Abstract
The anti-tuberculosis Bacille de Calmette et Guérin (BCG) vaccine was developed between 1905 and 1921 at Pasteur Institutes of Lille in France, and was adopted by many countries. BCG strains comprise natural mutants of major virulence factors of Mycobacterium tuberculosis and that BCG sub-strains differ markedly in virulence levels. The tuberculosis became endemic in Korea after the Korean War (1950s). The BCG strain, which was donated by Pasteur Institutes, was brought to Korea in 1955, and the first domestic BCG vaccine was produced by the National Defense Research Institute (NDRI), current Korea Centers for Disease Control and Prevention (KCDC), in 1960. Since 1987, BCG manufacture work was handed over to the Korean Institute of Tuberculosis (KIT), the freeze-dried BCG vaccine was manufactured at a scale required to meet the whole amount of domestic consumption. However, since 2006, the manufacture of BCG vaccine suspended and the whole amount of BCG was imported at this point of time. Now KIT is planning to re-produce the BCG vaccine in Korea under the supervision of KCDC, this will be render great role to National Tuberculosis Control Program (NTP) and provide initiating step for developing new tuberculosis vaccines in Korea.
The typical features of spinal tuberculosis (TB) had been identified in Egyptian mummies dated between 3000 and 2400 BC. The first evidence of the infection in humans was found in a cemetery near Heidelberg, in the Neolithic bone remains and some scholars call TB the first disease known to mankind [1,2].
In 1882, Robert Koch discovered the disease-causing agent, known as Mycobacterium tuberculosis.
In 1908, Albert Calmette and Camille Guérin, at the Institute Pasteur in Lille, isolated a virulent mycobacterium from a cow with tuberculous mastitis and attenuated the purified strain by serial passage over thirteen years on a glycerol-potato-bile medium [3]. The Bacille de Calmette et Guérin (BCG) vaccine was first used to immunize humans in 1921. BCG became widespread after its introduction into World Health Organization (WHO) Expended Program on Immunization (EPI) in 1974. At present, about 100 million children receive BCG vaccine each year in the world [4,5].
A key aspect of BCG vaccine nativity may concern the nature of BCG attenuation; the mechanisms of BCG attenuation remain largely unknown. This is further complicated by the fact that BCG is not a single strain, but instead comprises a number of sub-strains that exhibit phenotypic and biochemical differences. BCG strains also exhibit differences in residual virulence level [6-8].
The initial phase (1908-1921) comprises the 230 times in vitro passages conducted by Calmette and Guérin to produce the original vaccine. The second phase starts circa 1924 with widespread use and distribution of BCG. It ends several decades, and hundreds of passages, later (in 1966 for BCG Pasteur 1173P2, but different years for different sub-strains) with the establishment of frozen seed lots.
The loss of genomic regions during in vitro passaging is believed to delete not only the virulence factors but also some certain key protective antigens, hence resulting in impotency of BCG. Therefore, some researchers insist supplementing BCG with the missing immune-dominant region of difference (RD) antigens appears to be a promising strategy to restore its prophylactic potential.
The original strain of BCG, maintained at the Pasteur Institute in Lille, France, produced hundreds of "daughter" strains and became the progenitor of the most commonly used vaccines, the Danish strain, originating in 1931 as the 423rd transfer; the Tokyo strain, sent from France as a seed culture in 1925; and the Glaxo strain, derived from the 1077th transfer of the Danish strain.
As early as 1924, cultures of BCG were distributed by the Institute Pasteur to laboratories around the world. By 1926, at least 34 countries had received cultures from the Pasteur Institute. In 1927, another 26 countries received cultures of BCG (Fig. 1) [9].
With the lyophilization techniques, "seed-lot system" was introducedas part of the 'Requirements for Dried BCG Vaccine' initiated by WHO (WHO Expert Committee on Biological Standardization, 1966), and the process of in vitro evolution was discontinued.
Using subtraction hybridization, DNA microarrays and bacterial artificial chromosome arrays have provided information about 16 regions of the genome of M. tuberculosis that are lacking in BCG strain [11-13].
RD1 was absent from all BCG sub-strains but conserved in all virulent laboratory and clinical isolates of M. bovis and M. tuberculosis. The deletion of RD1 occurred before 1921 during the development of original BCG. Gene disruption and reintroduction studies had been conducted and then established that RD1-encoded genes were required for the full virulence of M. tuberculosis [14-16]. BCG complemented with RD1 initiate recruitment and activation of immune cells is comparable with that of fully virulent M. tuberculosis H37Rv [17]. RD1 is a 9.5 kb DNA segment deletion which includes Rv3871-3879C encodes 14 open reading frames (ORFs) with codon usage patterns consistent with those of expressed genes of M. tuberculosis [18]. The genes encoding ESX-1 which is required for release of a culture filtrate protein 10 kDa (CFP-10) and early secreted antigenic target 6 kDa (ESAT-6), two immune-dominant proteins useful in the immune-diagnosis in TB [19,20]. RD1 contains three main T-cell antigens, Rv3873a, ESAT-6, and CFP-10. ESAT-6 and CFP-10 induce strong antigen-induced interferon (IFN)-γ responses and T-cell proliferation in TB patients but weak in BCG-vaccinated healthy ones [21]. Another important T-cell antigen, encoded by the RD1 region, is Rv3873a PPE protein (PPE68). Rv3873a stimulated high levels of IFN-γ secretion in peripheral blood mononuclear cells isolated from TB patients [22].
Liu et al. [23] investigated another gene products encoded by RD1 (Rv3873, Rv3878, and Rv3879c). Peptides from each protein were designed to evaluate human T-cell response, and 45% and 53% of TB patients responded to Rv3879c and Rv3873 [23].
Rv3615c (=ESX-1 substrate protein C, EspC), encoded outside RD1, is a highly immune-dominant RD1-dependent secreted antigen. Rv3615c was secreted in an ESX-1 dependent fashion and immune-dominant as ESAT-6 and CFP-10 in TB infection. The high immune-dominance makes it a profound candidate for another TB vaccine development and immunediagnosis marker screening [24].
This 10.7 kb DNA segment is conserved in all virulent laboratory M. tuberculosis and clinical tubercle bacilli. But it is deleted from sub-strains of BCG Pasteur. These sub-strains arederived from the original BCG Pasteur strain between 1927 and 1931 [11]. The M. bovis RD2 region contains 11 ORFs with GC-rich codon usage.
Among them, Rv1980c encodes the immunogenic protein Mpt-64, which was investigated as an M. tuberculosis-specific secreted antigen was not expressed in the late BCG strains. Mpt-64 a 23-kDa secreted protein was reported as diagnostic candidate recognized by human T-cells. On stimulation with Mpt-64, the specific T-cell lines secreted IFN-γ but not interleukin-4. A minority of TB patients mounted an antibody response to Mpt-64 [25,26]. Mustafa et al. [27] suggests that Mpt-64 also have a role in inhibition of apoptosis of the M. tuberculosis infected cells and allowing mycobacteria to survive in macrophages [27]. CFP-21 protein is encoded in the RD2 region, and the diagnostic potential of this antigen was already evaluated. CFP-21 induced delayed-type hypersensitivity (DTH) in guinea pigs, which evaluated both in animals vaccinated with BCG and infected with M. tuberculosis. However, CFP-21 induced significantly high level of DTH response in the H37Rv-infected animals than the BCG-vaccinated ones [28].
Rv1989c, Rv1978, Rv1981c, and Rv1985c also encoded by RD2, were investigated for T-cell-based diagnosis of TB [23,29,30]. The loss of RD2 has an important influence on the protective efficacy of different BCG sub-strains. Previous study demonstrated that CFP-21 and MPT-64 fusion protein could stimulate higher level of IFN-γ secretion in tuberculin skin test-positive population. In immunogenicity studies, different combinations of antigens ESAT-6/CFP-10/CFP-21/MPT-64 with BCG supplementation resulted in significantly greater protection against experimental murine TB than BCG alone. The combination of RD2 antigen only was found to release cytokines as much as substantial levels [31,32].
Using comparative genomics (BAC array), Brosch et al. [33], identified two large tandem duplications of 29 and 36 kb (DU1 and DU2) in BCG Pasteur 1173P2. DU1 is restricted to BCG Pasteur and containing the region RNA polymerase sigma factor M (sigM)-para-aminobenzoate synthase component II (pabA)(Rv3911-Rv0013). DU1 represents a tandem genetic duplication because it results in a strain with two copies of oriC, the site of chromosomal origin of replication, and genes encoding key components of the chromosomal replication and cell division machinery [34]. The dnaA-dnaN region in DU1 is regarded as the functional origin of replication for mycobacteria. BCG Pasteur has two copies of dnaA-dnaN region and both copies of oriC should be functional [19,33]. In addition, to those involved in the replication initiation and cell division, DU1 is a coding sequence for DNA gyrase, two tRNAs, SigM, and a thioredoxin-thioredoxin reductase system [19].
DU2, the second large genomic rearrangement has been detected in all BCG sub-strains with different sizes (DU2-I-DU2-IV). DU2-I has been detected in the early strains; BCG Moreau, Russia, and Japan. BCG Birkhaug and Sweden displayed DU2-II, and DU2-III existed in the genome of BCG Prague, Glaxo, and Danish. In BCG Pasteur, DU2-IV, several regulatory genes including sigH and whiB1 occurs that might affect replication gene expression levels.
RD14 is a 9.1 kb region that containing 10 genes (Rv1765-Rv1773) had been deleted in BCG Pasteur while present in all other BCG sub-strains. The limited distribution of this deletion occurred in between 1938 and 1961. The biological impact of RD14 is nearly unknown, but the genes include a number of the Rv1768 (PE-PGRS family), Rv1771 (gulonolactone dehydrogenase) and several uncharacterized ORFs [12,39]. A previous study revealed Rv1773, a transcriptional repressor regulates expression of two genes, Rv1774 and Rv1775 [40].
RD16 is a 7.6 kb region that containing 6 ORFs (Rv3400-Rv3405c) is totally absent from only BCG-Moreau. Honda et al. [41] suggested an association between colony morphology and RD16 genotype of BCG-Tokyo strains. There were two colony types which showed differences in the presence or absence of 22 bp deletion in Rv3405c of the RD16. It was suggested that Rv3405c might be influencing on colony morphology through some changes in surface molecules of the bacilli [41,42]. Previous study showed that the phenolic glycolipid and phthiocerol dimycocerosate are involved with RD16 in the expression. However, Recent study revealed that their biosynthesis is caused by phenolpthiocerol synthesis type-I polyketide synthase (ppsA) gene mutation. Their biosynthesis is not regulated by the RD16 [43,44].
n-RD18 is a 1.5 kb DNA segment deletion only deleted in BCG sub-strains (BCG Pasteur, Phipps, Frappier, Connaught, Tice) obtained after 1933 [45]. n-RD18 is containing the genes encoding SigI, an alternative RNA polymerase sigma factor. SigI is present in M. tuberculosis and M. bovis but is absent in M. avium, M. paratuberculosis, M. marium, and M. smegmasis [46]. However, the role of SigI in virulence of BCG strains remains unknown.
The first systematical study for efficacy of BCG was conducted by Olaf Scheel and Johannes Heimbeck at Ullevål Hospital in Oslo. About half of the students were tuberculin skin test (TST) negative at the time of entry, but nearly all of them became infected with TB during their 3-year training. At that time BCG vaccination had recently introduced by Calmette. Heimbeck [47] offered BCG vaccination to TST negative individuals and it makes them to maintain a similar low risk of TB as those with positive TSTs. Early in 1943, an epidemic of TB was occurred. The 368 pupils have been followed up for a period of 12 years, and the protection against TB was 87-96% [48].
Extensive trials to assess the protective efficacy of BCG against pulmonary TB were provided. The observed vaccine efficacy ranged from 0% to 80%. To quantify the efficacy of BCG vaccine against TB, a meta-analysis was undertook including 14 prospective trials and 12 case-control studies. In these trials, the average protection against TB was 51% and in the case-control studies was 50%. The protective effect against TB-related death was 71% [49].
There is no conclusive evidence available to show which BCG strain offers better protection from TB in human. All different BCG strains differ in terms of their immunogenicity, efficacy and side effects. A number of studies have measured the ability of different vaccines. Gaisford and Griffiths [50], used two forms of vaccines, BCG Copenhagen and Pasteur, and another study has evaluated the efficacy of BCG Glaxo and Tokyo vaccines in South Africa [51]. In a previous study by Vallishayee et al. [52], 11 different BCG vaccines were compared by means of vaccinating children in India and Denmark. Only the BCG Prague strain induced delayed hypersensitivity strikingly weaker than that induced by any of the others (BCG Danish 1331, Tokyo 172, Pasteur 1173P2, etc.) [52]. Several studies reported the protective efficacy of BCG vaccines. The study conducted in Myanmar in 1982 showed 38% and 60% in South Africa in 1972-1976 against all forms of disease where the BCG-Tokyo vaccine used, and 74% against TB in Korea where Pasteur 1173P2 strain was used (Table 1)[51,53-59].
Commonly used and internationally distributed BCG vaccine strains were BCG Japan (Tokyo172), BCG Danish (Copenhagen 1331), BCG Pasteur 1173P2, etc., and these were categorized in Table 2 [60].
In 1924, Dr. Kiyoshi Shiga introduced a BCG strain to Japan from the Pasteur Institute in France. The strain has been propagated for research purposes, and large-scale production was instituted in 1946-1947. At the National Institute of Health, the 172nd passage of BCG was freeze-dried in 1961, named BCG-Tokyo 172 strain. In 1965, the Tokyo strain was registered as an International reference preparation of BCG vaccine by the WHO. In 1967, a multiple puncture method using a plastic cylinder was introduced to Japan, thereafter; the multiple puncture vaccination method replaced intradermal injections in Japan [61]. The present countries using Tokyo 172 strain are Japan, Bangladesh, Pakistan, Saudi Arabia, Taiwan, and Thailand, etc.
BCG vaccination were carried out in Denmark in 1927 by K. A. Jansen, however new strain, known as BCG Danish, was obtained from Pasteur Institute in 1931 [62]. BCG Danish is a synonym for BCG Denmark. BCG Prague (=Czechoslovakian) and BCG Glaxo 1077 were obtained from Danish in 1947 and 1954. In 1960, Danish 1331 were freeze-dried, and this batch was adopted as the primary seed-lot in 1966 [9,63]. More than 35 countries including Austria, Belgium, and Korea using BCG Danish manufactured from Statens Serum Institute (SSI), in Copenhagen.
The BCG Pasteur vaccine was first developed between 1905 and 1921 at Pasteur Institutes in France. Once safety had been confirmed, BCG was disseminated between 1921 and Calmette's death in 1933. Different laboratories maintained their own daughter strains by passaging, until the introduction of archival seed lots in the 1960s. Present Pasteur strain was lyophilized in 1961, thus M. bovis BCG Pasteur 1173P2 corresponds to the archive established after 1,173 passages. Genomic comparisons indicate that BCG Pasteur has undergone two duplications, one SNP, and four deletions between Pasteur 1921 and 1961 [33]. The entire genome sequences of BCG Pasteur 1173P2 has been published in 2007. The Pasteur strain contains two copies of DU1 which is restricted to BCG Pasteur only. There are several Countries and Institutes where they using BCG-Pasteur 1173P2 strains for vaccine production (Table 3).
In 1920s, BCG vaccination was initiated and daughter strains were distributed from Pasteur Institute when Korea was under Japanese imperialism. During this time, only little number of children in Korea was BCG vaccinated even though Japan had already produced liquid BCG vaccine. Japan succeeded in freeze-dried BCG vaccine production in 1943 and the actual mass production of dried BCG vaccine initiated in 1947.
During the Korean War, Korean government began the national BCG vaccination project in 1952, where the vaccine was provided by Japan Anti-Tuberculosis Association (JATA). In 1953, Korean National Tuberculosis Association (KNTA) was established and BCG vaccination project expanded significantly in a more systematical way.
In 1954, Korean government dispatched Mr. Hyun-gyu Kim, the bacteriologist of National Institution of Prevention & Epidemiology (NIPE, now changed to National Institute of Health), to SSI in Copenhagen under the support of United Nations Korean Reconstruction Agency (UNKRA). After Mr. Hyun-gyu Kim's visit, he stopped off at Pasteur Institution his wayover he brought BCG-Pasteur strain to Korea.
In 1956, research on a liquid BCG vaccine production had begun in NIPE, and the mass production of liquid BCG vaccine (20,000 mL) was initiated in 1960. Finally, BCG vaccine produced by NIPE was approved to be publicly used by the WHO in 1963.
In 1972, Korean Natioanl Institutes of Health (KNIH) decided to transfer the main role in BCG Production to KNTA and Minister of Health and Social Affairs (MHSA); now changed as Ministry of Health and Welfare (MHW); promoted researches on freeze-dried BCG vaccine with KNTA.
Actual production of freeze-dried BCG vaccine was started and the potency was proved by WHO in 1977. From 1978, freeze-dried BCG vaccine for 50 persons (5 mL/ampoule), 20 persons (2 mL/ampoule) was regularly produced.
In 1987, responsibility for BCG vaccine production was handed over from NIH to KNTA and the following year, KNTA produced sufficient nationwide consumable amount of BCG vaccines.
In 1988, Japan BCG vaccine import stopped which had 50% of market shares in Korea.
BCG vaccine which had been used for National Tuberculosis Program was produced from 1960 and halted temporally in 2006. At least for the upcoming five years, the domestic need for BCG vaccination of Korea depends entirely on imports from SSI and Japan-BCG.
In 2008, KNTA has contracted BCG production to Pharmaceutical Company, which had well established modernized good manufacturing practice (GMP) facilities funded by KNTA last year. Korean Institute of Tuberculosis (KIT), KNTA's affiliated research institute, has developed BCG-Korea strain and its whole genome sequencing is finished recently (nucleotide sequence accession number; the complete genome sequence of M. bovis BCG Korea 1168P has been assigned GenBank accession number CP003900). Additional characterization is undergoing with proteome analysis of the mother strain and standardizing the production process. After accomplishing non-clinical and clinical trials, high quality BCG vaccine will be distributed before 2017.
Figures and Tables
Fig. 1
Refined genealogy of BCG vaccines, modified from Liu et al. [10]. M. bovis, Mycobacterium bovis; BCG, Bacillus de Calmette et Guérin; RD, region of difference.
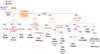
Table 1
Summary of the results of some studies on the protective efficacy of BCG vaccine, adapted from Milstien et al. [53]
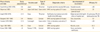
Table 2
Commonly used and internationally distributed BCG vaccine strains, adapted from Ritz and Curtis [60]
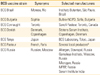
References
1. Madkour MM, Warrell DA. Tuberculosis. Berlin: Springer;2004.
2. Zink AR, Sola C, Reischl U, et al. Characterization of Mycobacterium tuberculosis complex DNAs from Egyptian mummies by spoligotyping. J Clin Microbiol. 2003; 41:359–367.


3. Calmette LC, Guérin C, Weill-Hallé B. Essais d'immunisation contre l'infection tuberculeuse. Rev Tuberc. 1924; 5:481–491.
4. Bannister C, Bennett L, Carville A, Azzopardi P. Evidence behind the WHO guidelines: hospital care for children: what is the evidence that BCG vaccination should not be used in HIV-infected children? J Trop Pediatr. 2009; 55:78–82.


5. World Health Organization. BCG vaccine. WHO position paper. Wkly Epidemiol Rec. 2004; 79:27–38.
7. Dubos RJ, Pierce CH. Differential characteristics in vitro and in vivo of several substrains of BCG. I. Multiplication and survival in vitro. Am Rev Tuberc. 1956; 74:655–666.
8. Leung AS, Tran V, Wu Z, et al. Novel genome polymorphisms in BCG vaccine strains and impact on efficacy. BMC Genomics. 2008; 9:413.


9. Oettinger T, Jorgensen M, Ladefoged A, Haslov K, Andersen P. Development of the Mycobacterium bovis BCG vaccine: review of the historical and biochemical evidence for a genealogical tree. Tuber Lung Dis. 1999; 79:243–250.


10. Liu J, Tran V, Leung AS, Alexander DC, Zhu B. BCG vaccines: their mechanisms of attenuation and impact on safety and protective efficacy. Hum Vaccin. 2009; 5:70–78.


11. Mahairas GG, Sabo PJ, Hickey MJ, Singh DC, Stover CK. Molecular analysis of genetic differences between Mycobacterium bovis BCG and virulent M. bovis. J Bacteriol. 1996; 178:1274–1282.


12. Behr MA, Wilson MA, Gill WP, et al. Comparative genomics of BCG vaccines by whole-genome DNA microarray. Science. 1999; 284:1520–1523.


13. Gordon SV, Brosch R, Billault A, Garnier T, Eiglmeier K, Cole ST. Identification of variable regions in the genomes of tubercle bacilli using bacterial artificial chromosome arrays. Mol Microbiol. 1999; 32:643–655.


14. Pym AS, Brodin P, Brosch R, Huerre M, Cole ST. Loss of RD1 contributed to the attenuation of the live tuberculosis vaccines Mycobacterium bovis BCG and Mycobacterium microti. Mol Microbiol. 2002; 46:709–717.


15. Hsu T, Hingley-Wilson SM, Chen B, et al. The primary mechanism of attenuation of bacillus Calmette-Guerin is a loss of secreted lytic function required for invasion of lung interstitial tissue. Proc Natl Acad Sci U S A. 2003; 100:12420–12425.


16. Lewis KN, Liao R, Guinn KM, et al. Deletion of RD1 from Mycobacterium tuberculosis mimics bacille Calmette-Guerin attenuation. J Infect Dis. 2003; 187:117–123.


17. Majlessi L, Brodin P, Brosch R, et al. Influence of ESAT-6 secretion system 1 (RD1) of Mycobacterium tuberculosis on the interaction between mycobacteria and the host immune system. J Immunol. 2005; 174:3570–3579.


18. Amoudy HA, Al-Turab MB, Mustafa AS. Identification of transcriptionally active open reading frames within the RD1 genomic segment of Mycobacterium tuberculosis. Med Princ Pract. 2006; 15:137–144.


19. Cole ST, Brosch R, Parkhill J, et al. Deciphering the biology of Mycobacterium tuberculosis from the complete genome sequence. Nature. 1998; 393:537–544.


20. Berthet FX, Rasmussen PB, Rosenkrands I, Andersen P, Gicquel B. A Mycobacterium tuberculosis operon encoding ESAT-6 and a novel low-molecular-mass culture filtrate protein (CFP-10). Microbiology. 1998; 144(Pt 11):3195–3203.


21. Mustafa AS, Al-Attiyah R, Hanif SN, Shaban FA. Efficient testing of large pools of Mycobacterium tuberculosis RD1 peptides and identification of major antigens and immunodominant peptides recognized by human Th1 cells. Clin Vaccine Immunol. 2008; 15:916–924.


22. Okkels LM, Brock I, Follmann F, et al. PPE protein (Rv3873) from DNA segment RD1 of Mycobacterium tuberculosis: strong recognition of both specific T-cell epitopes and epitopes conserved within the PPE family. Infect Immun. 2003; 71:6116–6123.


23. Liu XQ, Dosanjh D, Varia H, et al. Evaluation of T-cell responses to novel RD1- and RD2-encoded Mycobacterium tuberculosis gene products for specific detection of human tuberculosis infection. Infect Immun. 2004; 72:2574–2581.


24. Millington KA, Fortune SM, Low J, et al. Rv3615c is a highly immunodominant RD1 (region of difference 1)-dependent secreted antigen specific for Mycobacterium tuberculosis infection. Proc Natl Acad Sci U S A. 2011; 108:5730–5735.


25. Li H, Ulstrup JC, Jonassen TO, Melby K, Nagai S, Harboe M. Evidence for absence of the MPB64 gene in some substrains of Mycobacterium bovis BCG. Infect Immun. 1993; 61:1730–1734.


26. Roche PW, Feng CG, Britton WJ. Human T-cell epitopes on the Mycobacterium tuberculosis secreted protein MPT64. Scand J Immunol. 1996; 43:662–670.


27. Mustafa T, Wiker HG, Morkve O, Sviland L. Reduced apoptosis and increased inflammatory cytokines in granulomas caused by tuberculous compared to non-tuberculous mycobacteria: role of MPT64 antigen in apoptosis and immune response. Clin Exp Immunol. 2007; 150:105–113.


28. Weldingh K, Andersen P. Immunological evaluation of novel Mycobacterium tuberculosis culture filtrate proteins. FEMS Immunol Med Microbiol. 1999; 23:159–164.


29. Chen J, Su X, Zhang Y, et al. Novel recombinant RD2- and RD11-encoded Mycobacterium tuberculosis antigens are potential candidates for diagnosis of tuberculosis infections in BCG-vaccinated individuals. Microbes Infect. 2009; 11:876–885.


30. Chen J, Wang S, Zhang Y, et al. Rv1985c, a promising novel antigen for diagnosis of tuberculosis infection from BCG-vaccinated controls. BMC Infect Dis. 2010; 10:273.


31. Kalra M, Grover A, Mehta N, et al. Supplementation with RD antigens enhances the protective efficacy of BCG in tuberculous mice. Clin Immunol. 2007; 125:173–183.


32. Wang C, Chen Z, Fu R, et al. A DNA vaccine expressing CFP21 and MPT64 fusion protein enhances BCG-induced protective immunity against Mycobacterium tuberculosis infection in mice. Med Microbiol Immunol. 2011; 200:165–175.


33. Brosch R, Gordon SV, Buchrieser C, Pym AS, Garnier T, Cole ST. Comparative genomics uncovers large tandem chromosomal duplications in Mycobacterium bovis BCG Pasteur. Yeast. 2000; 17:111–123.


34. Salazar L, Fsihi H, de Rossi E, et al. Organization of the origins of replication of the chromosomes of Mycobacterium smegmatis, Mycobacterium leprae and Mycobacterium tuberculosis and isolation of a functional origin from M. smegmatis. Mol Microbiol. 1996; 20:283–293.


35. Mehra S, Kaushal D. Functional genomics reveals extended roles of the Mycobacterium tuberculosis stress response factor sigmaH. J Bacteriol. 2009; 191:3965–3980.


36. Manganelli R, Voskuil MI, Schoolnik GK, Dubnau E, Gomez M, Smith I. Role of the extracytoplasmic-function sigma factor sigma(H) in Mycobacterium tuberculosis global gene expression. Mol Microbiol. 2002; 45:365–374.


37. Brosch R, Gordon SV, Garnier T, et al. Genome plasticity of BCG and impact on vaccine efficacy. Proc Natl Acad Sci U S A. 2007; 104:5596–5601.


38. Kernodle DS. Warning: differences in the copy number of duplication unit 2 (DU2) within BCG Danish 1331 may influence findings involving genetically modified BCG Danish strains. Vaccine. 2012; 30:6013–6014.


39. Wolucka BA, Communi D. Mycobacterium tuberculosis possesses a functional enzyme for the synthesis of vitamin C, L-gulono-1,4-lactone dehydrogenase. FEBS J. 2006; 273:4435–4445.


40. Alexander DC, Behr MA. Rv1773 is a transcriptional repressor deleted from BCG-Pasteur. Tuberculosis (Edinb). 2007; 87:421–425.


41. Honda I, Seki M, Ikeda N, et al. Identification of two subpopulations of Bacillus Calmette-Guerin (BCG) Tokyo172 substrain with different RD16 regions. Vaccine. 2006; 24:4969–4974.


42. Bedwell J, Kairo SK, Behr MA, Bygraves JA. Identification of substrains of BCG vaccine using multiplex PCR. Vaccine. 2001; 19:2146–2151.


43. Naka T, Maeda S, Niki M, et al. Lipid phenotype of two distinct subpopulations of Mycobacterium bovis Bacillus Calmette-Guerin Tokyo 172 substrain. J Biol Chem. 2011; 286:44153–44161.


44. Chen JM, Islam ST, Ren H, Liu J. Differential productions of lipid virulence factors among BCG vaccine strains and implications on BCG safety. Vaccine. 2007; 25:8114–8122.


45. Mostowy S, Tsolaki AG, Small PM, Behr MA. The in vitro evolution of BCG vaccines. Vaccine. 2003; 21:4270–4274.


46. Manganelli R, Provvedi R, Rodrigue S, Beaucher J, Gaudreau L, Smith I. Sigma factors and global gene regulation in Mycobacterium tuberculosis. J Bacteriol. 2004; 186:895–902.


47. Heimbeck J. Tuberkuloseschutzmittel BCG, Prinzipien and Resultate. Schweiz Z Tuberk. 1949; 6:209–224.
48. Hyge TV. The efficacy of BCG-vaccination; epidemic of tuberculosis in a State School, with an observation period of 12 years. Acta Tuberc Scand. 1956; 32:89–107.
49. Colditz GA, Brewer TF, Berkey CS, et al. Efficacy of BCG vaccine in the prevention of tuberculosis. Meta-analysis of the published literature. JAMA. 1994; 271:698–702.


50. Gaisford W, Griffiths M. B.C.G. vaccination in the newborn; preliminary report. Br Med J. 1951; 2:702–705.
51. Cartwright JD. BCG vaccination of the newborn. S Afr Med J. 1978; 54:65–67.
52. Vallishayee RS, Shashidhara AN, Bunch-Christensen K, Guld J. Tuberculin sensitivity and skin lesions in children after vaccination with 11 different BCG strains. Bull World Health Organ. 1974; 51:489–494.
53. Milstien JB, Gibson JJ. Quality control of BCG vaccine by WHO: a review of factors that may influence vaccine effectiveness and safety. Bull World Health Organ. 1990; 68:93–108.
54. Myint TT, Win H, Aye HH, Kyaw-Mint TO. Case-control study on evaluation of BCG vaccination of newborn in Rangoon, Burma. Ann Trop Paediatr. 1987; 7:159–166.


55. Zilber N, Simchen E, Wartski S, Marcus JH, Shakya GR. Effect of mass BCG vaccination at birth on the incidence of tuberculosis among Jewish children in Israel. Isr J Med Sci. 1984; 20:1150–1157.
56. Padungchan S, Konjanart S, Kasiratta S, Daramas S, ten Dam HG. The effectiveness of BCG vaccination of the newborn against childhood tuberculosis in Bangkok. Bull World Health Organ. 1986; 64:247–258.
57. Young TK, Hershfield ES. A case-control study to evaluate the effectiveness of mass neonatal BCG vaccination among Canadian Indians. Am J Public Health. 1986; 76:783–786.


58. Tidjani O, Amedome A, ten Dam HG. The protective effect of BCG vaccination of the newborn against childhood tuberculosis in an African community. Tubercle. 1986; 67:269–281.


59. Jin BW, Hong YP, Kim SJ. A contact study to evaluate the BCG vaccination programme in Seoul. Tubercle. 1989; 70:241–248.


60. Ritz N, Curtis N. Mapping the global use of different BCG vaccine strains. Tuberculosis (Edinb). 2009; 89:248–251.


61. Yamamoto S, Yamamoto T. Historical review of BCG vaccine in Japan. Jpn J Infect Dis. 2007; 60:331–336.