.3%), respectively. Over 95% had Karnofsky performance status (KPS) scores above 70 in both the single dose radiosurgery and multisession radiosurgery groups (54 vs. 32). The number of patients who had previously had surgery was 11 (19.3%) in the single dose radiosurgery group and 13 (39.4%) in the multisession radiosurgery group. Previous WBRT was done in 18 patients (31.6%) in the single dose radiosurgery group and 12 (36.4%) in the multisession radiosurgery group. Sixteen (28.1%) patients in the single dose radiosurgery group had systemic chemotherapy, while 11 (33.3%) in the multisession radiosurgery group (Table 1). Among the 57 patients who had single dose radiosurgery, the tumor origin was non-small cell lung cancer (NSCLC) in 35 patients (61.4%), whereas only 9 out of 33 patients (27.3%) who had multisession radiosurgery had NSCLC as their primary tumor (Table 2).
Tight thermoplastic masks were applied for immobilization, and for all patients, we made treatment plans and did follow-up research, using thin-cut Helical CT with slice thickness of 1-2 mm, fused with Gadolinium-enhanced MRI scans. Planned target volume was derived by adding a 1 mm margin around the gross tumor volume with dose-limiting structures, such as eyes, optic nerve, optic chiasm and brain stem being properly delineated. Median tumor volume in the single dose radiosurgery group was 2.05±0.72 cc (range: 0.14-4.99 cc) and 5.30±1.70 cc (range: 0.77-50.49 cc) in the multisession radiosurgery group (p=0.026). Median treatment dose was 19.76±1.54 Gy (range: 10-25 Gy) and median isodose line was 79.88% (range: 42-88%) in the single dose radiosurgery group. Median treatment dose was 29.6±1.70 Gy (range: 21-30 Gy) and median isodose line was 83.48% (range: 77-83%) in multisession radiosurgery group. The mean number of sessions in the multisession radiosurgery group was 3.55 (range of 3 to 5 times) at 8.19 Gy per one session. We defined biological effective dose (BED) as nd (1+d/α/b), where n is the number of sessions, d is the daily dose, the unit of measurement was gray (Gy), and the assumed α/b ratio was 10. Multinomial logistic models were used to control for 2 potential confounding variables: BED and initial tumor volume. Treatments were delivered in a variable number of multisessions, and the relative BED in the single dose radiosurgery group compared with that in the multisession radiosurgery group had to be taken into account. The mean BED prescription dose, for an α/b ratio of 10, in the single dose radiosurgery group was significantly greater than that in the multisession radiosurgery (57.6 Gy vs. 48.7 Gy, respectively; p<0.001). Dosimetric parameters are summarized in Table 3.
After the Cyberknife procedures, we conducted serial examinations including neurologic and radiologic tests. Follow-up MRI scans were routinely conducted every 3 months, and additional scans were done in patients who developed unexpected neurological deteriorations. Systemic functional status was measured with KPS score and recursive partitioning analysis (RPA) class. Volumetric response was measured using gadolinium enhanced MRI, which helped in outlining the tumors to check the volume. The data were uploaded to our Dong-En information technology Picture Archiving and Communication System. Total volume was calculated by multiplying the area of one plane to slice thickness. Response criteria were defined as complete response (CR) when the tumor was completely gone, partial response (PR) for tumor reduction over 50%, progression of disease (PD) if the tumor became larger by at least 25%, and stable disease (SD) for tumor response pattern that did not meet any of the other criteria. Local progression (LP) is defined as enlargement of the previously treated tumor, and regional progression (RP) is defined as a tumor that is newly discovered elsewhere. Toxicity was graded according to the National Cancer Institute Common Terminology Criteria for Adverse Event (version 4.0), which defined Grade 1 as asymptomatic or mild symptoms that do not require intervention, such as headaches; Grade 2 as symptoms that require some medications; Grade 3 as symptoms that are not immediately life-threatening but require hospitalization; Grade 4 as life-threatening or requiring urgent intervention; and Grade 5 as fatal. Survival rate was considered from the date the treatment commenced to the last follow-up date. For those patients who died, we considered the date of death as the last follow-up date. The Kaplan-Meier Method was used for statistical analysis. Univariate log-rank tests and multivariate Cox regression analyses were used to compare prognostic variables that were necessary for survival length extension. SPSS statistical software (version 14.0; SPSS Inc., Chicago, IL, USA) was used for statistical analysis. The causes of deaths were obtained from medical records and the opinions of physicians. Phone calls were made when more detailed information was needed.
The mean follow-up periods for the single dose radiosurgery group and multisession radiosurgery groups were 10.4 months (range: 3.0-33 months) and 11.1 months (range: 3.2-24 months), respectively. Overall survival (OS) in the single dose radiosurgery group was 11.5 months, and 16.0 months in the multisession radiosurgery group. OS rates at 6 months and 1 year were 71.4% and 44.9%, respectively in the single dose radiosurgery group, while in the multisession radiosurgery group they were 69.1% and 58.3%, respectively (p=0.83) (Fig. 1). In the case of breast and colon cancer in the multisession radiosurgery group, their ratios are 27.3% and 33.3%, respectively, which are high when compared to the single dose radiosurgery group. There was no statistically significant correlation between the two groups (p=0.81) (Table 2).
Tumor responses were evaluated in 66 patients, except for 24 patients who were lost during follow-up. Distribution of tumor responses in the single dose radiosurgery group was CR, PR, SD, and PD in 6 (13.6%), 21 (47.7%), 13 (29.5%), and 4 (9.1%), respectively. If we regarded CR, PR and SD as tumor responsiveness to treatment, then the overall tumor response was 40 (90.0%). Distribution of tumor responses in the multisession radiosurgery group was CR, PR, SD, and PD in 5 (22.7%), 9 (40.9%), 7 (31.81%), and 1 (4.5%), respectively. The overall tumor response in the multisession radiosurgery group was 21 (95.45%). Even though the tumor response rate was better in the multisession radiosurgery group, the difference was not statistically significant (Table 4). In the single dose radiosurgery group, clinical improvement was seen in 4/44 (9%) of cases, no change in 33/44 (73%) and deterioration in 8/44 (18%). In the multisession radiosurgery group, clinical improvement, no change and clinical deterioration were seen in 2/22 (9%), 19/22 (86%), and 1/22 (4%), respectively. In detail, headache improved in 3 patients and 2 patients, respectively, followed by dizziness and hemiparesis. In the single dose radiosurgery group, there were 8 patients who showed clinical deterioration. They were dizziness (1), headaches (4), general weakness (1), lethargy (1), and hemiparesis (1). None of the results took the radiologic response into account. Patients who had dizziness and headache were hospitalized because the symptoms could not be controlled by medication. Clinical response in the multisession radiosurgery group was 95% versus 82% in the single dose radiosurgery group. Even though the results may not be statistically significant, the multisession radiosurgery group did get better results than the single dose radiosurgery group. Table 5 represents clinical deterioration, radiologic progression and neurologic death. In the multisession radiosurgery group, one patient who showed radiologic progression resulted in neurologic death because of clinical deterioration. In the single dose radiosurgery group, 8 patients deteriorated clinically. Among the 8 patients, 4 patients' tumor sizes increased radiologically (radiologic progression), 3 patients' tumor sizes were stable (SD) (2 of these received hospital treatment because of uncontrolled headaches, and 1 for dizziness), and 1 patient's tumor size decreased radiologically (PR). In these cases, 4 patients passed away due to neurologic reasons (Table 5). Of the 4 patients whose tumors did not increase in size, 3 patients had SD (3 cases) and 1 patient had PR (1 case); all of the 4 patients' brain edema increased in size. Of the 8 patients who deteriorated clinically, 3 patients had received radiation therapy previously.
Among the 44 patients who had single dose radiosurgery group, 21 (47.7%) and 9 (20.5%) showed regional and LP, respectively. Among the 22 patients in the multisession radiosurgery group, 7 (31.8%) and 5 (22.7%) had RP and LP, respectively. The numbers of patients who reached RP and LP without recurrence of tumors in the single dose radiosurgery group were 5 (11.3%), and 9 (20.5%), respectively. In the multisession radiosurgery group, the numbers were 4 (18.2%) and 6 (27.3%). In the single dose radiosurgery group especially, 20 patients had more than 3 tumors; among them (excluding 5 patients who were not followed up on), 8 patients had LP. In the multisession radiosurgery group, all 3 patients had LP. In the single dose radiosurgery group, the RP-free survival rate was 70.5% at 6 months and 47.6% at one year; and in the multisession radiosurgery group, they were 63.8% and 43.9% (p=0.30). LP-free survival rates were 80% and 69% at 6 months and 1 year in the single dose radiosurgery group, and were 92% and 70% in the multisession radiosurgery group (p=0.42), which tells us that both are irrelevant to regional and local free survival rate (Fig. 2). Many factors affecting OS were investigated. The KPS score, RPA class, and previous radiation therapy were associated with survival based on univariate analysis. The KPS score and RPA class were associated with survival based on multivariate analysis (Table 6).
Neurologic symptoms such as seizure were managed with steroids or anticonvulsants, and most of the symptoms subsided. There were 8 cases (18.1%) of adverse events in the single dose radiosurgery group; common terminology criteria for adverse events (CTCAE) grade 1 in 3 (6.8%), CTCAE grade 2 in 3 (6.8%), CTCAE grade 3 in 1 (2.27%), and CTCAE grade 4 in 1 (2.27%). There was 1 CTCAE grade 4 case (4%) in the multisession radiosurgery group. Toxicities were seen in 18.1% in the single dose radiosurgery group versus 4% in the multisession radiosurgery group. The difference was significant (p<0.05) (Table 7). Of the 22 patients in the multisession radiosurgery group that were successfully followed up on, 18 (80%) died of systemic causes, 1 (5%) died of neurologic causes, and the other patients died for unknown reasons. We analyzed the cause of death in 44 patients in the single dose radiosurgery group that was followed up on. In the single dose radiosurgery group, neurologic death was seen in 4 (8%) patients, systemic cause of death was seen in 30 (69%) patients, and death for unknown reasons was seen in approximately 23%.
Stereotactic radiosurgery has emerged as a treatment for brain metastases in the last 20 years. Stereotactic radiosurgery precisely delivers a single high dose of radiation to a small target while sparing surrounding tissue. It is generally considered suitable for metastases <3 cm3 in size that are radiographically discrete. Single dose radiosurgery group can control multiple brain metastases without the neurological side effects of WBRT or the need for invasive surgery [13].
A large retrospective study of patients with breast cancer undergoing radiosurgery showed that the number of brain metastases did not influence survival, whereas a total treated volume <3 cm3 was significantly associated with improved survival in univariate and multivariate analysis [14]. This may be because larger lesions are harder to control with radiation, since the total safe dose is lower. Furthermore, larger metastases may also have more aggressive biology. For instance, they may represent a group with higher-than-average growth rates and a more infiltrative growth pattern, making single dose radiosurgery group less effective without a bigger margin. The total volume of disease may also be important because, as volume increases, pressure effects and associated neurological dysfunction will also increase. This would frequently correlate with a deterioration in performance status and increase the risk of neurological death. One study is the Radiation Therapy Oncology Group which was published in 2000. This paper presented that acute toxicities increased as the treatment dose was increased and that dose reduction was associated with a decreasing local control rate [15]. Thus, much effort was devoted to reducing acute toxicities while improving the local control rate with large-sized tumors. Multisession radiosurgery was suggested as a solution to this dilemma. Multisession radiosurgery technique combines the advantages of single radiosurgery and conventional radiation therapy (CRT). Single radiosurgery offers spatial precision and high-dose radiation, and CRT offers the sparing effect of fractionation as well as potential application to large targets. Multisession radiosurgery technique, especially applied to large target, had reduced toxicities and escalated the dose and efficacy [16]. Thus, multisession radiosurgery can be performed on a large sized tumor, on a lesion in the eloquent area or adjacent to cranial nerves, or on a mass requiring re-irradiation.
A paper that compares single radiosurgery and multisession radiosurgery showed that 376 metastatic brain tumors in 85 renal carcinoma patients treated with single dose radiosurgery group alone (tumor dose; 21.2±3.2 Gy) produced a mean survival time of 11.1 months and a 94% local control rate, with few side effects [17]. According to Wang et al. [18], treating 72 metastatic brain tumor patients with 10-36 Gy, in two to five multisession, resulted in a tumor control rate of 95%, and a radiation damage rate close to 4%. Ernst-Stecken et al. [19] compared 51 patients treated with multisession radiosurgery for tumors over 3 cc, or with some critical structures nearby, to patients treated with through Phase II trials. They gave 30 Gy in 5 sessions. The median survival time was 11 months, and 1-year local progression-free survival (LPFS) was 76%. They reported that multisession was more effective and safer than single dose radiosurgery group. Aoyama et al. [20] investigated 87 patients treated with 35 Gy, in 4 sessions. Their median survival was 8.7 months, 1 year LPFS was 81%, and 4 (5%) cases were affected by toxicity. Manning et al. [3] prospectively analyzed 32 metastatic brain tumor patients who received 3-session treatment of 27 Gy. Their median survival was 12 months. Four (13%) of them had seizures in follow-up MRI, and 2 (6%) had clinical radio-necrosis after treatment. According to the research done for this article, there was no significant difference in the patient control group between single dose radiosurgery group and multisession radiosurgery groups, except for previous surgery. We were able to obtain 7 papers that compared the efficacy and toxicity of single dose radiosurgery group and multisession radiosurgery from 2006 to 2011. Almost all of the papers reported that multisession radiosurgery was more effective and less toxic. The published papers about multisession radiosurgery are summarized in Table 5.
Speaking of rates of previous surgery, in our series, it was 39.4% (13/33) in the multisession radiosurgery group, which means that there is a slightly significant difference from the single dose radiosurgery group group's rate of 19.3%. The metastatic brain tumors in the multisession radiosurgery group were bigger in size than the single lesions. Even though the tumors were located in a critical area, tumor resection was the first treatment to consider. Looking at the types of tumors in the 90 patients who had Cyberknife therapy, NSCLC was the most common. Breast cancer and colon cancer were relatively more common in the multisession radiosurgery group, but there was no significant difference, as in the case of other papers. The median survival was similar at 11.5 months in the single dose radiosurgery group and 16.0 months in the multisession radiosurgery group, with p value equal to 0.83. Survival seems to be related to pretreatment prognostic factors rather than stereotactic surgery itself, and with less toxicity in the multisession radiosurgery group. There was a statistically significant increase in the median survival of the multisession radiosurgery patients compared with the single dose radiosurgery group patients, despite the smaller calculated BED in the multisession radiosurgery group. Even though there were differences between the tumor size ranges of 2.45±0.93 cc versus 2.05±0.72 cc, the survival rates and recurrence rates were similar in the single dose radiosurgery group and multisession radiosurgery groups. According to the survey, age, KPS score, RPA class, and previous radiotherapy turned out to be relevant factors in the log rank test, which proved that the patient's status before the treatment affected the survival rate and prognosis after the treatment. Preoperative status can be the factor most influential to survival rate. Most of the papers announced that, even though previous WBRT did not correlate to survival, it remarkably decreased intracranial relapse and the need for salvage brain treatment [2]. However, Stafinski et al. [21] published a paper which set forth that in the case of 1 brain metastasis, additional WBRT compared with single radiosurgery increased 24 month tumor control rate and improved survival. In this research, previously-received radiotherapy was meaningful in logrank tests which did not consider other factors. However, it was not significant in Cox regression analysis which considered various factors. These results were caused by not looking at extracranial metastasis, number of tumors, etc. Also in this research, 20 patients had more than 3 tumors in the single dose radiosurgery group, and 5 patients were excluded because of inability to follow-up. In the remaining 15 patients, 8 patients were found to have new metastasis. The new metastasis of 8 patients in the single dose radiosurgery group and in all 3 of the patients in the multisession radiosurgery group reflects the correlation between the number of tumors and new metastasis. With regard to the radiologic response, if CR, PR, and SD were considered tumor response, it showed a difference of 95% versus 82%, and lack of a statistically-significant difference notwithstanding, in terms of clinical response, multisession suggested the better result. More research is needed on whether multisession actually reduced acute complications more effectively in the treatment of tumors.
See Table 4: after radiosurgery, radiologic response is also important, but brain edema has a decisive effect on the clinical results. In the single dose radiosurgery group, 4 patients had radiologic progression. Among them the first follow-up date was more than 10 months later in 2 patients.
This result shows that it is possible, if the first follow-up date had been less than 10 months in 2 patients, that the patients would have been radiologically classified in the "stable disease" or "partial response" group regarding tumor size. Also, in general, 1 case would have been expected to progress because a small cell lung cancer case, which has poor response to radiotherapy, was irradiated. The local-progression-free survival rates at 6 months and 1 year were 80% and 69%, respectively, in the single dose radiosurgery group; while they were 92% and 70%, respectively, in the multisession radiosurgery group (p=0.42). There was no significant difference between the two groups. Toxicities were seen in 18.1% of the single dose radiosurgery group, significantly more than the 4% seen in the multisession radiosurgery group (p value<0.05). Most of the toxicities were headaches and there was one case of neurologic problems such as hemiparesis. The patients who reported hemiparesis had these symptoms prior to radiosurgery, rendering it difficult to determine whether the treatment itself caused the symptoms, and acute toxicity affected just one case in the multisession radiosurgery group, showing that multisession obviously had fewer toxic effects than single dose radiosurgery group. If the toxicity level is lower in the multisession radiosurgery group, and the tumor is bigger and located in a critical area, it would be beneficial to perform multisession radiosurgery instead.
Although potential bias existed because we retrospectively analyzed a small sample, we were able to compare the results of single dose radiosurgery group and multisession radiosurgery using Cyberknife in a single center. Since there were no articles to refer to, a single-center comparative study has its value in contributing to consensus regarding toxicity criteria and radiation technique. In addition, we adjusted for differing multisession schedules by calculating for the BED using an assumed α/b ratio of 10. We recognize that different histological types will have varying ratios, but a value of 10 was chosen for the purposes of this calculation. The limitation of this research is that 10 Gy is generally a small quantity for metastatic brain tumors. Since 2008, when WBRT began with Cyberknife, there were several cases which were irradiated with a 10-Gy single dose for palliative treatment, and 1 case in which the patient had 12 tumors treated with Cyberknife. We consider that with Cyberknife, dose and the number of tumors would create bias in results of toxicity evaluation.
In conclusion, despite the issues related to tumor size and location, which can be the biggest sources of bias in comparing single dose radiosurgery group and multisession radiosurgery, there was no significant difference in LPFS between the two groups. Moreover, toxicity was less frequently observed in the multisession radiosurgery group than the single dose radiosurgery group.
In this study, there are a number of limitations, such as the following. Many of prognostic factors possibly affecting the clinical outcomes have not been balanced by use of the eligibility criteria. The effect of extent of surgical resection was not analyzed. Continued studies should be done to find optimal dosage and number of multisession radiosurgery sessions.
Figures and Tables
Fig. 1
Kaplan-Meier curve demonstrating SRS and MSR survival rates, respectively. Median survival rate was 71.4%, 44.9% in the SRS group (1) and 69.1%, 58.3% in the MSR group (2) at 6 months and 1 year (p=0.83). SRS, single-dose radiosurgery; MSR, multisession radiosurgery, OS, overall survival.
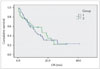
Fig. 2
Local progression-free survival rates at 6 months and 1 year were 80% and 69%, respectively, for the single-dose radiosurgery (SRS) group and 92% and 70%, respectively, for the multisession radiosurgery (MRS) group (p=0.42). LPFS, local progression-free survival.
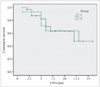
Table 1
Patient characteristics
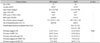
Table 2
Distribution of tumor type in 90 patients
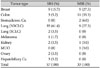
Table 3
Dosimetric parameters

Table 4
Relationship of clinical deterioration and radiologic progression, neurologic death
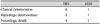
SRS | MSR | |
---|---|---|
Clinical deterioration | 8 | 1 |
Radiologic deterioration | 4 | 1 |
Neurologic death | 4 | 1 |
Table 5
Summary of studies analyzing efficacy of multisession radiosurgery for patients with brain metastases
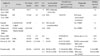
MTV, mean tumor volume; LPFS, local progression-free survival; CK, cyberkinfe; LINAC, linear accelerator; IGRS, image-guided radiosurgery; SRS, single-dose radiosurgery; NR, not reported; MSR, nultisession radiosurgery; FSRT, fractionated stereotactic radiotherapy; KPS, Karnofsky performance status; WBRT, whole brain radiotherapy; RPA, recursive partitioning analysis; GPA, graded prognostic assessment
Table 6
Prognostic variables predicting overall survival
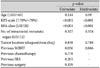
Table 7
Toxicity
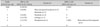
References
1. Cairncross JG, Kim JH, Posner JB. Radiation therapy for brain metastases. Ann Neurol. 1980; 7:529–541.
2. Aoyama H, Shirato H, Tago M, et al. Stereotactic radiosurgery plus whole-brain radiation therapy vs stereotactic radiosurgery alone for treatment of brain metastases: a randomized controlled trial. JAMA. 2006; 295:2483–2491.


3. Manning MA, Cardinale RM, Benedict SH, et al. Hypofractionated stereotactic radiotherapy as an alternative to radiosurgery for the treatment of patients with brain metastases. Int J Radiat Oncol Biol Phys. 2000; 47:603–608.


4. Andrews DW, Scott CB, Sperduto PW, et al. Whole brain radiation therapy with or without stereotactic radiosurgery boost for patients with one to three brain metastases: phase III results of the RTOG 9508 randomised trial. Lancet. 2004; 363:1665–1672.


5. Wowra B, Siebels M, Muacevic A, Kreth FW, Mack A, Hofstetter A. Repeated gamma knife surgery for multiple brain metastases from renal cell carcinoma. J Neurosurg. 2002; 97:785–793.


6. Shimamoto S, Inoue T, Shiomi H, et al. CyberKnife stereotactic irradiation for metastatic brain tumors. Radiat Med. 2002; 20:299–304.
7. Gaspar L, Scott C, Rotman M, et al. Recursive partitioning analysis (RPA) of prognostic factors in three Radiation Therapy Oncology Group (RTOG) brain metastases trials. Int J Radiat Oncol Biol Phys. 1997; 37:745–751.


8. Mintz A, Perry J, Spithoff K, Chambers A, Laperriere N. Management of single brain metastasis: a practice guideline. Curr Oncol. 2007; 14:131–143.


9. Muacevic A, Kreth FW, Horstmann GA, et al. Surgery and radiotherapy compared with gamma knife radiosurgery in the treatment of solitary cerebral metastases of small diameter. J Neurosurg. 1999; 91:35–43.


10. Muacevic A, Wowra B, Siefert A, Tonn JC, Steiger HJ, Kreth FW. Microsurgery plus whole brain irradiation versus Gamma Knife surgery alone for treatment of single metastases to the brain: a randomized controlled multicentre phase III trial. J Neurooncol. 2008; 87:299–307.


11. Vecht CJ, Haaxma-Reiche H, Noordijk EM, et al. Treatment of single brain metastasis: radiotherapy alone or combined with neurosurgery? Ann Neurol. 1993; 33:583–590.


12. Nishizaki T, Saito K, Jimi Y, et al. The role of cyberknife radiosurgery/radiotherapy for brain metastases of multiple or large-size tumors. Minim Invasive Neurosurg. 2006; 49:203–209.


13. Chang EL, Wefel JS, Maor MH, et al. A pilot study of neurocognitive function in patients with one to three new brain metastases initially treated with stereotactic radiosurgery alone. Neurosurgery. 2007; 60:277–283. discussion 283-4


14. Kased N, Binder DK, McDermott MW, et al. Gamma Knife radiosurgery for brain metastases from primary breast cancer. Int J Radiat Oncol Biol Phys. 2009; 75:1132–1140.


15. Coughlin C, Scott C, Langer C, Coia L, Curran W, Rubin P. Phase II, two-arm RTOG trial (94-11) of bischloroethyl-nitrosourea plus accelerated hyperfractionated radiotherapy (64.0 or 70.4 Gy) based on tumor volume (> 20 or < or = 20 cm(2), respectively) in the treatment of newly-diagnosed radiosurgery-ineligible glioblastoma multiforme patients. Int J Radiat Oncol Biol Phys. 2000; 48:1351–1358.


16. Sturm V, Kober B, Höver KH, et al. Stereotactic percutaneous single dose irradiation of brain metastases with a linear accelerator. Int J Radiat Oncol Biol Phys. 1987; 13:279–282.


17. Muacevic A, Kreth FW, Mack A, Tonn JC, Wowra B. Stereotactic radiosurgery without radiation therapy providing high local tumor control of multiple brain metastases from renal cell carcinoma. Minim Invasive Neurosurg. 2004; 47:203–208.


18. Wang ZZ, Yuan ZY, Zhang WC, You JQ, Wang P. Brain metastasis treated with Cyberknife. Chin Med J (Engl). 2009; 122:1847–1850.
19. Ernst-Stecken A, Ganslandt O, Lambrecht U, Sauer R, Grabenbauer G. Phase II trial of hypofractionated stereotactic radiotherapy for brain metastases: results and toxicity. Radiother Oncol. 2006; 81:18–24.


20. Aoyama H, Shirato H, Onimaru R, et al. Hypofractionated stereotactic radiotherapy alone without whole-brain irradiation for patients with solitary and oligo brain metastasis using noninvasive fixation of the skull. Int J Radiat Oncol Biol Phys. 2003; 56:793–800.


21. Stafinski T, Jhangri GS, Yan E, Menon D. Effectiveness of stereotactic radiosurgery alone or in combination with whole brain radiotherapy compared to conventional surgery and/or whole brain radiotherapy for the treatment of one or more brain metastases: a systematic review and meta-analysis. Cancer Treat Rev. 2006; 32:203–213.

