Abstract
Tularemia is a high-risk infectious disease caused by Gram-negative bacterium Francisella tularensis. Due to its high fatality at very low colony-forming units (less than 10), F. tularensis is considered as a powerful potential bioterrorism agent. Vaccine could be the most efficient way to prevent the citizen from infection of F. tularensis when the bioterrorism happens, but officially approved vaccine with both efficacy and safety is not developed yet. Research for the development of tularemia vaccine has been focusing on the live attenuated vaccine strain (LVS) for long history, still there are no LVS confirmed for the safety which should be an essential factor for general vaccination program. Furthermore the LVS did not show protection efficacy against high-risk subspecies tularensis (type A) as high as the level against subspecies holarctica (type B) in human. Though the subunit or recombinant vaccine candidates have been considered for better safety, any results did not show better prevention efficacy than the LVS candidate against F. tularensis infection. Currently there are some more trials to develop vaccine using mutant strains or nonpathogenic F. novicida strain, but it did not reveal effective candidates overwhelming the LVS either. Difference in the protection efficacy of LVS against type A strain in human and the low level protection of many subunit or recombinant vaccine candidates lead the scientists to consider the live vaccine development using type A strain could be ultimate answer for the tularemia vaccine development.
Francisella tularensis causing zoonotic infectious disease "tularemia" is Gram-negative facultative non-motile intracellular coccobacillus bacteria considered as a potential bioterrorism agent because subspecies tularensis classified as type A is a high-risk pathogen which can cause severe symptoms when infected with only 10 colony-forming units or less [1-3].
F. tularensis is largely classified as four subspecies: subspecies tularensis, subspecies holarctica, subspecies mediasiatica, and subspecies novicida (Table 1). Among the subspecies, subspecies tularensis is called as type A causing high fatality if not properly treated with antibiotics, and another subspecies holarctica is called as type B showing comparatively much less severe symptom. Subspecies mediasiatica has low pathogenicity and it is distributed locally in Central Asia. Subspecies novicida is used for study of microbiological characteristics and vaccine research without biosafety level 3 facilities because it is known as a nonpathogenic in human [4]. The results from current bioinformatic study revealed that there are remarkable differences in genomic sequence between subspecies, and some taxonomists insist on the distinguished new species as Francisella novicida instead of the Francisella tularensis subsp. novicida [5]. Both strains of tularensis and holarctica among four subspecies are responsible for human pathogenesis of tularemia, and subspecies tularensis causes severe pathogenic symptoms such as fever, edema and influenza-like illness comparing mild symptoms raised by subspecies holarctica. In some cases [6,7], subspecies tularensis finally can lead the infected patients to death if they are not properly treated with antibiotics though the symptoms and severity are different according to the infection routes [8]. Although major route of infection is dermal penetration by tick-biting from rabbits as a vector, F. tularensis can infect human through the respiratory ducts or oral routes [9]. Usually man-to-man transmission is not considered to be happened because any cases infected by the bacteria transmission through the air flow were not reported yet, however it is not totally impossible to infect human within close distance by the saliva droplets from infected patient's cough to another human's nostrils or mouth. Ticks from rabbits are the most common vectors for tularemia infection in United States, but the deer flies from small rodents are also can be a vector to deliver the bacteria in western area of North America and mosquitoes are vectors and reservoir in Northern Europe including Russia and Sweden (Fig. 1).
F. tularensis generally enters the inside of phagosome by the phagocytosis of macrophage (Fig. 2), however many captured bacteria are not scavenged by the macrophage. The survived bacteria usually replicate themselves enough to infect other adjacent cells, and then the quickly growing population of bacteria raises the pathogenic symptoms after eruption from infected cells [10,11]. Mutation of the genes in Francisella pathogenic islands (FPI) and key virulence factors usually inhibits the ability of bacteria escaping from phagosome, and the mutants are considered as possible live vaccine strains.
Tularemia was firstly discovered as an infectious disease from wild animals such as rabbits by Edward Francis at Tulare County of United States in 1912. Since the first diagnosis of human tularemia was happened and the bacteria was isolated by G. W. McCoy at the Plaque laboratory of the US Public Health Services in 1912, some levels of endemic cases were continuously reported in North America, and Eurasia, especially in the regions with cold winter [12]. The oldest history about human outbreak by tularemia in Canaan region was recorded during the 17th century BC, also history tells the long-lasting endemic tularemia in Eastern Mediterranean area and Middle East Asia in the 14th century BC. In Japan, a rabbit-transmitting infectious disease named "Yatobito"was described in its medical history written in the 18th century. As a modern history, the outbreak with one fatality at Martha's Vineyard in 2000 reminded the US Center for Disease Control and Prevention that the aerosolized F. tularenisis can be a fatal bio-threat and another outbreak occurred in Kosovo of Europe in 2000 [13]. Well-known tularemia endemic place is Gori region in Eurasian country Georgia and there was the last outbreak in 2006 [12].
F. tularensis can be used as a bioterrorism agent because of its high infectivity and mortality without treatment. Furthermore, it is easy to make aerosolized F. tularensis and the decontamination is easy comparing Bacillus anthracis or other agents. There are some reports that former Soviet Union developed F. tularensis as a bio-weapon [14], and other countries such as United States and Japan focused on F. tularensis and made provision against risk of bio-threat by the bacteria. Kenneth Alibek, a bio-weapon scientist of Soviet Union, has alleged the tularemia among German soldiers in Stalingrad by release of the bacteria from Soviet forces [15]. In 1954, United States also performed the research for practical use of F. tularensis as a biological weapon. Type A strain Schu S4 was standardized as a highly pathogenic bacteria [16]. Although vaccination is the best prevention for bioterrorism, there is no officially approved vaccine to date against tularemia due to the various issues including safety concern about live bacteria candidates.
Tularemia vaccine development was started by several western countries from 1940's when the F. tularensis was firstly considered as a potential biological weapon. Similar to the common history of any vaccine development, development procedure for tularemia vaccine was also tried in both types of whole bacteria vaccine and subunit vaccine including split or recombinant forms. Among the types of whole vaccine, killed vaccine may not be considered possible format to be developed because the most trials including the heat-inactivated or the formalin-treated did not show enough efficacies to protect against F. tularensis infection. Actually the first trial for tularemia vaccine development was split vaccine. In 1940's, Foshay group tried to develop killed vaccine, which was conserved in phenol after acid extraction of the bacteria. This candidate was considered as a very safe form and it was tested for human volunteers in 1933 and 1941. However the researchers did not get clear results about protective efficacy because of the limited clinical information about the vaccinated group. Foshay's this trial was the first vaccine trial against tularemia even though it did not show enough efficacies in the animal models either [17].
After 1940's, live attenuated strains were developed to be used as a vaccine by former USSR [2,3], and this type of live attenuated vaccine strain (LVS) is considered as the most efficient vaccine candidate which is limitedly used for tularemia researchers as an unofficial vaccine. However, the LVS is live bacteria still having potential risk to replicate while the immunogenicity is also reduced by the attenuation of pathogenicity. Most scientists studying tularemia vaccine still keep trying to develop better mutant strains with higher immunogenicity and lower pathogenicity. Currently, advanced countries for tularemia vaccine research, such as United States and Sweden, are trying to develop recombinant vaccine which is considered as a type bearing high protection efficacy with guaranteed safety [1].
The first meaningful attempt for tularemia vaccine development was accomplished by former USSR before 1950's. Scientist of USSR have repeatedly cultured F. tularensis and selected colony which have less virulence with various conditions, and F. tularensis LVS was isolated. In 1960's and 1970's, F. tularensis LVS was used as vaccine for tularemia in United States governmental research institutes and the results showed good efficacy [2,3]. During this time, many experiments using mice model suggested F. tularensis LVS has good efficacy for prevention of tularemia [18]. However, F. tularensis LVS has a limit as tularemia vaccine. First, F. tularensis LVS cannot completely prevent pneumonic infection of type A bacteria. Second, the exact mechanism of attenuation in F. tularensis LVS is not fixed yet. These characters can raise concern about safety, the most important consideration for vaccine, and F. tularensis LVS is not approved by US Food and Drug Administration nowadays [12].
Recently, with great advance of genetic engineering technique, exact gene mapping or targeted mutagenesis are possible in F. tularensis biology. It allowed mutated F. tularensis with deletion of specific virulrence factor, and many tests using those bacteria as live vaccine have reported (Table 2). Swedish group and National Institutes of Health (NIH) group developed various mutant strains with deleted or changed genes for virulence factors on the FPI for live vaccine with higher safety which are not confirmed simultaneously conserving the high protection efficacy yet; Mainly Omp gene for capsule formation was deleted, or MglAB and PmrA that are responsible for transcriptional regulation during bacterial replication were mutated [1,12,19,20]. Canadian research group and another United States research group tried to develop new types of vaccine with mixing some of these attenuated strains. As a new candidate for live vaccine development, F. novicida was also tesed after mutated for antigen-mimicking. However, the trials with the mutants of F. novicida did not produce enough protection efficacies for the challenge using type A strain. Until now, LVS strains attenuated from F. tularensis subsp. holarctica is the only vaccine candidate eliciting strong vaccination results concerning type A strain [21].
Otherwise some tularemia scientists think the subspecies tularensis (experimentally confirmed type A strains such as Schu S4) rather than other subspecies should be the best candidate for live vaccine if safety can be guaranteed [22]. It was reported that the immune responses during LVS infection was much different from the responses to the infection of type A strains, especially about the responses against the respiratory infection by bacteria [23]. The difference may imply that the development of tularemia vaccine would be focused on the type A strains for vaccine materials instead of genetically different type B strains.
At the early time of subunit vaccine development, research was focused on the protective proteins or components of the bacteria surface, and lipopolysaccharides (LPS) is one of the major targets for subunit vaccine because F. tularensis is Gram-negative bacteria containing LPS on the outer membranes. Foshay's split vaccine trial also was continued for a while even though the results were not very clear [17]. While in vitro research on the cell level indicated increase of proteins activating T-cell-mediated immune responses were secreted by LPS injection into the animals or human, the split vaccine components boosting these immune responses did not generate enough protection efficacy on the whole organism level comparing to LVS injection [24] (Table 3). Other antigenic proteins in addition to the LPS did not show remarked efficacy against the type A strains while they induced somewhat protective capacity against lower pathogenic type B strains [25].
Outer membrane protein is another candidate for the development of subunit vaccine. FopA is major component of outer-membrane protein in F. tularensis, however the results from the efficacy test using the protein still did not get expected efficacy. Antigens such as GroEL, KatG, and Tul4 did not suggest any successful results of vaccination efficacies either, and the continuous failure of subunit vaccine trials from Foshay's split vaccine raises the assumption; it should be required that host system could recognize the multiple antigens to induce combined immune responses including cellular immunity. Based on this idea, combined vaccination of LVS and LPS is currently tested for the generation of higher efficacy [26].
Types of recombinant vaccine using subunits as immunogens can guarantee the safety comparing currently developed LVS, also they can maximize the immunogenicity mixing multiple antigens from different variants. F. tularensis is intracellular bacteria, and its pathogenesis raises both antibody-mediated and cell-mediated immunity. Not only antibody generation but also induction of T-cell response would be required to obtain sufficient vaccine efficacies. To induce the combined immune response, research considering cellular immunity is actively added to the traditional trials of vaccine development using antibody induction [24].
F. tularensis is one of the most dangerous pathogens having potential to generate the nationwide disaster through deliberate use because there are no experts in clinical fields who can rapidly find a way to diagnose or treat the infected patients due to very few cases of natural infection in Korea while the bacteria keep heavy pathological traits causing severe symptoms in infected human body. Furthermore the high fatality of these bacteria, leading victims to death with only 10 multiplicity of infection, can cause the embarrassing results from delayed treatment following improper diagnostics. Vaccination should be the most efficient and practical way to prepare the real situation of this bio-threat. However, there is no vaccine officially approved until now. Development of tularemia vaccine with undoubted safety and rapid protection efficacy should be an essential part of national preparedness even though LVS or LPS could be used for real dangerous situation in spite of the safety issues and less efficacy. Strategic support by government should be urgently required for the development and stockpile of reliable vaccine to reach this final destination of tularemia preparedness.
Figures and Tables
Fig. 1
Transmission of Francisella tularensis from wild animals and environments to human. Type A tularemia caused by F. tularensis subsp. tularensis is usually transmitted through the terrestrial route by vectors such as ticks and deer flies while type B tularemia raised by F. tularensis subsp. holarctica and other subspecies is transmitted through both routes of aquatic and terrestrial cycles.
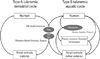
Fig. 2
Survival mechanism of Francisella tularensis in macrophage after infection. Infected bacteria is engulfed by endosome for phagocytic activity, however some bacteria developed the immune escaping capability to develop autophagy instead of phagocytosis. EEA, early endosome antigen; LAMP, lysosome-associated membrane protein.
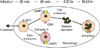
Table 2
Brief list of live attenuated vaccine candidates reported for partial protective efficacy (results from animal tests using mice) [1]
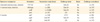
Table 3
Brief list of subunit vaccine cadidates reported for partial protective efficacy (results from animal tests using mice) [1]

References
1. Pechous RD, McCarthy TR, Zahrt TC. Working toward the future: insights into Francisella tularensis pathogenesis and vaccine development. Microbiol Mol Biol Rev. 2009. 73:684–711.


2. Saslaw S, Eigelsbach HT, Prior JA, Wilson HE, Carhart S. Tularemia vaccine study. II. Respiratory challenge. Arch Intern Med. 1961. 107:702–714.
3. Saslaw S, Eigelsbach HT, Wilson HE, Prior JA, Carhart S. Tularemia vaccine study. I. Intracutaneous challenge. Arch Intern Med. 1961. 107:689–701.
4. Dennis DT, Inglesby TV, Henderson DA, et al. Tularemia as a biological weapon: medical and public health management. JAMA. 2001. 285:2763–2773.
5. Keim P, Johansson A, Wagner DM. Molecular epidemiology, evolution, and ecology of Francisella. Ann N Y Acad Sci. 2007. 1105:30–66.


6. Pullen RL, Stuart BM. Tularemia: analysis of 225 cases. JAMA. 1945. 129:495–500.
7. Evans ME, Gregory DW, Schaffner W, McGee ZA. Tularemia: a 30-year experience with 88 cases. Medicine (Baltimore). 1985. 64:251–269.
8. Conlan JW, KuoLee R, Shen H, Webb A. Different host defences are required to protect mice from primary systemic vs pulmonary infection with the facultative intracellular bacterial pathogen, Francisella tularensis LVS. Microb Pathog. 2002. 32:127–134.


9. Hopla CE. The multiplication of tularemia organisms in the lone star tick. Am J Hyg. 1955. 61:371–380.
10. Checroun C, Wehrly TD, Fischer ER, Hayes SF, Celli J. Autophagy-mediated reentry of Francisella tularensis into the endocytic compartment after cytoplasmic replication. Proc Natl Acad Sci U S A. 2006. 103:14578–14583.


11. Clemens DL, Lee BY, Horwitz MA. Virulent and avirulent strains of Francisella tularensis prevent acidification and maturation of their phagosomes and escape into the cytoplasm in human macrophages. Infect Immun. 2004. 72:3204–3217.


13. World Health Organization. WHO/CDS/EPR/2007.7. WHO guidelines on tularaemia. 2007. Geneva: World Health Organization.
14. Kaufmann AF, Meltzer MI, Schmid GP. The economic impact of a bioterrorist attack: are prevention and postattack intervention programs justifiable? Emerg Infect Dis. 1997. 3:83–94.


15. Tigertt WD. Soviet viable Pasteurella tularensis vaccines: a review of selected articles. Bacteriol Rev. 1962. 26:354–373.


16. Christopher GW, Cieslak TJ, Pavlin JA, Eitzen EM Jr. Biological warfare: a historical perspective. JAMA. 1997. 278:412–417.


17. Foshay L, Hesselbrock WH, Wittenberg HJ, Rodenberg AH. Vaccine prophylaxis against tularemia in man. Am J Public Health Nations Health. 1942. 32:1131–1145.


18. Centers for Disease Control and Prevention. Summary of notifiable diseases, United States, 1997. MMWR Morb Mortal Wkly Rep. 1998. 46:ii–vii. 3–87.
19. Gray CG, Cowley SC, Cheung KK, Nano FE. The identification of five genetic loci of Francisella novicida associated with intracellular growth. FEMS Microbiol Lett. 2002. 215:53–56.


20. Nano FE, Zhang N, Cowley SC, et al. A Francisella tularensis pathogenicity island required for intramacrophage growth. J Bacteriol. 2004. 186:6430–6436.


21. Shen H, Chen W, Conlan JW. Mice sublethally infected with Francisella novicida U112 develop only marginal protective immunity against systemic or aerosol challenge with virulent type A or B strains of F. tularensis. Microb Pathog. 2004. 37:107–110.


22. Wu TH, Hutt JA, Garrison KA, Berliba LS, Zhou Y, Lyons CR. Intranasal vaccination induces protective immunity against intranasal infection with virulent Francisella tularensis biovar A. Infect Immun. 2005. 73:2644–2654.


23. Hall JD, Woolard MD, Gunn BM, et al. Infected-host-cell repertoire and cellular response in the lung following inhalation of Francisella tularensis Schu S4, LVS, or U112. Infect Immun. 2008. 76:5843–5852.


24. Golovliov I, Ericsson M, Akerblom L, Sandstrom G, Tarnvik A, Sjostedt A. Adjuvanticity of ISCOMs incorporating a T cell-reactive lipoprotein of the facultative intracellular pathogen Francisella tularensis. Vaccine. 1995. 13:261–267.

