Abstract
Purpose
Materials and Methods
Results
Figures and Tables
Fig. 1
Src homology 2 domain–containing adaptor protein B (SHB) expression in bone marrow (BM)–derived dendritic cell (BMDC) development. BM cells from C57BL/6 mice were cultured for 6 days in the presence of granulocyte-macrophage colony-stimulating factor (10 ng/mL) to generate BMDCs. (A) Total RNA was isolated from immature dendritic cells (imDCs) and lipopolysaccharide (LPS)-treated mature dendritic cells (mDCs), and SHB mRNA was assessed from each sample by reverse transcriptase polymerase chain reaction (RT-PCR) and quantitative real-time polymerase chain reaction (qRT-PCR) with the Maxime RT-PCR PreMix (iNtRON) and Fast SYBR Green Master Mix (Life Technologies) kits, respectively. (B) The amount of SHB protein expressed in imDCs and mDCs was assessed by Western blot (WB) assay. (C) SHB expression in imDCs and mDCs was assessed by fluorescence-activated cell sorting after intracellular staining. (D, E) Splenic DCs (spDCs) were isolated from mice using a CD11c+ isolation kit (Miltenyi Biotech) and treated or not with LPS (200 ng/mL) for 24 hours. Intracellular SHB protein expression in spDCs was assessed by fluorescence-activated cell sorter (D) and WB assay (E). RT-PCR data are shown as the mean±standard deviation of nine samples pooled from three independent experiments.
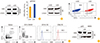
Fig. 2
Src homology 2 domain–containing adaptor protein B (SHB) expression requires mitogen-activated protein kinase signaling and Foxa2 activation during dendritic cell development. Mouse bone marrow (BM)–derived dendritic cells (BMDCs) were treated or not with p38-inhibitor SB2013580 (200 µM) for 2 hours prior to cell harvest. (A) Cell lysates were subjected to sodium dodecyl sulfate polyacrylamide gel electrophoresis separation and then assessed by Western blot assay for p38, p-p38, Foxa2, and SHB. (B) Foxa2 and p-Foxa2 were assessed by immunoprecipitation (IP) with anti-Foxa2 antibody and protein A/G agarose. (C) BM cells were transfected with si-con and si-Foxa2 on day 4 during BMDC development and then harvested 48 hours after transfection. Cell lysates were analyzed by Western blot assay for Foxa2 and SHB.
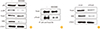
Fig. 3
Effects of Src homology 2 domain–containing adaptor protein B (SHB)–knockdown (SHBKD) on bone marrow–derived dendritic cells (BMDC) phenotypes. Dendritic cell (DC) precursor cells were transfected with SHB-specific siRNA (si-shb) or control siRNA (si-con) on day 4 during BMDC development. Cells were harvested 48 hours after transfection and were used as a source of wild type (WT) and SHBKD DCs. (A) Silencing was assessed by Western blot. (B) Representative fluorescence-activated cell sorting data of surface phenotypes showing SHBKD DCs (si-shb) or WT DCs (si-con) in their immature form. (C) Statistical data showing mean fluorescence intensity (MFI) of DC surface phenotypes in B are presented as the mean ± standard deviation from three individual experiments. Student's t test, **p < 0.01, ***p < 0.001 in comparison with WT DCs.
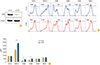
Fig. 4
The effects of Src homology 2 domain–containing adaptor protein B (SHB)–silencing on the cytokine profiles of bone marrow (BM)–derived dendritic cells. Pro- and anti-inflammatory Th1-type cytokines were assessed by enzyme-linked immunosorbent assay from the culture supernatants of SHB-knockdown (SHBKD) and wild type mDCs, which were generated from the BM cells of C57BL/6 mice and matured with lipopolysaccharide (200 ng/mL) for 24 hours. Data are shown as the mean±standard deviation of nine samples pooled from three independent experiments. IL, interleukin; TNF-α, tumor necrosis factor α.
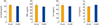
Fig. 5
The effects of Src homology 2 domain–containing adaptor protein B (SHB)–silencing on the T-cell stimulation capacity of dendritic cells (DCs). OT-2 T cells were co-cultured with wild type (WT) (si-con) and SHB-knockdown (SHBKD) OVA323-339 peptide-pulsed DCs. (A) A bright field image of representative T-cell colonies (OT-2) on day 3 of DC/T cell co-culture (upper). T-cell proliferation was assessed by MTT assay (lower). (B) OVA323-339 peptide-pulsed or unpulsed (up) WT and SHBKD mDCs were co-cultured with 5,6-carboxyflouroscein succinimidyl ester (CFSE)–labeled OT-2 T cells for 72 hours at a 1:5 ratio of DC:T cells. CFSE-labeled T-cell proliferation was assessed by fluorescence-activated cell sorting (left) and represented by fold increase at three different ratios using T cell alone as a control (right). (C) Th1 (interferon γ [IFN-γ]), Th17 (interleukin [IL]-17), and Th2 (IL-4, IL-13) cytokines were assessed by enzyme-linked immunosorbent assay from the culture supernatants of DC:T cell co-cultures at a 1:5 ratio on day 3. (D) Treg cell populations were assessed by intracellular Foxp3 staining and CD25 surface staining from the co-cultures of OVA323-339 peptide-pulsed mDCs (C57BL/6) and OT-2 T cells at a ratio 1:5 for 4 days. Data in C and D are shown as the mean ± SD of six samples pooled from three independent experiments. Student's t test, *p<0.05, **p<0.01.
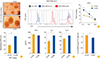
Fig. 6
Src homology 2 domain–containing adaptor protein B–knockdown (SHBKD) dendritic cells (DCs) induce severe symptoms of atopic dermatitis in mice. Induction of atopic dermatitis was performed in BALB/c mice (n = 4) by applying dinitrocholorobenzene (Sigma) to the outer and inner surfaces of ears as described in the “Materials and Methods.” The mice were then subcutaneously injected twice on days 3 and 10 with 1×106 OVA323-339 peptide-pulsed SHBKD or wild type (WT) mDCs. (A) Ears of mice with atopic dermatitis are shown after DC inoculation. (B) Ear thickness of mice with atopic dermatitis was monitored every 3 days and represented as the mean±standard deviation of 4 mice from each experiment. Student's t test. *p < 0.05, **p < 0.005. (C) Histological analysis of atopic dermatitis after inoculation with WT or SHBKD DCs. The ears with dermatitis were obtained on day 33, fixed, dehydrated, and embedded in paraffin blocks. The histopathology of each paraffin section was examined after (× 400). Infiltrating immune cells are indicated by arrows.
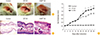
Notes
This work was supported by the Bio & Medical Technology Development Program of the National Research Foundation (NRF) funded by the Korean Ministry of Education Science and Technology (2012M3A9B4028271), and in part by the Infectious Disease Risk Management and Technology Development grants (HI16C1074) from the Korean Ministry of Health and Welfare.
References
























