Abstract
Despite the tremendous efforts to develop a successful human immunodeficiency virus (HIV) vaccine, the quest for a safe and effective HIV vaccine seems to be remarkably long and winding. Disappointing results from previous clinical trials of VaxGen's AIDSVAXgp120 vaccine and MRKAd5 HIV-1 Gag/Pol/Nef vaccine emphasize that understanding the correlates of immune protection in HIV infection is the key to solve the puzzle. The modest vaccine efficacy from RV144 trial and the successive results obtained from the correlate of risk analysis have reinvigorated the HIV vaccine research field leading to various novel strategies. This paper will review the brief history and recent advances in HIV vaccine development.
Despite the continuous search for an effective vaccine against human immunodeficiency virus (HIV), our effort to develop the vaccine that could end the global HIV-1 pandemic has not been rewarded. Various prevention strategies, such as provision and scaling up highly active antiretroviral therapy, large-scale circumcision programs, pre- and post-exposure prophylaxis for high-risk individuals, topical microbicides, and introduction of antiretroviral therapy to prevent mother to child transmission have contributed to substantial reduction in global HIV incidence and mortality [123456]. However, the benefits of nonvaccine prevention strategies are diminished by factors such as inadequate financial and human resources and limited compliance and poor adherence [7]. Conflicting results from recent preexposure prophylaxis (PrEP) trials highlight the issues of which strategy to take in PrEP (focused vs. general) considering the epidemiologic impact and cost-effectiveness of combining prevention methods in resource-limiting settings. For this reason, HIV vaccine remains to be essential in combating current HIV pandemics. In this paper, brief history and the recent advances in HIV vaccine development will be reviewed.
In 1983, when the HIV was isolated and confirmed as the cause of acquired immunodeficiency syndrome (AIDS), the optimism toward HIV vaccine development was prevailing. The danger of integration of the proviral DNA in the host chromosome prohibited traditional approaches to use live attenuated or whole inactivated viruses in HIV vaccine development. With the new recombinant DNA technologies and rapid advances in the molecular biology of HIV, two gp120 products emerged as potential candidates for an efficacy trial. Despite the debates between scientists supporting empirical approach and those supporting theoretical approach, rapidly growing HIV pandemic put immense pressure to expedite vaccine development. The phase III VaxGen's AIDSVAXgp120 trial moved forward with industry support. Unfortunately, two phase III gp120 vaccine trials in men who have sex with men in the North America (VAX004) and injection drug users in Thailand (VAX003) failed to demonstrate protection [89]. Disappointing results of VaxGen trial made HIV researchers to turn from B-cell targeted vaccines designed to induce neutralizing antibodies to T-cell targeted approach. The cytotoxic T lymphocyte (CTL) vaccine approach is to develop a vaccine designed to lower viral set point and delay disease progression, rather than to prevent initial infection [10]. Pox viruses and adenoviruses have been preferentially used for the viral vectors. With piling evidences highlighting the importance of CTL response in the virus control from preceding studies, Phase IIb, proof of concept, MRKAd5 HIV-1 Gag/Pol/Nef vaccine was further proceeded. Among the two viral vectors, adenovirus type 5 vectored (Ad5) candidates were moved on to the clinical trials due to their favorable immunogenicity in animal studies and early-stage human trials. Much to our dismay, two phase IIb trials (STEP and Phambili) were halted because the vaccine revealed not only poor efficacy but it also demonstrated evidence of increased risk of viral acquisition among vaccine recipient as compared with placebo [1112]. It was later suggested that vaccine-related immune activation might have led to increased susceptibility to infection [13]. With disappointing results from the vaccines based on the virus envelope protein gp120 and CTL directed vaccines, pessimism and frustration grew among HIV vaccine researchers. The future prospect for HIV vaccinology seemed grim.
The approach of combining the AIDSVAX B/E gp120 vaccine with the CD4+ T-cell stimulating ALVAC canarypox vaccine (ALVAC-HIV/AIDSVAX B/E) was tested in the face of considerable criticism. Not only AIDSVAX B/E protein vaccine had proven ineffective when used alone in preventing HIV-1, ALVAC was less immunogenic than Ad5 vectors in preclinical testing [2814]. However, the RV144 trial turned out to be the first trial of a vaccine against HIV-1 to show any degree of efficiency. RV144 was a randomized, multicenter, double blind, placebo controlled efficacy trial of recombinant canarypox vector vaccine done among 16,402 health participants in Thailand. The first year vaccine efficacy approached 60%; however, the efficacy waned over time to 31.2% (95% confidence interval, 1.1 to 52.1; p=0.04) over 42 months suggesting early but nondurable vaccine effect [15]. This modest but unexpected result obtained from RV144 trial revived the depressed HIV vaccine research field. With the extensive search for the correlate of protection among vaccinees, two strong correlates with vaccine efficacy were found. Plasma concentration of IgG antibody specific for V1V2loop region of envelope gp120 was inversely correlated with risk for HIV acquisition while IgA antibody to HIV-1 Env was directly correlated with acquisition of infection [16]. This result highlighted the importance of antibody mediated protection and turned the HIV vaccine paradigm back to the induction of antibodies. Further analysis showed that in vaccinees with low levels of Env-specific IgA antibodies, IgG avidity, antibody-dependent cell-medicate cytotoxicity, non-neutralizing antibodies (nNAbs) and Env-specific CD4+ T cell, were inversely correlated with risk of infection [161718]. A correlate of risk analysis of RV144 provided the novel direction for the future research.
One of the important finding from RV144 trial is that it suggested that nNAb can be functional and indeed neutralize HIV-1 in vivo. The vaccine efficacy from the ALVAC-HIV/AIDSVAX B/E vaccine in RV144 trial is not due to broadly neutralizing antibodies (bNAbs) nor does it induce measurable CTL responses [1519]. Monomeric gp120 HIV-1 envelop proteins alone fail to show protection from HIV acquisition in two previous efficacy trials, Vax003 and Vax004. The RV144 correlates of risk analysis suggested that plasma IgG binding antibody to scaffolded gp70 V1V2 case A2 envelope protein correlated inversely with risk. The differences between the immune responses in RV144 (with observed vaccine efficacy) and those seen in HVTN505 and VAX003 (without vaccine efficacy) provide indirect evidence of the potential importance of the type of nNAbs in reducing HIV-1 acqusition [2]. Further studies suggest that V2 IgG plays a role in protection against HIV-1 acquisition but the mechanism is yet to be cleared [1520]. Recently published nonhuman primate studies also indicate that nNAbs are associated with protection form experimental challenges with both simian immunodeficiency virus (SIV) and SIV/HIV hybrid virus. In a SIVmac251 low dose intrarectal challenge study of rhesus macaques, protection by an Ad26-MVA Gag-Pol-Env vaccine combination was associated with requirement for Env immunogen, anti-Env biding antibody, and V2-specific antibody response [21]. The HIV vaccine efficacy trials are summarized in Table 1.
Series of discoveries related to bNAbs have been made re cently. Investigators found that roughly 20% of infected individuals developed bNAbs, antibodies capable of neutralizing a wide range of viral isolates, after 2-3 years of infection. However, unlike classical viral infection, these bNAbs do not control the viral replication due to continuously emerging escape viruses [2223]. Most bNAbs fall into following groups on the bases of the location on the viral spike of the conserved epitopes that they recognize: CD4-binding site, V1/V2 variable loops, certain exposed glycans, and membrane proximal external region (Fig. 1) [24].
Their efficacy in preventing infection is yet to be proven in humans; however, nonhuman primate studies with passive transfer of bNAbs have promising results. With passive transfer of bNAbs, the studies demonstrated that prevention of viral acquisition among uninfected animals [25] and suppression of viremia among infected animals [2627]. Ex vivo studies have shown marked suppression of expression of virus from HIV viral reservoirs by bNAbs [28]. Human in vivo studies have shown decreased levels of circulating virus after administration of bNAbs [29]. However, development of bNAbs typically occurred only after many years of HIV infection due to evolutionary hurdles that bNAbs must overcome. Some are autoreactive and clonally deleted to prevent the development of autoimmunity; some have specialized structures, such as long complementary-determining regions that are rare among germline B cells; and others require a high degree of somatic hypermutation [30].
With the promising results of passive transfer studies, induction of bNAbs by active immunization is pursued for the future vaccine strategies. The challenge is to develop a pure stable envelop immunogen that mimics the antigenic profile of the functional envelope spike.
HIV Env neutralization is hampered by (1) its remarkable antigenic diversity, (2) concealing critical epitope via its quaternary structure and heavy glycosylation, (3) antibodies must go extensive somatic hypermutation to gain the ability to recognize the native trimer and block HIV infection [3132]. To solve the problem, the envelop trimer itself is now stabilized in a soluble form and used as a immunogen [33343536]. Recent animal study using envelop timer, BG505 SOSIP, to immunize rabbits and monkeys were published suggesting the possibility of using envelop timer for the further development of HIV vaccine.
On the other hand, other researchers pursue the B-cell lineage vaccine design strategies which aim at engaging the naïve B-cell repertoire. This approach is to identify the drivers that are responsible for the sequential stimulation of HIV-1 reactive B-cell lineage to yield the bNAbs and use the information to make templates for designing immunogens. Those constructed immunogens are further used in prime boost to engage the naïve B cell in vivo and stimulate B-cell evolution until bNAbs producing cells are elicited.
With piling evidences suggesting CTL responses targeting Gag have been associated with relative control of viral replication and elite control of viremia in vivo, the failure of MRK rAd5 vaccine trial, known as STEP trial, was a genuine set back. Sieve analysis of breakthrough infection in the STEP trial demonstrated that vaccine induced immune pressure was detected by the identification of virus-escape mutants to vaccine induced T-cell responses [37]. This and proceeding positive results from nonhuman primates studies have suggested that the CTL vaccine might be still worth investigating [213839].
One of the major obstacles for an effective CTL vaccine is the virus variability. The CTL escape mutant emerges as to evade T-cell responses, and eventually emasculate the vaccine. It is evident that viral escape is influenced by the strength of T-cell responses as well as its ability to escape with a minimal fitness cost [40]. To overcome the virus variability, several research groups adapted the conserved-epitope strategies to target the most conserved regions of HIV which is usually a critical viral elements and mutations in which typically cause a replicative fitness loss. Another feasible approach is computing multivalent mosaic proteins which maximize the coverage of potential 9-mer T-cell epitopes of the input viral sequences. By broadening the T-cell responses to stimulate responses to those normally subdominant epitopes as well as the commoner variable epitopes, this mosaic immunogen strategies may contribute to protect against infection by genetically diverse viruses and to control the possible CTL escapes mutants [41]. Another promising method using replicating vectors are also being pursued. A replication-competent rhesus cytomegalovirus vaccine expressing SIV proteins induced and maintained high frequency of SIV specific CD4+ and CD8+ T-cell responses without measurable antibody responses to SIV. This strategy does not prevent initial infection but control and eliminate virus in 50% of animals via a potent stimulation of SIV-specific CTL reponses [3942].
To date, RV144 trial is the only trial of a vaccine against HIV-1 to show any degree of efficiency. The modest vaccine efficacy from RV144 trial and the successive results obtained from the correlate of risk analysis have reinvigorated the HIV vaccine research field leading to various novel strategies. Although the quest to develop a successful HIV vaccine is long and winding, our understanding of viral immunology and immune correlate of protection has progressed remarkably during the journey. We now are starting to have a glimpse of correlate of protection in HIV infection, better understanding of the immune pathway leading to effective antibodies and newer form of vaccine immunogens. The ultimate goal of the quest to develop the successful HIV vaccine is not yet accomplished but it is evident that we are entering the modern era of HIV vaccinology.
Figures and Tables
Fig. 1
Schematic drawing of human immunodeficiency virus type 1 Env trimer with sites of epitopes for broadly neutralizing antibodies. Adapted and modified from Haynes et al. (2012), Nat Biotechnol 2012;30:423-33 [30]. The currently known four general specificity for broadly neutralizing antibodies detected are the CD4 binding site, the V1/V2 variable loops, certain exposed glycans and the membrane proximal external region (MPER). Structures expressed as follows: blue, gp120 core; dark blue, V1/V2 loops; magenta, V3 loop; green, gp41; red, MPER of gp41; light gray, viral membrane bilayer.
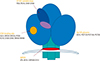
Table 1
The HIV vaccine efficacy trial
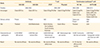
References
1. Tanser F, Barnighausen T, Grapsa E, Zaidi J, Newell ML. High coverage of ART associated with decline in risk of HIV acquisition in rural KwaZulu-Natal, South Africa. Science. 2013; 339:966–971.


2. Corey L, Gilbert PB, Tomaras GD, Haynes BF, Pantaleo G, Fauci AS. Immune correlates of vaccine protection against HIV-1 acquisition. Sci Transl Med. 2015; 7:310rv7.


3. Gray R, Kigozi G, Kong X, et al. The effectiveness of male circumcision for HIV prevention and effects on risk behaviors in a posttrial follow-up study. AIDS. 2012; 26:609–615.


4. Haberer JE, Baeten JM, Campbell J, et al. Adherence to antiretroviral prophylaxis for HIV prevention: a substudy cohort within a clinical trial of serodiscordant couples in East Africa. PLoS Med. 2013; 10:e1001511.


5. Cohen MS, Chen YQ, McCauley M, et al. Prevention of HIV-1 infection with early antiretroviral therapy. N Engl J Med. 2011; 365:493–505.


6. Abdool Karim Q, Abdool Karim SS, Frohlich JA, et al. Effectiveness and safety of tenofovir gel, an antiretroviral microbicide, for the prevention of HIV infection in women. Science. 2010; 329:1168–1174.


7. Fauci AS, Folkers GK, Marston HD. Ending the global HIV/AIDS pandemic: the critical role of an HIV vaccine. Clin Infect Dis. 2014; 59:Suppl 2. S80–S84.


8. Pitisuttithum P, Gilbert P, Gurwith M, et al. Randomized, double-blind, placebo-controlled efficacy trial of a bivalent recombinant glycoprotein 120 HIV-1 vaccine among injection drug users in Bangkok, Thailand. J Infect Dis. 2006; 194:1661–1671.


9. Flynn NM, Forthal DN, Harro CD, et al. Placebo-controlled phase 3 trial of a recombinant glycoprotein 120 vaccine to prevent HIV-1 infection. J Infect Dis. 2005; 191:654–665.


10. Fauci AS, Marston HD. Public health: toward an HIV vaccine: a scientific journey. Science. 2015; 349:386–387.
11. Buchbinder SP, Mehrotra DV, Duerr A, et al. Efficacy assessment of a cell-mediated immunity HIV-1 vaccine (the Step Study): a double-blind, randomised, placebo-controlled, test-of-concept trial. Lancet. 2008; 372:1881–1893.


12. Duerr A, Huang Y, Buchbinder S, et al. Extended follow-up confirms early vaccine-enhanced risk of HIV acquisition and demonstrates waning effect over time among participants in a randomized trial of recombinant adenovirus HIV vaccine (Step Study). J Infect Dis. 2012; 206:258–266.


13. Fauci AS, Marovich MA, Dieffenbach CW, Hunter E, Buchbinder SP. Immunology: immune activation with HIV vaccines. Science. 2014; 344:49–51.
14. Nitayaphan S, Pitisuttithum P, Karnasuta C, et al. Safety and immunogenicity of an HIV subtype B and E prime-boost vaccine combination in HIV-negative Thai adults. J Infect Dis. 2004; 190:702–706.


15. Rerks-Ngarm S, Pitisuttithum P, Nitayaphan S, et al. Vaccination with ALVAC and AIDSVAX to prevent HIV-1 infection in Thailand. N Engl J Med. 2009; 361:2209–2220.


16. Haynes BF, Gilbert PB, McElrath MJ, et al. Immune-correlates analysis of an HIV-1 vaccine efficacy trial. N Engl J Med. 2012; 366:1275–1286.


17. Zolla-Pazner S, deCamp AC, Cardozo T, et al. Analysis of V2 antibody responses induced in vaccinees in the ALVAC/AIDSVAX HIV-1 vaccine efficacy trial. PLoS One. 2013; 8:e53629.


18. Karasavvas N, Billings E, Rao M, et al. The Thai Phase III HIV Type 1 Vaccine trial (RV144) regimen induces antibodies that target conserved regions within the V2 loop of gp120. AIDS Res Hum Retroviruses. 2012; 28:1444–1457.


19. Montefiori DC, Karnasuta C, Huang Y, et al. Magnitude and breadth of the neutralizing antibody response in the RV144 and Vax003 HIV-1 vaccine efficacy trials. J Infect Dis. 2012; 206:431–441.


20. Excler JL, Robb ML, Kim JH. Prospects for a globally effective HIV-1 vaccine. Vaccine. 2015; 33:Suppl 4. D4–D12.


21. Barouch DH, Liu J, Li H, et al. Vaccine protection against acquisition of neutralization-resistant SIV challenges in rhesus monkeys. Nature. 2012; 482:89–93.
22. Sather DN, Armann J, Ching LK, et al. Factors associated with the development of cross-reactive neutralizing antibodies during human immunodeficiency virus type 1 infection. J Virol. 2009; 83:757–769.


23. Gray ES, Madiga MC, Hermanus T, et al. The neutralization breadth of HIV-1 develops incrementally over four years and is associated with CD4+ T cell decline and high viral load during acute infection. J Virol. 2011; 85:4828–4840.


24. Kwong PD, Mascola JR. Human antibodies that neutralize HIV-1: identification, structures, and B cell ontogenies. Immunity. 2012; 37:412–425.


25. Shingai M, Donau OK, Plishka RJ, et al. Passive transfer of modest titers of potent and broadly neutralizing anti-HIV monoclonal antibodies block SHIV infection in macaques. J Exp Med. 2014; 211:2061–2074.


26. Barouch DH, Whitney JB, Moldt B, et al. Therapeutic efficacy of potent neutralizing HIV-1-specific monoclonal antibodies in SHIV-infected rhesus monkeys. Nature. 2013; 503:224–228.


27. Shingai M, Nishimura Y, Klein F, et al. Antibody-mediated immunotherapy of macaques chronically infected with SHIV suppresses viraemia. Nature. 2013; 503:277–280.


28. Chun TW, Murray D, Justement JS, et al. Broadly neutralizing antibodies suppress HIV in the persistent viral reservoir. Proc Natl Acad Sci U S A. 2014; 111:13151–13156.


29. Caskey M, Klein F, Lorenzi JC, et al. Viraemia suppressed in HIV-1-infected humans by broadly neutralizing antibody 3BNC117. Nature. 2015; 522:487–491.
30. Haynes BF, Kelsoe G, Harrison SC, Kepler TB. B-cell-lineage immunogen design in vaccine development with HIV-1 as a case study. Nat Biotechnol. 2012; 30:423–433.


31. Klein F, Mouquet H, Dosenovic P, Scheid JF, Scharf L, Nussenzweig MC. Antibodies in HIV-1 vaccine development and therapy. Science. 2013; 341:1199–1204.


32. Mascola JR, Haynes BF. HIV-1 neutralizing antibodies: understanding nature's pathways. Immunol Rev. 2013; 254:225–244.


33. Sanders RW, van Gils MJ, Derking R, et al. HIV-1 VACCINES. HIV-1 neutralizing antibodies induced by native-like envelope trimers. Science. 2015; 349:aac4223.
34. Chen J, Kovacs JM, Peng H, et al. HIV-1 ENVELOPE. Effect of the cytoplasmic domain on antigenic characteristics of HIV-1 envelope glycoprotein. Science. 2015; 349:191–195.


35. Jardine JG, Ota T, Sok D, et al. HIV-1 vaccines: priming a broadly neutralizing antibody response to HIV-1 using a germline-targeting immunogen. Science. 2015; 349:156–161.


36. Dosenovic P, von Boehmer L, Escolano A, et al. Immunization for HIV-1 broadly neutralizing antibodies in human Ig knockin mice. Cell. 2015; 161:1505–1515.


37. Rolland M, Tovanabutra S, deCamp AC, et al. Genetic impact of vaccination on breakthrough HIV-1 sequences from the STEP trial. Nat Med. 2011; 17:366–371.


38. Liu J, O'Brien KL, Lynch DM, et al. Immune control of an SIV challenge by a T-cell-based vaccine in rhesus monkeys. Nature. 2009; 457:87–91.


39. Hansen SG, Piatak M Jr, Ventura AB, et al. Immune clearance of highly pathogenic SIV infection. Nature. 2013; 502:100–104.


40. Ferrari G, Korber B, Goonetilleke N, et al. Relationship between functional profile of HIV-1 specific CD8 T cells and epitope variability with the selection of escape mutants in acute HIV-1 infection. PLoS Pathog. 2011; 7:e1001273.

