Abstract
Chronic diseases such as obesity and diabetes are major causes of death and disability throughout the world. Many causes are known to trigger these chronic diseases, and infectious agents such as viruses are also pathological factors. In particular, it is considered that adenovirus 36 infections may be associated with obesity. If this is the case, a vaccine against adenovirus 36 may be a form of prophylaxis to combat obesity. Other types of therapeutic vaccines to combat obesity are also being developed. Recently, hormones such as glucagon-like peptide-1, ghrelin, and peptide YY have been studied as treatments to prevent obesity. This review describes the ongoing development of therapeutic vaccines to treat obesity, and the possibility of using inactivated adenovirus 36 as a vaccine and an anti-obesity agent.
Vaccines have been developed for many infectious diseases since the early twentieth century and they have provided numerous benefits to human society. One of these benefits is the increased lifespan caused by the prevention of infectious diseases. However, the types of diseases have changed alongside the increased lifespan caused by the prevention of infectious disease. These new health threats are chronic diseases such as cancer, cardiovascular diseases, diabetes, and obesity [1]. Many therapeutic vaccine approaches have been developed for preventing chronic diseases [2]. In this review, we discuss the prophylaxes and therapeutic vaccinations that could be used to reduce the incidence of obesity and diabetes. In particular, we describe the possibility of treating pathogen-induced obesity.
Obesity is classified as one of the eight significant causes of chronic disease by the World Health Organization (WHO). Sixty-five percent of the world's population lives in countries where overweight and obesity kills more people than underweight [3]. The number of health problems linked to chronic diseases is increasing dramatically and obesity is related to numerous chronic diseases. Many studies have also shown that obesity has a specific relationship with type 2 diabetes [4-6].
Obesity causes many problems in humans, but the treatment options for obesity are very limited. It is widely accepted that a combination of dieting and physical activity is the most effective way of reducing obesity [7]. However, these methods are highly dependent on the motivation of individual subjects. Thus, healthy practices are readily stopped and subjects may return to their previous weight, which is called the yo-yo effect. Therefore, several medications are being developed for treating obesity. However, current medications only have a beneficial effect if the treatment is sustained continuously [8]. In addition, bariatric surgery may lead to permanent weight loss but this method has significant perioperative risks and an economic burden for the patient [9,10]. To address the problems of obesity treatment, recent studies have shown that therapeutic vaccines may be new targets for the development of anti-obesity medications. The therapeutic vaccines could treat alternatively the obesity by suppressing the appetite-stimulating hormone and blocking absorption of nutrients.
One therapeutic strategy is to block endogenous signals to reduce the appetite and body weight. In particular, immunization against the gut peptide, ghrelin, has entered phase I and IIa trials as an anti-obesity therapy [11]. Ghrelin is produced mainly in the small and large intestines, although it is also secreted by the lungs, pancreatic islets, gonads, kidney, and brain [12]. Ghrelin is an appetite-regulating hormone and a contributor to mealtime hunger [13]. The levels of ghrelin increase before meals and decrease after feeding [13]. Ghrelin has a wide range of biological functions. In particular, it promotes weight gain by increasing the appetite and food intake, while reducing the energy expenditure [14,15]. However this vaccine could cause emotional side effects. To identify the possibility of its use as a vaccine, synthetic ghrelin analogs were conjugated to haptens [16]. Mice immunized with the ghrelin peptide sequence gained less body weight and had lower body fat and circulating leptin levels. In addition, a therapeutic vaccine has been tested that used a noninfectious virus to carry ghrelin to elicit an immune response to obstruct this hormone. This vaccine induced antibodies against ghrelin, showing that it can suppress this hormone. Vaccinated mice had higher levels of anti-ghrelin antibodies compared with unvaccinated mice. Moreover, the vaccinated mice had a lower food intake and increased energy expenditure, i.e., obese mice had 82% of the food intake consumed by the control mice after the first vaccination injection and only 50% of the food intake of the control mice after the final vaccination [16]. In addition, the vaccinated obese mice exhibited reduced neuropeptide Y (NPY) expression. NPY is the most potent signal that increases the appetite via the central nervous system [17,18].
Furthermore, a 'flab jab' vaccine has been reported that reduced the body weight by 10% four days after a single injection [19]. The vaccine was designed to block the effects of somatostatin, which has many different functions in various organs. In particular, somatostatin can suppress the release of pancreatic hormones. The release of somatostatin from the pancreas prevents insulin secretion and reduces the release of glucagon. Blocking this growth hormone has positive effects on obesity in obese animal models and in human studies. For example, vaccinated mice produced antibodies against somatostatin and their food intake was reduced compared with control mice at six weeks after vaccination. The vaccinated mice lost 12-13% of their body weight at four days after the first injection, although all of the mice consumed similar amounts of food [19]. Mice treated with the two vaccines, JH17 and JH18, exhibited 4% and 7% increases in their body weight, respectively, compared with their body weight at the start of the study. By contrast, control mice exhibited a 15% increase in their body weight. However, this study had several limitations, such as problems with the control vaccination, the overall weight gain, and the unrealistic volume of vaccine required [19]. In addition, this vaccine could cause steatorrhea, diarrhea, gastrointestinal cramps, and occasional nausea. Therefore, somatostatin vaccines can reduce weight gain and the final body weight compared with the baseline weight, although there are some problems with this approach.
Active vaccination against target molecules is another therapeutic vaccine approach. The recombinant virus-like particle (VLP) has a similar structure to viruses but lacks sufficient genetic information to replicate [20,21]. Thus, this type of vaccine is less dangerous compared with live virus vaccines. Moreover, VLPs derived from the coat protein of bacteriophage Qβ have no preexisting immunity [22]. During VLP assembly, single-stranded RNA triggers Toll-like receptors 7 and 8 [23], and these receptors induce co-stimulatory molecules and cytokines that promote antigen-specific IgG2a responses [24,25]. Chemical conjugation can be used to attach antigens to the surface of Qβ-VLP, thereby triggering potent antibody responses in animals and humans. In particular, VLP-based vaccines can induce antibodies against interleukin (IL)-1β to treat type 2 diabetes [26]. The cytokine IL-1β triggers islet cell apoptosis and is a primary agonist in the loss of beta cell mass in type 2 diabetes. Therefore, the prevention of IL-1β may improve the symptoms of type 2 diabetes patients [27,28]. When mice were immunized with the full-length IL-1β protein coupled to Qβ-VLP, they produced high neutralizing antibody titers with specificity for IL-1β [29]. The antibody response against the mutant form of IL-1β conferred protection in a rheumatoid arthritis animal model and the response also ameliorated the diabetic phenotype in a diet-induced obesity model [30].
Obesity is a trigger of metabolic disease. Interestingly, the immune system is functionally linked to obesity. In particular, lymphoid tissues appear to be very sensitive to nutrient imbalances [31]. Therefore, these tissues have effects on metabolic pathways and the functions required for immune defense. Any impairment of the immune response may make obese individuals more susceptible to infection. In addition, it is well known that the effect to vaccination is reduced by obesity (Fig. 1) [32]. A previous study demonstrated an association between low antibody levels, obesity, and the response to hepatitis B vaccines [33]. Another study showed that a standard hepatitis B vaccine had a 71% protection rate in obese adults (body mass index >30) compared with 91% in the healthy control group [34]. A further study investigated the mechanism related to the reduced antibody response to vaccination [35], where ob/ob obese mice exhibited 21% and 12% lower levels of pre-B and immature B cells, respectively, compared with wild type C57BL/6 mice [35]. These results indicate that obesity can modify the immune system to produce an immunodeficient state, including altered lymphocyte and monocyte functions. Diet-induced and genetic obesity increase the susceptibility to bacterial and viral infections in humans and animals. Therefore, it is necessary to perform intensive studies to determine the effects of obesity on the efficacy of vaccinations.
Recently, many studies have reported that infections with pathogens can induce obesity and metabolic diseases. Thus, preventing and treating infection by selected pathogens may help to reduce the risk of the adverse effects of obesity.
Of the known obesity-inducing pathogens, human Ad36 induces adiposity but paradoxically it improves insulin sensitivity [36-38]. Ad36-infected animals have a higher body weight and fat content, but hypolipidemia compared with control animals. There is a strong association between Ad36 infection and human obesity [36-38]. Ad36 is a human adenovirus that belongs to serotype D and it is antigenically distinct [39]. According to epidemiological data, 30% of obese and 11% of non-obese adults in the United States possess antibodies against Ad36 [40]. Moreover, it has been reported that there is a relationship between viral infections with Ad36 and obesity in several nations [41-44]. However, research into the treatment of viral infections and vaccine development is still lacking in this area.
A previous study tested the possibility of using an inactivated Ad36 vaccine [45], where Ad36 was inactivated using ultraviolet (UV) irradiation. In trials, mice were inoculated with live Ad36, UV-inactivated Ad36, or the medium alone. Live Ad36 increased the size of the epididymal fat pad at 4 days post-inoculation, whereas UV-inactivated Ad36 did not cause an increase [45]. Furthermore, our group demonstrated a 'proof of concept' using an Ad36 vaccine (unpublished data). We injected mice with UV-inactivated Ad36, live Ad36 as the positive control, and medium as the negative control, before challenge with live Ad36 (unpublished data). The mice were sacrificed and their organs were used to study the chronic effects at 90 days post-challenge. Live Ad36-injected mice had larger epididymal fat pads and exhibited inflammation of their fat deposits. However, the body weight and epididymal fat levels of the UV-inactivated Ad36-injected mice were similar to those of the control mice. Moreover, treatment with UV-inactivated Ad36 decreased the level of inflammation in the fat deposits. Indeed, inactivated Ad36 reduced the levels of inflammatory cytokines and immune cells. Overall, these results show that UV-inactivated Ad36 can prevent increases in body fat and inflammation. Therefore, inactivated Ad36 may be a potential vaccine to prevent Ad36-induced obesity.
Hormones have been tested as targets to treat 'conventional' obesity by developing therapeutic vaccines. The incidence of pathogen-induced obesity is increasing, but the development of vaccines to treat these pathogens is insufficient at present. Several studies have demonstrated the 'proof of concept' of the potential application of a prophylactic vaccine against Ad36 as an anti-obesity agent. An inactivated Ad36 vaccine can prevent increases in the body weight and fat content, as well as reducing inflammation in fat deposits. However, this type of anti-obesity vaccine research is just beginning. Therefore, more intensive studies are required to develop prophylactic and therapeutic vaccines to prevent obesity.
Figures and Tables
Fig. 1
(A) Mortality after challenge with pandemic 2009 H1N1 virus in vaccinated mice fed with a normal chow diet or a high-fat diet. (B) A 2009 H1N1 virus-specific serum antibody titers were analyzed by enzyme-linked immunosorbent assay and monitored by OD readings at 450 nm. The number of HI-positive serum samples was calculated in each group. PBS, phosphate-buffered saline; normal, normal chow diet; HFD, high-fat diet; vaccine, influenza inactivated vaccine; HI, hemagglutination inhibition. Adapted from Kim et al. [32], with permission from Oxford University Press.
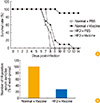
Notes
This work was supported by a grant from the Korean Healthcare Technology R&D project of the Ministry of Health & Welfare (A103001), the Basic Science Research Program of the National Research Foundation of Korea (NRF), which is funded by the Ministry of Education, Science, and Technology (2012R1A1A2039819), and a grant from GRRC at the Catholic University of Korea.
References
1. World Health Organization. WHO World Health Report 2002: reducing risks, promoting healthy life. p. 49-97 [Internet]. Geneva: World Health Organization;2003. cited 2013 Aug 17. Available from: http://www.who.int/whr/2002/index.html.
2. Rohn TA, Bachmann MF. Vaccines against non-communicable diseases. Curr Opin Immunol. 2010; 22:391–396.


3. World Health Organization. Fact Sheet 311: obesity and overweight [Internet]. Geneva: World Health Organization;2013. cited 2013 Aug 17. Available from: http://www.who.int/mediacentre/factsheets/fs311/en.
4. Eckel RH, Kahn SE, Ferrannini E, et al. Obesity and type 2 diabetes: what can be unified and what needs to be individualized? J Clin Endocrinol Metab. 2011; 96:1654–1663.


5. Schuster DP. Obesity and the development of type 2 diabetes: the effects of fatty tissue inflammation. Diabetes Metab Syndr Obes. 2010; 3:253–262.


6. Chen L, Magliano DJ, Zimmet PZ. The worldwide epidemiology of type 2 diabetes mellitus: present and future perspectives. Nat Rev Endocrinol. 2012; 8:228–236.


7. Dansinger ML, Gleason JA, Griffith JL, Selker HP, Schaefer EJ. Comparison of the Atkins, Ornish, Weight Watchers, and Zone diets for weight loss and heart disease risk reduction: a randomized trial. JAMA. 2005; 293:43–53.


8. Padwal RS, Majumdar SR. Drug treatments for obesity: orlistat, sibutramine, and rimonabant. Lancet. 2007; 369:71–77.


9. Adams TD, Gress RE, Smith SC, et al. Long-term mortality after gastric bypass surgery. N Engl J Med. 2007; 357:753–761.


10. Sjostrom L, Narbro K, Sjostrom CD, et al. Effects of bariatric surgery on mortality in Swedish obese subjects. N Engl J Med. 2007; 357:741–752.


12. Ariyasu H, Takaya K, Tagami T, et al. Stomach is a major source of circulating ghrelin, and feeding state determines plasma ghrelin-like immunoreactivity levels in humans. J Clin Endocrinol Metab. 2001; 86:4753–4758.


13. Inui A, Asakawa A, Bowers CY, et al. Ghrelin, appetite, and gastric motility: the emerging role of the stomach as an endocrine organ. FASEB J. 2004; 18:439–456.


14. Klok MD, Jakobsdottir S, Drent ML. The role of leptin and ghrelin in the regulation of food intake and body weight in humans: a review. Obes Rev. 2007; 8:21–34.


15. St-Pierre DH, Karelis AD, Cianflone K, et al. Relationship between ghrelin and energy expenditure in healthy young women. J Clin Endocrinol Metab. 2004; 89:5993–5997.


16. Zorrilla EP, Iwasaki S, Moss JA, et al. Vaccination against weight gain. Proc Natl Acad Sci U S A. 2006; 103:13226–13231.


18. Rojas JM, Stafford JM, Saadat S, Printz RL, Beck-Sickinger AG, Niswender KD. Central nervous system neuropeptide Y signaling via the Y1 receptor partially dissociates feeding behavior from lipoprotein metabolism in lean rats. Am J Physiol Endocrinol Metab. 2012; 303:E1479–E1488.


19. NHS Choice. 'Obesity vaccine' hope [Internet]. Geneva: World Health Organization;2003. cited 2013 Aug 17. Available from: http://www.nhs.uk/news/2012/07July/Pages/obesity-vaccine-fat-jab-hope.aspx.
20. Johnson JE, Chiu W. Structures of virus and virus-like particles. Curr Opin Struct Biol. 2000; 10:229–235.


21. Pumpens P, Grens E. Hepatitis B core particles as a universal display model: a structure-function basis for development. FEBS Lett. 1999; 442:1–6.


22. Kozlovska TM, Cielens I, Dreilinna D, et al. Recombinant RNA phage Q beta capsid particles synthesized and self-assembled in Escherichia coli. Gene. 1993; 137:133–137.


23. Heil F, Hemmi H, Hochrein H, et al. Species-specific recognition of single-stranded RNA via toll-like receptor 7 and 8. Science. 2004; 303:1526–1529.


24. Bessa J, Jegerlehner A, Hinton HJ, et al. Alveolar macrophages and lung dendritic cells sense RNA and drive mucosal IgA responses. J Immunol. 2009; 183:3788–3799.


25. Hou B, Saudan P, Ott G, et al. Selective utilization of Toll-like receptor and MyD88 signaling in B cells for enhancement of the antiviral germinal center response. Immunity. 2011; 34:375–384.


26. Kahn SE, Hull RL, Utzschneider KM. Mechanisms linking obesity to insulin resistance and type 2 diabetes. Nature. 2006; 444:840–846.


27. Bendtzen K, Mandrup-Poulsen T, Nerup J, Nielsen JH, Dinarello CA, Svenson M. Cytotoxicity of human pI 7 interleukin-1 for pancreatic islets of Langerhans. Science. 1986; 232:1545–1547.


28. Dinarello CA, Donath MY, Mandrup-Poulsen T. Role of IL-1beta in type 2 diabetes. Curr Opin Endocrinol Diabetes Obes. 2010; 17:314–321.
29. Bachmann MF, Jennings GT. Therapeutic vaccines for chronic diseases: successes and technical challenges. Philos Trans R Soc Lond B Biol Sci. 2011; 366:2815–2822.


30. Spohn G, Keller I, Beck M, Grest P, Jennings GT, Bachmann MF. Active immunization with IL-1 displayed on virus-like particles protects from autoimmune arthritis. Eur J Immunol. 2008; 38:877–887.


31. Marti A, Marcos A, Martinez JA. Obesity and immune function relationships. Obes Rev. 2001; 2:131–140.


32. Kim YH, Kim JK, Kim DJ, et al. Diet-induced obesity dramatically reduces the efficacy of a 2009 pandemic H1N1 vaccine in a mouse model. J Infect Dis. 2012; 205:244–251.


33. Weber DJ, Rutala WA, Samsa GP, Santimaw JE, Lemon SM. Obesity as a predictor of poor antibody response to hepatitis B plasma vaccine. JAMA. 1985; 254:3187–3189.


34. Young MD, Gooch WM 3rd, Zuckerman AJ, Du W, Dickson B, Maddrey WC. Comparison of a triple antigen and a single antigen recombinant vaccine for adult hepatitis B vaccination. J Med Virol. 2001; 64:290–298.


35. Claycombe K, King LE, Fraker PJ. A role for leptin in sustaining lymphopoiesis and myelopoiesis. Proc Natl Acad Sci U S A. 2008; 105:2017–2021.


36. Dhurandhar NV, Israel BA, Kolesar JM, Mayhew GF, Cook ME, Atkinson RL. Increased adiposity in animals due to a human virus. Int J Obes Relat Metab Disord. 2000; 24:989–996.


37. Dhurandhar NV, Israel BA, Kolesar JM, Mayhew G, Cook ME, Atkinson RL. Transmissibility of adenovirus-induced adiposity in a chicken model. Int J Obes Relat Metab Disord. 2001; 25:990–996.


38. Dhurandhar NV, Whigham LD, Abbott DH, et al. Human adenovirus Ad-36 promotes weight gain in male rhesus and marmoset monkeys. J Nutr. 2002; 132:3155–3160.


39. van Ginneken V, Sitnyakowsky L, Jeffery JE. "Infectobesity: viral infections (especially with human adenovirus-36: Ad-36) may be a cause of obesity. Med Hypotheses. 2009; 72:383–388.


40. Atkinson RL, Dhurandhar NV, Allison DB, et al. Human adenovirus-36 is associated with increased body weight and paradoxical reduction of serum lipids. Int J Obes (Lond). 2005; 29:281–286.


41. Na HN, Hong YM, Kim J, Kim HK, Jo I, Nam JH. Association between human adenovirus-36 and lipid disorders in Korean schoolchildren. Int J Obes (Lond). 2010; 34:89–93.


42. Atkinson RL, Lee I, Shin HJ, He J. Human adenovirus-36 antibody status is associated with obesity in children. Int J Pediatr Obes. 2010; 5:157–160.


43. Trovato GM, Castro A, Tonzuso A, et al. Human obesity relationship with Ad36 adenovirus and insulin resistance. Int J Obes (Lond). 2009; 33:1402–1409.

