Abstract
Low pathogenic avian influenza (LPAI) H9N2 viruses have been circulating in the Eurasian poultry industry resulting in great economic losses due to declined egg production and moderate to high mortality. In Korea, H9N2 LPAI was first documented in 1996 and it caused serious economic loss in the Korean poultry industry, including layer and broiler breeder farms. Since then, the H9N2 viruses that belong to the Korea group have been prevalent in chickens and have continuously evolved through reassortment in live bird markets. To control LPAI outbreaks, since 2007, the Korean veterinary authority has permitted the use of the inactivated oil adjuvant H9N2 LPAI vaccine. Although only oil-based inactivated vaccine using the egg-passaged vaccine virus strain (A/chicken/Korea/01310/2001) is permitted and used, several new technology vaccines have been recently suggested for the development of cost-effective and highly immunogenic vaccines. In addition, several different differentiation of infected from vaccinated animals (DIVA) strategies have been suggested using appropriate vaccines and companion serologic tests for discriminating between naturally infected and vaccinated animals. Recent reports demonstrated that the Korean LPAI H9N2 virus underwent antigenic drift and evolved into distinct antigenic groups and thus could escape from vaccine protection. Therefore, improved vaccination strategies including periodic updates of vaccine seed strains are required to achieve efficient control and eradication of LPAI H9N2 in Korea. Further, vaccination should be part of an overall integrated strategy to control the disease, including continued nation-wide surveillance, farm biosecurity, and DIVA strategy.
Avian influenza virus (AIV) belongs to the influenza virus A genus of the Orthomyxoviridae family [1]. The AIV genome consists of eight segments of negative-sense, single-stranded RNA that encode at least ten proteins including two surface glycoproteins (haemagglutinin [HA] and neuraminidase [NA]), nucleoprotein (NP), three polymerase proteins (polymerase basic [PB] 2, PB1 and polymerase acidic), two matrix (M1 and M2) proteins, and two non-structural (NS1 and NS2) proteins. AIV is classified into subtypes base on the antigenic differences between their two surface glycoproteins, HA and NA. Sixteen HA subtypes (H1 to H16) and nine NA subtypes (N1 to N9) have been identified among influenza A viruses, and viruses of all subtypes and of the majority of possible combinations have been isolated from avian species.
AIV infection can cause a range of disease symptoms from asymptomatic infection to respiratory disease and drops in egg production to severe, systemic disease with near 100% mortality rates. Genetic features and/or severity of disease in poultry determine whether the virus is classified as low pathogenic avian influenza (LPAI) or highly pathogenic avian influenza (HPAI). LPAI includes viruses in all H1 to H16 subtypes. On the other hand, HPAI has traditionally been either H5 or H7 subtypes [2]. The HA gene is a key determinant of virulence in poultry species. The HA gene of HPAI have multiple basic amino acids at the cleavage site that is cleavable by Furin-like cellular enzymes, leading to systemic infection and mortality [3]. Since 2005, the widespread of HPAI H5N1 has caused infection to wild waterfowls and domestic poultry in Central and South Asia, Middle East, Europe, and Africa [4]. These unprecedented outbreaks of HPAI H5N1 and zoonotic infections threatened not only the poultry industry but also public health.
Among the many subtypes, the subtype H9N2 is thought to rapidly spread and become one of the most prevalent LPAI viruses in the domestic poultry industry. For LPAI H9N2 virus, detailed antigenic and molecular analysis have identified several groups of H9N2 influenza virus in avian species in Eurasia: the G1 lineage, represented by A/quail/Hong Kong/G1/97 (G1-like); the Y280 lineage, represented by three prototype viruses A/duck/Hong Kong/Y280/97 (Y280-like), A/chicken/Beijing/1/94 (BJ94-like), and A/chicken/Hong Kong/G9/97 (G9-like) and the Korean lineage, represented by A/chicken/Korea/38349-p96323/96 (Korean-like) and A/duck/Hong Kong/Y439/97 (Y439-like) [5-7]. LPAI H9N2 viruses have been circulating in multiple avian species in Eurasia resulting in great economic losses in the poultry industry due to declined egg production or moderate to high mortality [8-12]. Furthermore, the H9N2 LPAI virus is known to possess human-like receptor specificity [5] and transmissibility to mammalian species including humans, is raising public health concerns [13,14].
To control HPAI outbreaks, several strategies have been applied including a high level of biosafety, movement control, stamping out, and vaccination. Although vaccination in poultry species against HPAI is still a controversial topic and has been discouraged in the past, vaccination has also been recommended as one of the HPAI control strategies [15]. For HPAI, once an outbreak has been identified, eradication measures based on the stamping-out and restriction measures on the movement of live poultry, vehicles and staff should be enforced. However, when an outbreak of HPAI occurs in an area with a high poultry density in which the application of biosecurity measures is not compatible with the modern poultry rearing systems, vaccination should be considered as a first option to control the spread of infection [16]. On the other hand, for LPAI H9N2, due to the widespread of the H9N2 viruses and the zoonotic potential of the virus, an oil-based inactivated H9N2 LPAI vaccine was employed in the chicken industry for preventing H9N2 infection in several countries [12,17,18].
This review aims to elucidate crucial issues regarding the evolution of the Korean H9N2 virus and its vaccine strategy in the poultry industry. In addition, recent advances and challenges of vaccination against LPAI H9N2 in Korea are discussed.
The first field outbreak of the H9N2 virus in Korea was caused by A/chicken/Korea/96006/96 (H9N2), a virus genetically closely related to the A/duck/Hong Kong/Y439/97 (Korea group) virus later isolated from aquatic birds. Since then, H9N2 LPAI viruses have been endemic in domestic poultry farms in Korea and have often caused slight to moderate mortality (5-30%) with apparent clinical signs that is characterized by depression, edema of head, cyanosis of comb and legs, and drop in egg production [19,20].
The role of live bird market (LBM) is very important in the ecology of Korean H9N2 AIVs (Fig. 1). The H9N2 viruses that belong to the Korea group have continuously evolved by antigenic drift and have undergone reassortment with aquatic AIV isolates circulating in the Korean LBMs [9,21-26]. LBMs bring together a number of hosts in a high-density setting, providing an ideal environment for interspecies transmission and viral evolution [27]. In Korea, traditional livestock production is still preserved on small scale farms, in which some minor poultry species, such as quail, pheasants, silky fowls and Korean native chickens are raised. These minor poultry species are generally reared in poorly managed farms and are mainly retailed via LBMs. In addition, traditional markets such as permanent markets and 5-day markets began to appear toward the end of the 15th century and are still being held today. In the marketplaces, LBMs offer a variety of avian species, including chickens, quails, pheasants, turkeys, domestics ducks, mallard ducks, Muscovy ducks, and pigeons [24].
In 2004, there was a report of reassortant H9N8 virus containing genes from both Eurasian and North American phylogenetic lineages. Kwon et al. [28] reported that a LPAI outbreak caused by H9N8 was first detected in chickens at Korean native chicken farms. This isolate caused moderate respiratory distress, depression, mild diarrhea, loss of appetite and a slightly elevated mortality (1.4% in 5 days). Phylogenetic analysis of the surface genes of the H9N8 isolate showed that the HA gene was related to that of Korean H9N2 AIVs, but the NA gene was closely related to an unknown aquatic AIV isolate in Korea. Later, the NA gene of this H9N8 isolate was found to be from North American phylogenetic lineages [29].
In addition to biosecurity measures, the vaccination practice is an important tool for prevention and control of AIVs. Since vaccination against AIV was not allowed in Korea, the Korean veterinary authorities took governmental stamping-out and compensation policies between the 1996 and 1999 H9N2 LPAI outbreaks. In order to control the LPAI endemic situation, since 2007, the Korean veterinary authority has permitted the use of the oil-based inactivated H9N2 LPAI vaccine. A vaccine strain virus that had been isolated in 2001 (A/chicken/Korea/01310/2001) was selected based on its pathogenic, antigenic, and genetic properties. In a chicken experiment, a single administration of the oil-based inactivated vaccine using the 20th passage of the vaccine virus strain (A/chicken/Korea/01310/2001) showed efficient protection against a homologous virus and Korean H9N2 virus isolated in 2004 [30]. Oil-based inactivated H9N2 LPAI vaccines using this vaccine strain have been produced by 5 Korean vaccine companies and used in Korean broiler breeder and layer chicken farms.
Vaccination against AIV, an effective control measure for the eradication of LPAI or HPAI, may result in issues related to the international trade of poultry products and AIV surveillance programs. Therefore, several different DIVA strategies have been suggested using appropriate vaccines and companion serologic tests for discriminating between naturally infected and vaccinated animals. These strategies include: the use of sentinels, the use of subunit vaccines, heterologous neuraminidase strategy, and the NS1 strategies [31]. In Korea, several studies for DIVA strategies have been suggested (Table 1). These strategies include: the H5N3 heterologous neuraminidase strategy for HPAI H5N1 [32], the use of H9 virus-like particle (VLP) vaccine for LPAI H9N2 [33], the reassortant H9N8 (rgH9N8) vaccine and the companion N2-specific enzyme-linked immunosorbent assay (ELISA) for LPAI H9N2 [34], and the M2e ELISA for LPAI H9N2 [35].
For the heterologous neuraminidase strategy, Lee et al. [32], suggested the heterologous neuraminidase H5N3 vaccine for the control of H5N1 HPAI. The vaccine strain was derived from the LPAI H5N3 virus isolated from wild bird feces. This vaccine allowed DIVA by indirect immunofluorescent antibody (IFA) test. In addition, two doses of 64 HA unit of heterologous H5N3 inactivated vaccine as well as one dose of 1,024 HA unit of heterologous H5N3 inactivated vaccine induced 100% protective efficacy against lethal HPAI H5N1 infection and could prevent viral shedding completely.
For the H9 VLP vaccine, since this VLP vaccine contains only HA and M1 proteins, it allows differentiation of AIV-infected chickens from vaccinated chickens with a commercial ELISA using the NP antigen [33]. In animal studies, a single dose of H9 HA VLP vaccine induced high levels of haemagglutination inhibition (HI) antibodies and lowered frequencies of virus isolation after the wild-type virus challenge.
For the rgH9N8 vaccine and companion N2-ELISA, Kwon et al. [34] suggested a N2-ELISA for a high throughput screening test as an alternative method to an indirect IFA method. This strategy used reverse genetics derived heterologous neuraminidase vaccine containing H9 and N8 proteins and companion N2-ELISA. The sera of uninfected chickens and those vaccinated with rgH9N8 vaccine were negative by the N2-ELISA, whereas infected sera with H9N2 were positive.
For M2e ELISA, Kim et al. [35] developed an ELISA using the extracellular domain of the Korean H9N2 M2 peptides. This strategy is based on differential epitope density of M2e peptides on the surfaces of infected cells and on infectious viral particles. In field applications of M2e ELISA, anti-M2e antibody produced in infected chickens after vaccination or in re-infected chickens could be identified as AIV-infected chickens, although HI test could not distinguish infected from vaccinated chickens.
By virtue of greatly advanced molecular virology and the availability of a great deal of genomic information on AIV, there are new opportunities to create novel concept vaccines. In order to be a cost-effective and highly immunogenic vaccine, a seed virus strain should have high growth characteristics in eggs or cells and proper antigenicity with regard to wild type viruses. Occasionally, the prototype vaccine strains do not replicate well in eggs. If a seed virus strain cannot grow well in eggs, it would require more egg quantity and high cost processing to concentrate. Therefore, to increase a yield of vaccine strain, multiple passages in embryonated eggs were performed in previous studies. For example, Choi et al. [30] developed a high-yielding H9N2 vaccine seed strain, through the 20th passage of the vaccine virus strain in embryonated chicken eggs.
Recently, using plasmid-based reverse genetics (RG) to improve the ability of vaccine strains to replicate in eggs has been widely used in influenza vaccine studies [36,37]. The RG allows rapid generation of a desired recombinant vaccine seed strain from cloned cDNAs through simple DNA transfection protocols. Using the RG, a reassortant vaccine seed strain can be rapidly generated by co-transfection of cDNA mixture consisting of the six internal genes of a high-growth donor strain and two surface genes from a wild type virus, as an alternative to the classical multiple passage procedure using embryonated chicken eggs, allowing the conversion of a wild type virus into a genetically homologous AIV vaccine strain. For the H9N2 LPAI vaccine, Song et al. [38] developed a high-growth H9N2 vaccine strain using the RG system. The reassortant vaccine strains (H9N2/PR8) contains the internal genes of the high-yielding PR8 strain and the surface gene of the current Korean vaccine seed strain (A/chicken/Korea/01310/01). The reassortant viruses replicated in eggs more efficiently than the wild-type viruses. Similar to the A/chicken/Korea/01310/01 strain, the H9N2/PR8 strain could be propagated well in eggs and provided high antibody titers in vaccinated chickens and protective efficacy against homologous H9N2 virus infection. Based on its growth properties and immunogenicity, a reassortant vaccine strain is suggested to be an alternative vaccine candidate to current vaccine strains made by multiple passages.
VLP has been suggested as a new generation of non-egg-based vaccine platform against various viral infections with solid safety profiles [39]. VLP resembles infectious virus particles in structure and morphology with multiple antigenic epitopes which were shown to be highly immunogenic probably due to its ability to stimulate a diverse set of host immune responses [40]. VLP vaccines containing influenza HA and/or NA antigens are produced easily in insect or mammalian cells via the simultaneous expression of HA protein and NA protein along with a viral core protein, such as influenza M1 protein. Most influenza VLPs have been produced in baculovirus expression systems, and the safety and immunogenicity have been evaluated in various animal models [41-44]. Particularly, the H5N3 AIV VLP vaccine was applied to duck species and provided the possibility of reliable use of the VLP vaccine in poultry species [44,45]. For the LPAI H9N2 vaccine, the VLP vaccine against Korean H9N2 LPAI is developed and evaluated in an specific pathogen-free (SPF) chicken model [33]. This H9 VLP contains the HA and M1 protein of the current Korean vaccine seed strain (A/chicken/Korea/01310/01). VLP vaccine was prepared by emulsifying the purified VLP antigen solution with Montanide ISA70 (Seppic, Paris, France) oil adjuvant. A single dose of vaccination with H9 VLP vaccine induced high antibody titers and lessened the number of chickens with viral shedding from respiratory and gastrointestinal tracts. In addition, it allows differentiation of AIV-infected chickens from vaccinated chickens with an ELISA using nucleocapsid antigen, which offers a promising strategy to DIVA. However, vaccine cost has been thought to be an important issue that influences the feasibility of recombinant subunit vaccines including VLP for poultry. As performed by other VLP studies, H9 VLP antigen was purified by sucrose density gradient purification requiring expensive equipment and time-consuming process, which would potentially increase the vaccine production cost. Recently, Park et al. [43] developed H5N1 VLP antigen without further expensive and time-consuming purification except for low centrifugation to remove large cell debris. It was evident that the H5N1 VLP vaccine without the purification process used in this study costs less to produce compared with highly purified VLP vaccines reported in previous studies. Although H5N1 VLP antigen was prepared without purification, VLP-vaccinated chickens showed high levels of antibody titers without any signs or symptoms attributable to crude VLP antigen. These results provided promising methods for decreasing the production cost of VLP vaccines in poultry use.
Although a single administration of oil-based inactivated H9N2 LPAI vaccine is very immunogenic and highly protective in laboratory trials using SPF chickens, use of that vaccine in broiler breeder farms induced poor antibody response [46]. In general, it should be emphasized that although experimental studies may show good immunogenicity after vaccination, in the complexity of the field, vaccine use and immunogenicity will not reach maximum potential. Difference in environmental conditions and species of chickens used in the laboratory or farm trials might influence the immune response. In particular, the stress associated with the farm conditions and the heavy weight of broiler breeders are considered as causes of the decrease of the antibody response [47]. Therefore, improved vaccination strategy is required to increase the immunogenicity in chicken farms. These strategies could include: the use of a highly immunogenic vaccine seed strain, the improvement of an effective adjuvant for chickens, and the consideration of a 2-dose regimen. For example, a gel adjuvant-primed and mineral oil adjuvant-boosted regimen was suggested for improvement of immune responses to H9N2 LPAI in broiler breeder farms [46]. In the broiler breeder farm study, a 2-dose regimen of inactivated H9N2 LPAI vaccine enhanced the antibody response. Interestingly, the gel-primed and oil-boosted regimen produced slower antibody response after the first vaccination but showed higher antibody response than the oil-primed and oil-boosted regimen in a 44-week long-term study.
For the optimal protection efficiency, the antigenicity of the vaccine strain should match that of the prevailing AIV. Immune pressure would select only escaping mutants which possess alteration of key amino acids within antigenic sites. Immunity provoked by vaccination with the previous strain is unable to neutralize the escaped AIV mutants. Compared to human influenza virus, the antigenicity of AIV is relatively stable, which may be due to the lack of immune pressure. However, as large-scale and long-term vaccinations against AIV have been performed in several countries, AIVs have also undergone antigenic drift due to the presence of immune pressure [48]. After mass vaccination against LPAI H9N2 in Korea, virus isolation was limited to LBMs or poultry slaughter houses, indicating that LPAI H9N2 is under the control of vaccination. However, previous studies demonstrated that the Korean LPAI H9N2 virus underwent antigenic drift and evolved into distinct antigenic groups and thus could escape from vaccine protection [49,50]. Moreover, Park et al. [49] suggested that H9N2 viruses from domestic poultry have undergone substantial evolution after mass vaccination against LPAI H9N2 in Korea by immune selection as a result of vaccinal and natural immunity, coupled with reassortment. Also, several studies reported that the current Korean LPAI H9N2 vaccine strain (A/chicken/Korea/01310/01) could provide only partial protection against recent Korean LPAI H9N2 viruses isolated after 2004 [38,49,51]. Therefore, selection of more suitable vaccine strains, based on continued nation-wide surveillance data, is needed to prevent an LPAI H9N2 endemic in Korea.
Wide use of AIV vaccine in animal population could enhance the immune pressure and drive the mutation resulting in rapid antigenic drift at the antigenic sites [52]. Therefore, improved vaccination strategies and periodic updates of vaccine seed strains are required to increase immunogenicity and cross protective efficacy in chicken farms. These strategies could include: the selection of highly immunogenic vaccine seed strains, the use of effective adjuvants for chickens, and the use of new technology vaccines. Several studies reported that the recent Korean LPAI H9N2 virus underwent antigenic drift and could escape from vaccine protection. Thus, continued active surveillance of poultry farms and LBMs to reveal new variant LPAI H9N2 viruses in Korea and analyzing appropriate vaccine seed viruses should be considered to prevent new outbreaks.
In Korea, although only oil-based inactivated vaccines using the multiple passage of the vaccine virus strain (A/chicken/Korea/01310/2001) is used, several new technology vaccines have been recently suggested for development of cost-effective and highly immunogenic vaccines. In particular, genetically engineered vaccines showed promise as the next generation of AI vaccines in Korea. These new vaccines, as well as use of effective adjuvants, will certainly contribute to a better protection against LPAI H9N2 in the Korean poultry industry.
In order to achieve efficient control and eradication of LPAI H9N2 in Korea, vaccination should be part of an overall integrated strategy to control the disease, including continued nation-wide surveillance, farm biosecurity, and DIVA strategy. Eradication of LPAI H9N2 in Korea can only be achieved if all aspects of the control strategy are effectively operational.
Figures and Tables
Fig. 1
Role of live bird markets in facilitating the evolution of low pathogenic avian influenza H9N2 virus in Korea. The H9N2 viruses that belong to the Korea group have continuously evolved by antigenic drift and have undergone reassortment with avian influenza virus genes from wild bird isolates, poultry farm isolates, and minor poultry isolates in the Korean live bird markets.
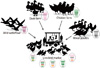
References
1. Webster RG, Bean WJ, Gorman OT, Chambers TM, Kawaoka Y. Evolution and ecology of influenza A viruses. Microbiol Rev. 1992. 56:152–179.


4. Munster VJ, Fouchier RA. Avian influenza virus: of virus and bird ecology. Vaccine. 2009. 27:6340–6344.


5. Matrosovich MN, Krauss S, Webster RG. H9N2 influenza A viruses from poultry in Asia have human virus-like receptor specificity. Virology. 2001. 281:156–162.


6. Butt AM, Siddique S, Idrees M, Tong Y. Avian influenza A (H9N2): computational molecular analysis and phylogenetic characterization of viral surface proteins isolated between 1997 and 2009 from the human population. Virol J. 2010. 7:319.


7. Guan Y, Shortridge KF, Krauss S, Webster RG. Molecular characterization of H9N2 influenza viruses: were they the donors of the "internal" genes of H5N1 viruses in Hong Kong? Proc Natl Acad Sci U S A. 1999. 96:9363–9367.


8. Guo YJ, Krauss S, Senne DA, et al. Characterization of the pathogenicity of members of the newly established H9N2 influenza virus lineages in Asia. Virology. 2000. 267:279–288.


9. Lee YJ, Shin JY, Song MS, et al. Continuing evolution of H9 influenza viruses in Korean poultry. Virology. 2007. 359:313–323.


10. Monne I, Cattoli G, Mazzacan E, et al. Genetic comparison of H9N2 AI viruses isolated in Jordan in 2003. Avian Dis. 2007. 51:1 Suppl. 451–454.


11. Toroghi R, Momayez R. Biological and molecular characterization of Avian influenza virus (H9N2) isolates from Iran. Acta Virol. 2006. 50:163–168.
12. Wu R, Sui ZW, Zhang HB, et al. Characterization of a pathogenic H9N2 influenza A virus isolated from central China in 2007. Arch Virol. 2008. 153:1549–1555.


13. Butt KM, Smith GJ, Chen H, et al. Human infection with an avian H9N2 influenza A virus in Hong Kong in 2003. J Clin Microbiol. 2005. 43:5760–5767.


14. Lin YP, Shaw M, Gregory V, et al. Avian-to-human transmission of H9N2 subtype influenza A viruses: relationship between H9N2 and H5N1 human isolates. Proc Natl Acad Sci U S A. 2000. 97:9654–9658.


15. Butler D. Vaccination will work better than culling, say bird flu experts. Nature. 2005. 434:810.


16. Capua I, Marangon S. The use of vaccination as an option for the control of avian influenza. Avian Pathol. 2003. 32:335–343.


17. Naeem K, Siddique N, Ayaz M, Jalalee MA. Avian influenza in Pakistan: outbreaks of low- and high-pathogenicity avian influenza in Pakistan during 2003-2006. Avian Dis. 2007. 51:1 Suppl. 189–193.


18. Perk S, Panshin A, Shihmanter E, et al. Ecology and molecular epidemiology of H9N2 avian influenza viruses isolated in Israel during 2000-2004 epizootic. Dev Biol (Basel). 2006. 124:201–209.
19. Lee CW, Song CS, Lee YJ, et al. Sequence analysis of the hemagglutinin gene of H9N2 Korean avian influenza viruses and assessment of the pathogenic potential of isolate MS96. Avian Dis. 2000. 44:527–535.


20. Kwon HJ, Cho SH, Kim MC, Ahn YJ, Kim SJ. Molecular epizootiology of recurrent low pathogenic avian influenza by H9N2 subtype virus in Korea. Avian Pathol. 2006. 35:309–315.


21. Choi YK, Seo SH, Kim JA, Webby RJ, Webster RG. Avian influenza viruses in Korean live poultry markets and their pathogenic potential. Virology. 2005. 332:529–537.


22. Kim HR, Park CK, Oem JK, et al. Characterization of H5N2 influenza viruses isolated in South Korea and their influence on the emergence of a novel H9N2 influenza virus. J Gen Virol. 2010. 91(Pt 8):1978–1983.


23. Kim JA, Cho SH, Kim HS, Seo SH. H9N2 influenza viruses isolated from poultry in Korean live bird markets continuously evolve and cause the severe clinical signs in layers. Vet Microbiol. 2006. 118:169–176.


24. Lee HJ, Kwon JS, Lee DH, et al. Continuing evolution and interspecies transmission of influenza viruses in live bird markets in Korea. Avian Dis. 2010. 54:1 Suppl. 738–748.


25. Lee HJ, Lee DH, Lee YN, et al. Generation of reassortant influenza viruses within the non-industrial poultry system. Infect Genet Evol. 2012. 12:933–946.


26. Moon HJ, Song MS, Cruz DJ, et al. Active reassortment of H9 influenza viruses between wild birds and live-poultry markets in Korea. Arch Virol. 2010. 155:229–241.


27. Liu M, He S, Walker D, et al. The influenza virus gene pool in a poultry market in South central china. Virology. 2003. 305:267–275.


28. Kwon YK, Lee YJ, Choi JG, et al. An outbreak of avian influenza subtype H9N8 among chickens in South Korea. Avian Pathol. 2006. 35:443–447.


29. Lee DH, Lee HJ, Lee YN, et al. Evidence of intercontinental transfer of North American lineage avian influenza virus into Korea. Infect Genet Evol. 2011. 11:232–236.


30. Choi JG, Lee YJ, Kim YJ, et al. An inactivated vaccine to control the current H9N2 low pathogenic avian influenza in Korea. J Vet Sci. 2008. 9:67–74.


32. Lee YJ, Sung HW, Choi JG, et al. Effects of homologous and heterologous neuraminidase vaccines in chickens against H5N1 highly pathogenic avian influenza. Avian Dis. 2007. 51:1 Suppl. 476–478.


33. Lee DH, Park JK, Lee YN, et al. H9N2 avian influenza virus-like particle vaccine provides protective immunity and a strategy for the differentiation of infected from vaccinated animals. Vaccine. 2011. 29:4003–4007.


34. Kwon JS, Kim MC, Jeong OM, et al. Novel use of a N2-specific enzyme-linked immunosorbent assay for differentiation of infected from vaccinated animals (DIVA)-based identification of avian influenza. Vaccine. 2009. 27:3189–3194.


35. Kim MC, Choi JG, Kwon JS, et al. Field application of the H9M2e enzyme-linked immunosorbent assay for differentiation of H9N2 avian influenza virus-infected chickens from vaccinated chickens. Clin Vaccine Immunol. 2010. 17:1977–1984.


36. Engelhardt OG. Many ways to make an influenza virus: review of influenza virus reverse genetics methods. Influenza Other Respi Viruses. 2012. [Epub]. http://dx.doi.org/10.1111/j.1750-2659.2012.00392.x.
37. Neumann G, Ozawa M, Kawaoka Y. Reverse genetics of influenza viruses. Methods Mol Biol. 2012. 865:193–206.


38. Song JM, Lee YJ, Jeong OM, et al. Generation and evaluation of reassortant influenza vaccines made by reverse genetics for H9N2 avian influenza in Korea. Vet Microbiol. 2008. 130:268–276.


39. Roldao A, Mellado MC, Castilho LR, Carrondo MJ, Alves PM. Virus-like particles in vaccine development. Expert Rev Vaccines. 2010. 9:1149–1176.


40. Kang SM, Compans RW. Host responses from innate to adaptive immunity after vaccination: molecular and cellular events. Mol Cells. 2009. 27:5–14.


41. Kang SM, Song JM, Quan FS, Compans RW. Influenza vaccines based on virus-like particles. Virus Res. 2009. 143:140–146.


42. Pushko P, Kort T, Nathan M, Pearce MB, Smith G, Tumpey TM. Recombinant H1N1 virus-like particle vaccine elicits protective immunity in ferrets against the 2009 pandemic H1N1 influenza virus. Vaccine. 2010. 28:4771–4776.


43. Park JK, Lee DH, Youn HN, et al. Protective efficacy of crude virus-like particle vaccine against HPAI H5N1 in chickens and its application on DIVA strategy. Influenza Other Respi Viruses. 2012. [Epub]. http://dx.doi.org/10.1111/j.1750-2659.2012.00396.x.


44. Prel A, Le Gall-Recule G, Jestin V. Achievement of avian influenza virus-like particles that could be used as a subunit vaccine against low-pathogenic avian influenza strains in ducks. Avian Pathol. 2008. 37:513–520.


45. Prel A, Le Gall-Recule G, Cherbonnel M, Grasland B, Amelot M, Jestin V. Assessment of the protection afforded by triple baculovirus recombinant coexpressing H5, N3, M1 proteins against a homologous H5N3 low-pathogenicity avian influenza virus challenge in Muscovy ducks. Avian Dis. 2007. 51:1 Suppl. 484–489.


46. Lee DH, Kwon JS, Lee HJ, et al. Inactivated H9N2 avian influenza virus vaccine with gel-primed and mineral oil-boosted regimen could produce improved immune response in broiler breeders. Poult Sci. 2011. 90:1020–1022.


48. Sun Y, Pu J, Fan L, et al. Evaluation of the protective efficacy of a commercial vaccine against different antigenic groups of H9N2 influenza viruses in chickens. Vet Microbiol. 2012. 156:193–199.


49. Park KJ, Kwon HI, Song MS, et al. Rapid evolution of low-pathogenic H9N2 avian influenza viruses following poultry vaccination programmes. J Gen Virol. 2011. 92(Pt 1):36–50.


50. Lee CH, Byun SH, Lee YJ, Mo IP. Genetic evolution of the H9N2 avian influenza virus in Korean poultry farms. Virus Genes. 2012. 45:38–47.

