Abstract
Objective
This study describes a method for inducing spinal cord injuries in dogs by using balloon catheters via laminectomy and the subsequent changes in the electrophysiological response.
Methods
Female Beagle (Orient Bio, Seongnam, Korea) dogs weighing 10 kg at the time of injury were used. Under inhalation anesthesia, a posterior midline approach laminectomy was performed. A silicone balloon catheter (size 6 Fr; Sewoon Medical, Cheonan, Korea) was then inserted into the vertebral canal at the center of T10. The balloon was inflated to the maximum volume for 1, 2, or 3 days. Open field testing was performed for evaluating motor functions of the hindlimbs. Motor evoked potentials (MEPs) induced by electrical and magnetic stimulation were recorded before and after spinal cord injury.
Results
Open field testing yielded locomotor scores of 0 or 1 for dogs subjected to compression for 3 days. These dogs showed no obvious improvement throughout the observation period, and the tonus of their hindlimbs was flaccid. In contrast, motor functions of dogs that had experienced compression for 1 or 2 days were variable, and all dogs showed spastic tonus in their hindlimbs. In dogs subjected to after compression for 3 days, electrically stimulated MEPs for the hindlimbs showed a significant amplitude reduction. Further, hindlimb movements were not evoked by magnetic stimulation of the cervical spine and vertex area.
Severe spinal cord injury (SCI), which leads to complete loss of sensory and motor functions, is one of the most serious neurological problems.6,9,12) Although it has been believed that the damaged central nervous system (CNS) cannot regenerate after injury, there is an emerging hope for regeneration-based therapies for the damaged CNS due to the progress in developmental biology and regenerative medicine, including stem cell biology.9,12,16) However, in studies aiming to assess the effects of treatments, such as antioxidant, steroid, and stem cell therapies, it is important to identify whether the chosen animal model is a reliable model of SCI in humans.
Dogs may be suitable for such studies, since dogs not only have very similar clinical signs and equally poor prognosis as their human counterparts, but also serve as a good intermediate biological model, bridging the large gap between human and rat studies.7) Unfortunately, the procedure for creating a canine model of spinal SCI and for electrophysiological assessments in these models has not been described in detail in the literature. In this study, we focused on describing a method for inducing SCI and performing electrophysiological assessments in a canine model; we used a balloon catheter via laminectomy for inducing the injury.
Animal experiments were carried out in accordance with established practices as described in the National Institutes of Health Guide for Care and Use of Laboratory Animals and approved by the Institutional Animal Care and Use Committee of the Asan Institute for Life Sciences, Seoul, Korea. Female Beagle (Orient bio, Seongnam, Korea) dogs weighing 8 kg to 12 kg at the time of injury were used. Before injury, all dogs were housed one per cage under simulated daylight conditions with alternating 12-hour light-dark cycles and had free access to food and water.
The dogs were anesthetized using an intramuscular injection of Zoletil (25 mg/kg) and Rompun (5 mg/kg) together with atropine sulfate (0.25 mg/dog). Anesthesia was maintained by inhalation of 70% N2O and 1% isoflurane (Hana Pharm Co. Ltd., Hwaseong, Korea) through an endotracheal tube. The isoflurane concentration was increased to 2% during induction of the SCI. Dogs were laid in ventral recumbence on the operating bed with their limbs immobilized and pulled caudally. The posterior midline approach was selected. Usual muscle dissection was performed using electrocautery, and laminectomy was performed using an electrical drill. We identified the spinal cord between the L1 and L2 spinous processes of the vertebrae; a silicone balloon catheter (size 6 Fr; Sewoon Medical Co. Ltd., Cheonan, Korea) was then inserted into the vertebral canal. The balloon catheter was inserted in the cranial direction, to a distance corresponding to that previously measured between T10 and L1, thereby positioning it for inflation at the center of T10. The balloon was then inflated to the maximum volume (1.5 mL, via saline injection) in the spinal extradural space for 1, 2, or 3 days. After surgery, the skin was closed, and the dogs were administered a prophylactic intramuscular injection of cefazolin (20 mg/kg). For pain management, the animals intramuscularly injected with ketoprofen (10 mg/kg) once daily for 1 week. After injury, all dogs were housed individually in the same pre-injury environment. Their bladders were emptied manually twice daily until spontaneous control of urination returned.
Hindlimb motor function was assessed after 1, 2, or 3 days of compression SCI. The open field test was performed for all dogs as previously described.1,2,4) Before this test, each animal was allowed to adapt to an open field for 5 min. Once a dog demonstrated acclimatization on the open field, locomotor performance was evaluated for 5 min. We evaluated hindlimb motor function every 2 weeks from 1 day to 3 months after surgery.
Under inhalation anesthesia, spinal cord motor evoked potential (MEP) in response to electrical or magnetic stimulation (spinal-MEP) was recorded from the forelimbs and hindlimbs of dogs subjected to compression for 3 days using a Medelec Synergy system (Oxford Instrument Medical Inc., Surrey, UK). First, an electrical stimulus was delivered through two needle-type electrodes placed on the vertex, with an intensity of 100 mA, pulse duration of 0.5 ms, and pulse rate of 4.0 Hz. The recorded potentials were amplified and averaged over 100 impulses/recording. Second, a magnetic stimulation system (Magstim M200®; Magstim, Whiteland, UK) was used to analyze motor function. This apparatus provided a brief magnetic pulse that stimulated the spinal cord. Clinical and experimental applications of this apparatus have been reported elsewhere.3,5,15) An operational setting of 80% of the maximum output power was used, and the duration of the stimulus was 0.01 ms. Stimuli were delivered using an 8-figure coil (double 70-mm coil) applied to both the vertex and cervical spine, with identical tests performed on both injured and control dogs. Following stimulation of the spinal cord, Medelec Synergy® (Oxford Instrument Medical Inc., Surrey, UK) was used to record compound muscle action potentials (CMAP) with a needle electrode placed on the left triceps surae and tibia. The average of 10 recordings was calculated, and CMAP curves were obtained. A reduction in amplitude to less than 50% of the amplitude for controls was considered to represent critical SCI.
The Basso, Beattie, and Bresnahan (BBB) locomotor rating scale scores for dogs subjected to compression for 3 days remained at 0 or 1, without any obvious improvement, throughout the observation period. The tonus of their hindlimbs remained flaccid. These dogs moved using only their forelimbs, with no joint contracture evident in the hindlimbs. The hindlimbs were dragged with the dorsal surface of the paws against the floor. No voluntary hindlimb movement was observed. In contrast, motor function in dogs that had experienced compression for only 1 or 2 days was variable; all these dogs showed spastic tonus in their hindlimbs. Further, these dogs showed improvement in their BBB scores over the observation period.
For forelimbs of dogs subjected to compression for 3 days, the amplitudes of MEPs induced by electrical stimulation showed minimal changes. However, there was a significant reduction in the MEP amplitude for the hindlimbs of these dogs (Figure 1). Figures 2 and 3 show the CMAPs of a dog subjected to compression for 3 days. Forelimb movements were evoked by magnetic stimulation at the cervical spine and cranial areas (Figure 2) of these dogs. However, hindlimb movements were not evoked by magnetic stimulation at these areas, as shown in Figure 3.
To date, rats have been the most commonly used animals for studies of SCI because they are economical to obtain and maintain and are readily available.6) However, the rat spinal cord is much smaller than that of humans. If the rat cerebral cortex is destroyed, delicate paw movements are disturbed.11) However, injury to the rat corticospinal tract is known to have minimal effects on standing posture and gait function.8,13) Thus, the role of the extrapyramidal tract is more distributed for motor function.8) Furthermore, unlike the human counterpart, the corticospinal tract of the rat is known to be relatively unimportant for motor function. Therefore, the rat model is not suitable for detailed physical analyses or accurate evaluation of recovery. In canine models, physical examinations can be carried out more easily, and more detailed pathophysiological studies can be conducted using magnetic resonance imaging, clinical angiography systems, and clinical computed tomography systems. The results of this study indicate that canine paraplegic models can be produced by using a balloon catheter inflated for 3 days. This technique is simple and takes about 15 minutes, with few complications associated with the operation.
Compression is one of the methods used to induce an SCI in a safe manner and yielded injury similar to human SCI.10) Furthermore, complete spinal injuries generally do not heal, regardless of intervention, whereas minor injuries can show improvement with little or no therapy. As mentioned above, rats have been most commonly used for SCI studies. However, the rat SCI model has shown improvement in motor function of the extremities without any treatment. Therefore, researchers had to compare the degree of motor function improvement between the treated and non-treated group. In our SCI model, postoperative observation of the dogs indicated that compression for 3 days was equal to, or longer than, the minimum compression time necessary to produce irreversible paraplegia. On the other hand, in dogs subjected to compression for 1 or 2 days, varying degrees of hindlimb movement were evident after surgery. Therefore, producing irreversible and complete paraplegia requires compression for 3 days. Our 3-day compression SCI model in canines is comparable to the complete injury model, and the dogs showed no improvement in motor or urinary function until they were euthanized (from 1 day to 3 months after surgery).
This study has some limitations. In accordance with the nature of the animal model and the lesion type, motor and somatosensory evoked potentials may be affected. Furthermore, although the same procedure was used to induce complete paraplegia, the type and severity of the SCI may depend on the compression pressure and exact location of the balloon in the spinal canal. Therefore, attempting neurological evaluation of animal models by electrophysiological assessment, we have to consider the anatomical location and extent of lesions of the SCI. Another factor to be considered is the effect of the anesthetics. Anesthetics are known to affect motor and somatosensory evoked potentials.14) In this study, in order to minimize the effects of anesthesia, we performed shallow anesthesia so that an electrical response was evident at the time of recording and at a minimum of 2 hours after anesthesia induction in order to minimize the effects of muscle relaxants. We believe future research comparing the electrophysiological changes according to the location of the lesion, compression pressure, compression time, and degree of tissue damage is needed.
Notes
References
1. Aguilar RM, Steward O. A bilateral cervical contusion injury model in mice: assessment of gripping strength as a measure of forelimb motor function. Exp Neurol. 2010; 221:38–53. PMID: 19815010.


2. Basso DM, Beattie MS, Bresnahan JC. A sensitive and reliable locomotor rating scale for open field testing in rats. J Neurotrauma. 1995; 12:1–21. PMID: 7783230.


3. Chiba A, Oshio K, Inase M. Magnetically evoked EMGs in rats. Neurol Res. 2003; 25:87–91. PMID: 12564132.


4. Couto PA, Filipe VM, Magalhães LG, Pereira JE, Costa LM, Melo-Pinto P, et al. A comparison of two-dimensional and three-dimensional techniques for the determination of hindlimb kinematics during treadmill locomotion in rats following spinal cord injury. J Neurosci Methods. 2008; 173:193–200. PMID: 18606186.


5. Estenne M, Pinet C, De Troyer A. Abdominal muscle strength in patients with tetraplegia. Am J Respir Crit Care Med. 2000; 161(3 Pt 1):707–712. PMID: 10712311.


6. Fukuda S, Nakamura T, Kishigami Y, Endo K, Azuma T, Fujikawa T, et al. New canine spinal cord injury model free from laminectomy. Brain Res Brain Res Protoc. 2005; 14:171–180. PMID: 15795171.


7. Jeffery ND, Lakatos A, Franklin RJ. Autologous olfactory glial cell transplantation is reliable and safe in naturally occurring canine spinal cord injury. J Neurotrauma. 2005; 22:1282–1293. PMID: 16305316.


8. Kamida T, Fujiki M, Hori S, Isono M. Conduction pathways of motor evoked potentials following transcranial magnetic stimulation: a rodent study using a "figure-8" coil. Muscle Nerve. 1998; 21:722–731. PMID: 9585325.


9. Kang SK, Shin MJ, Jung JS, Kim YG, Kim CH. Autologous adipose tissue-derived stromal cells for treatment of spinal cord injury. Stem Cells Dev. 2006; 15:583–594. PMID: 16978061.


10. Khan M, Griebel R, Rozdilsky B, Politis M. Hemorrhagic changes in experimental spinal cord injury models. Can J Neurol Sci. 1985; 12:259–262. PMID: 4052887.


11. K L. The Cerebral Cortex of the Rat. Bryan Kolb and Richard C. Tees, Eds. MIT Press, Cambridge, MA, 1990. xii, 645 pp., illus. Paper, $35. A Bradford Book. Science. 1990; 250:1457.
12. Lim JH, Byeon YE, Ryu HH, Jeong YH, Lee YW, Kim WH, et al. Transplantation of canine umbilical cord blood-derived mesenchymal stem cells in experimentally induced spinal cord injured dogs. J Vet Sci. 2007; 8:275–282. PMID: 17679775.


13. Loy DN, Talbott JF, Onifer SM, Mills MD, Burke DA, Dennison JB, et al. Both dorsal and ventral spinal cord pathways contribute to overground locomotion in the adult rat. Exp Neurol. 2002; 177:575–580. PMID: 12429203.


14. Luft AR, Kaelin-Lang A, Hauser TK, Cohen LG, Thakor NV, Hanley DF. Transcranial magnetic stimulation in the rat. Exp Brain Res. 2001; 140:112–121. PMID: 11500803.


15. Poirrier AL, Nyssen Y, Scholtes F, Multon S, Rinkin C, Weber G, et al. Repetitive transcranial magnetic stimulation improves open field locomotor recovery after low but not high thoracic spinal cord compression-injury in adult rats. J Neurosci Res. 2004; 75:253–261. PMID: 14705146.


16. Shyu WC, Lin SZ, Chiang MF, Su CY, Li H. Intracerebral peripheral blood stem cell (CD34+) implantation induces neuroplasticity by enhancing beta1 integrin-mediated angiogenesis in chronic stroke rats. J Neurosci. 2006; 26:3444–3453. PMID: 16571751.


FIGURE 1
Electrophysiological recordings after electrical stimulation in a dog subjected to 3 days of compression. A: Forelimb. B: Hindlimb. Forelimb recordings after 3-day compression showed minimal changes in amplitudes. However, the hindlimb recordings after 3-day compression showed a significant amplitude reduction.
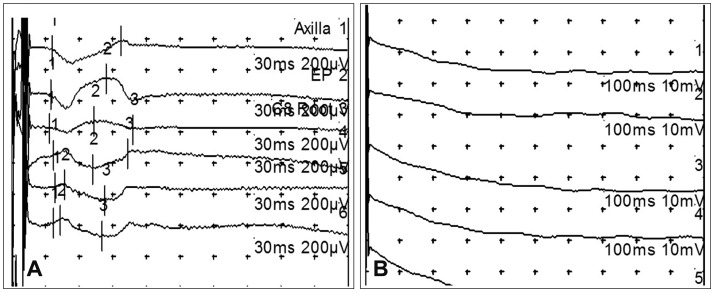