Abstract
Objectives
The purpose of this study was to evaluate the performance of half-dose chest CT using an iterative reconstruction technique in patients with lung malignancies.
Methods
The Dual-source CT scans were obtained and half-dose datasets were reconstructed with 5 different strengths in 38 adults with lung malignancies. Two radiologists graded subjective image quality; noise, contrast and sharpness at the central/peripheral lung, mediastinum and chest wall of the reconstructed half-dose images, compared with those of standard-dose images, using a three-point scale. A lesion assessment; lesion conspicuity and diagnostic confidence, was also performed. The quantitative image noises; contrast-to-noise ratio (CNR) and signal-to-noise ratio (SNR) were measured and compared with those of standard-dose images.
Results
The subjective image noise in the half-dose images was less than that of the standard-dose images. The contrast in strengths 2 to 5 was superior, the sharpness of the lung parenchyma in strengths 3 to 5 was inferior, and the CNR/SNR in all strengths were higher than those of standard-dose images (P < 0.05). The improvement of subjective image noise and contrast, the decrease in sharpness, were correlated with strength level (P < 0.05). The lesion conspicuity in half-dose images of strengths 4 and 5 was decreased. The diagnostic confidence of the half-dose images of all strengths was comparable to that of the standard-dose images (P < 0.05).
Over the past decade in South Korea, the annual number of computed tomography (CT) examinations has increased from approximately 1.7 million in 2003 to 4.8 million in 2009.1 The patient radiation dose from CT is the highest of all imaging studies, with CT contributing more than 40% of the estimated collective effective radiation dose to the population from medical imaging.2 In patients with lung malignancy, in whom radiation exposure poses a worrisome risk, chest CT is performed repeatedly to evaluate primary and metastatic lesions. The high inherent contrast between inhaled air and the lung parenchyma should theoretically allow a reduction in radiation dose without compromising image quality. Although the radiation dose can be reduced with scanner technology, low-dose CT images have increased noise compared to standard-dose CT.
Recently, an alternative mathematical algorithm called iterative reconstruction has been developed to reduce image noise. The iterative reconstruction method, which is the reference method in nuclear medicine to reconstruct images, is being increasingly used with CT scans.3 There are already several commercial iterative reconstruction algorithms available for clinical use, such as iterative reconstruction in image space (IRIS; Siemens Medical Systems, Erlangen, Germany), adaptive statistical iterative reconstruction (ASIR; GE Healthcare, Waukesha, WI, USA), and adaptive iterative dose reduction (AIDR; Toshiba Medical System, Otawara, Japan).456 Sinogram affirmed iterative reconstruction (SAFIRE) is a second-generation variant of iterative reconstruction which has the unique feature of using two iteration reconstruction loops: one in the raw data domain to reduce artifact and the other in the image domain to reduce noise. Previous studies have reported that SAFIRE maintains CT image quality at low doses of radiation in coronary and other types of angiography.678 However, 5 different strengths of iteration are used to reduce noise in SAFIRE. Few studies had been conducted to evaluate the differences between the 5 different reconstruction strengths.
The purpose of this study was to evaluate the performance of half-dose chest CT using an iterative reconstruction technique based on the raw data named Sinogram Affirmed Iterative Reconstruction (SAFIRE) and to determine the optimal strength images in patients with primary or metastatic lung malignancies.
Institutional review board approval was obtained and individual patient consent was not required; the retrospective analysis of data was possible by generating the two series of reconstructions in only one routine CT scan in each patient (Institutional Review Board of Kosin University Gospel Hospital, No. 13-035). From March to July 2013, we enrolled 38 consecutive patients (22 men and 16 women) who underwent follow-up chest CT examinations for therapeutic evaluation of primary lung cancer or metastatic pulmonary lesions from other extra-thoracic malignancies. The underlying malignancies were located in the lung (n = 8), colon (n = 7), head and neck (n = 4), stomach (n = 3), kidney (n = 3), bladder (n = 3), breast (n = 2) and other locations (n = 8). The mean age was 60.2 years (range 37-78 years). The mean body mass index (BMI) of the patients was 23.15 ± 3.88 kg/m2 (range, 17.0-36.5 kg/m2). They all had solitary or multiple pulmonary lesions which were solid nature. The mean size of the lesions was 23 ± 5.24 mm (range 5-62 mm).
All CT scans were obtained in the supine position after IV contrast administration with a dual-source Flesh 128-slice multi-detector CT system (SOMATOM Definition Flash; Siemens Medical Solutions, Forchheim, Germany). The standard-dose CT scans were performed under automated dose modulation using two tubes in dual-source modes with the following settings: 120 kVp, 100-230 mA; tube rotation time, 0.5 seconds; pitch 1.2 (CARE Dose 4D; Siemens Healthcare, Erlangen, Germany). The half-dose data sets (50-115 mA) were obtained using projection data from only one of the two x-ray detectors in this dual-source CT system.
From each CT image, 6 image serials were reconstructed. First a standard-dose image serial was reconstructed using a conventional filtered back projection algorithm to evaluate the lung and mediastinal images using kernel B60f. Scanned raw data were exported to an external hard drive and 5 half-dose SAFIRE image serials (strength 1-5; S1-S5) were reconstructed on an offline workstation provided by the vendor (Siemens) using kernel B50f. In order to compare standard-dose and half-dose SAFIRE images at a radiation dose ratio of 2:1, SAFIRE reconstructions used the data acquired from only one tube of the dual-source CT system. All data sets were reconstructed with a slice thickness of 3 mm in increments of 3 mm. Consecutive CT examinations were dicomized and displayed with a window level of -700 Hounsfield units (HU) and width of 1500 HU for lung images, as well as a window level of 25 HU and width of 400 HU for mediastinal images.
Two independent radiologists (reader 1, B.S.K. and reader 2, J.G.B with 4 and 7 years of experience, respectively) evaluated the CT scans. They were blinded to image strength and independently compared the half-dose SAFIRE images with corresponding standard-dose images in the same patient at a similar level in a side-by-side manner on two monitors using a digital picture-archiving and communicating system diagnostic workstation (PACS; Marosis m-view, Infinitt, Korea). The radiologists reviewed the images at a constant window width and level to simulate both lung and mediastinal window settings. Images were assessed for the following 10 factors: lung noise, lung contrast, central vessel and airway sharpness, peripheral vessel and airway sharpness (i.e., within 2 cm of the parietal pleura), mediastinal noise, mediastinal contrast, mediastinal sharpness, chest wall noise, chest wall contrast and chest wall sharpness. In comparison with the corresponding baseline images, the factors were graded as better than (score 2), equal to (score 1), or worse than (score 0) the standard-dose images. The subjective image noise was defined as overall graininess or mottling in the lung parenchyma, mediastinum or chest wall. The contrast was scored on the basis of relative ability to discern various anatomic structures with different densities. Sharpness of the lung vessels, mediastinal structures and chest wall were assessed on the basis of visually sharp reproduction of the structures.9 In addition, the conspicuity of pulmonary lesions was analyzed using 5-point scale (1 = no lesion seen, 2 = questionable lesion or an artifact mimicking a lesion, 3 = subtle lesion with ill-defined margin, 4 = well-visualized lesion with ill-defined margins, 5 = well-visualized lesion with sharp margins). Diagnostic confidence was evaluated using 3-point scale (1 = unacceptable for diagnostic interpretation, 2 = confidence limited to only large or well-defined lesions, 3 = fully confident).10
We obtained the quantitative measurements on all 228 images (6 images × 38 patients) of attenuation values (in Hounsfield units) and image noise (SD of attenuation coefficients) in the descending thoracic aorta and chest wall muscle of subscapularis at the level of inferior pulmonary vein with a region-of-interest (ROI) of a constant size and shape. Signal-to-noise ratio (SNR) and contrast-to-noise ratio (CNR) of the descending thoracic aorta with respect to the chest wall muscle were calculated for all images according to the following standard equations:
All data were analyzed using SPSS version 20.0 software (SPSS Inc, Chicago, Il). For each subset of standard-dose and reconstructed half-dose images, subjective image noise, sharpness and contrast scores for all 10 factors and lesion assessments (conspicuity and diagnostic confidence) were reported as the mean ± standard error. Individual scores of subjective image factors were compared using the Wilcoxon signed-rank test. Objective image noise including SNR and CNR was compared using the paired t-test. The correlation between subjective factors and objective image noise (SNR and CNR) was determined using the Spearman correlation test. Significant correlation was defined as a difference with a 2-sided p-value less than 0.05. The Cohen κ test was used to assess the degree of intra- and inter-observer agreement between the two readers. The κ coefficient value was considered as follows: slight (< 0.20), fair (0.21 - 0.40), moderate (0.41 - 0.60), substantial (0.61 - 0.80); or near-perfect (0.81 - 1.00).
Moderate inter-observer agreement was noted between the two independent radiologists (simple κ coefficient, 0.51 ; P < 0.05). Intra-observer agreement was moderate (simple κ coefficient, 0.48; P < 0.05) determined by reader 1, who was blinded to the previous reconstructed half-dose image information.
The mean subjective image noise, sharpness, contrast, and lesion assessment scores of standard-dose and five subsets of half-dose images reconstructed with SAFIRE are summarized in (Table 1). The score above 1 means the subjective factor is better and the score less than 1 means the subjective factor is worse than the corresponding standard dose image. There was significantly less subjective image noise in the lung, mediastinum and chest wall of the half-dose images reconstructed with strengths 1 to 5 compared to the corresponding standard-dose images (P < 0.05). Subjective image noise was positively correlated with strength (P < 0.05) (Fig. 1). Compared to standard-dose images, there was significantly less central and peripheral lung sharpness in the S4 and S5 half-dose images, mediastinal sharpness in the S3-S5 half-dose images, and chest wall sharpness in the S2-S5 half-dose images (P < 0.05). The reduction in sharpness was correlated with strength (P < 0.05) and associated with loss of detailed visualization of the small vascular structures in the peripheral or central lung fields, mediastinum, or chest wall (Fig. 2). There was a statistically significant improvement in lung contrast of the half-dose images of all strengths, as well as in mediastinum and chest wall contrast in S2-S5 images (P < 0.05). Image contrast was positively correlated with strength (P < 0.05). Lesion conspicuity and diagnostic confidence of reconstructed half-dose images are demonstrated in (Table 2). Lesion conspicuity was significantly decreased in the S4-S5 half-dose images (P < 0.05). However, the diagnostic confidence was fully confident in all half-dose image strengths. In particular, S2 and S3 reconstructed images had the lowest subjective image noise while maintaining sharpness of the lung parenchyma and lesion conspicuity (Fig. 2).
The SNR and CNR of standard-dose and reconstructed half-dose images are presented in (Table 3). CNR and SNR, which were both positively correlated with strength (P < 0.05), were significantly higher in reconstructed half-dose images of all strengths compared to standard-dose images (P < 0.05). There was a statistically significant correlation between the subjective image noise assessment and the quantitative image noise, including SNR and CNR, of the descending thoracic aorta (Spearman correlation coefficient, 1.0 in lung, 0.99 in mediastinum and chest wall; P < 0.01).
The increase in CT use has raised concerns regarding its carcinogenic potential from irradiation of the thorax. In recent years, many clinicians and radiologists have begun to use low-dose chest CT for screening and diagnostic purposes. However, reductions in radiation dose result in increased image noise, which is one of the most important attributes of image quality. Various technical advances to improve the image quality of low-dose CT scans have been developed or are in the experimental stage.111213
The term iteration refers to series of multiple passes or repetitions of mathematical calculations in an image reconstruction chain to achieve the desired CT image quality. The noise content is estimated and subtracted from the current data set in each iteration. Afterward, the results are compared with the initial data, leading to an updated image, and added to the previous data set before the next iteration is performed. This procedure can be regarded as a validation loop.14 The iterative reconstruction algorithm is based on a correction loop within the image generation process. The novel raw data-based iterative reconstruction algorithm, SAFIRE, uses two processes: first, raw data are projected backwards and forwards to correct geometrical imperfections and reduce image artifact. Second, data are sent to the image space iteration loops to reduce image noise. The looping process controls the strength of noise reduction, which users can set on a scale of 1 to 5, with 1 being the weakest and 5 being the strongest noise reduction.6715
This study demonstrates that half-dose CT images reconstructed using SAFIRE were superior to standard-dose images in regards to subjective image noise and contrast. The improvements in subjective image noise and contrast were correlated with the strength. The signal-to-noise ratio (SNR) and contrast-to-noise ratio (CNR) were also improved with the increase in SAFIRE level. Several investigators have reported that a number of iterative reconstruction techniques available for clinical use in low-dose CT can reduce image noise: SAFIRE, iterative reconstruction in image space (IRIS; Siemens Medical Systems, Erlangen, Germany), adaptive statistical iterative reconstruction (ASIR; GE Healthcare, Waukesha, WI, USA), and adaptive iterative dose reduction (AIDR; Toshiba Medical Systems, Otawara, Japan).45671016171819 Noise reduction is less marked and perhaps less necessary in the lung window because the high background contrast in the lung parenchyma's air-soft tissue interfaces renders structures and lesions distinctly visible.10
This study found that the sharpness of the half-dose SAFIRE images was inferior to that of the standard-dose image. The texture pattern of the images with higher reconstruction strength resulted in excessive smoothing and pixilation, as well as different textures, producing a so-called “blotchy pixelated appearance”.20 Interestingly, this suboptimal appearance has also been reported at higher iterative strengths in several prior studies using different hybrid iterative reconstruction techniques.2021222324 Although the lower noise level is preferable for diagnostic purposes, we found that the strongest reconstruction strength did not always result in the best image quality. Therefore, it is important to determine the optimal SAFIRE strength to use in clinical practice. Although decreased sharpness was noted with increased SAFIRE strength, half-dose S2 and S3 images had lung parenchyma sharpness, lesion conspicuity and diagnostic confidence comparable to those of standard-dose images in this study. In other recent studies, Baumueller et al. selected S3 and S4, Yang et al. selected S3, and another group selected S2 and S3, as the optimal strength levels for low-dose lung CT.161719 These differences may be influenced by body mass index (BMI), given that image noise is higher in patients with higher BMIs.25 The mean BMI in previous studies is slightly higher than in our study. Taken together, the results of this and previous studies suggest that S3 is the optimal strength for half-dose SAFIRE chest CT.
This study has several limitations. First, all CT scans were obtained with intravenous contrast, which provides higher signal-to-noise and contrast-to-noise ratios than non-contrast images. Second, the standard-dose images and half-dose images with SAFIRE were reconstructed with different kernels. A slightly higher B-value was used in standard-dose images, which causes image mottling and graininess. Third, ground-glass opacities were not evaluated in this study and remain to be investigated. Fourth, the mean patient BMI was relatively low, likely related to their underlying malignancies, which limits the generalizability of our results to patients with higher BMIs. Future studies would benefit from a larger patient population that includes obese patients.
The half-dose images reconstructed with SAFIRE had decreased subjective and quantitative image noise and increased contrast compared to corresponding standard-dose images. Although the image sharpness and lesion conspicuity were decreased in high strength S4 and S5 images, images reconstructed with S2 and S3 maintained lung parenchyma sharpness, lesion conspicuity, and diagnostic confidence comparable to those of standard-dose images.
In conclusion, half-dose chest CT images using SAFIRE can improve image quality, as evidenced by decreased noise and increased contrast, while resulting in diagnostic confidence comparable to standard-dose images. In addition, image reconstruction with strength levels 2 and 3 appear to be the optimal choice in clinical practice by maintaining lung parenchyma sharpness and lesion conspicuity.
Figures and Tables
Fig. 1
52-year-old woman with stomach cancer. The reconstructed half-dose CT images with 5 strengths (b, strength 1; c, strength 2; d, strength 3; e, strength 4; f, strength 5) show decreased image noise in the aorta and mediastinal fat compared to the standard-dose image (a). Image noise was inversely correlated with strength.
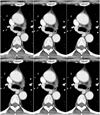
Fig. 2
53-year-old woman with rectal cancer. The reconstructed half-dose CT images with strength 3, 4 and 5 (d, e and f) show poor visualization of the small vascular structures in the peripheral 2 cm of the lung compared to the standard-dose image (a). Image sharpness was inversely correlated with strength (b, strength 1; c, strength 2; d, strength 3; e, strength 4; f, strength 5). However, the conspicuity of the small metastatic pulmonary nodules is comparable to the standard-dose image.
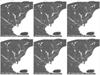
Table 1
Scores of subjective factors including noise, contrast and sharpness in the lung, mediastinum and chest wall in each half-dose image reconstructed with different 5 strengths of SAFIR
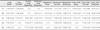
References
1. Park MY, Jung SE. CT radiation dose and radiation reduction strategies. J Korean Med Assoc. 2011; 54:1262–1268.


2. Crawley MT, Booth A, Wainwright A. A practical approach to the first iteration in the optimization of radiation dose and image quality in CT: estimates of the collective dose savings achieved. Br J Radiol. 2001; 74:607–614.


3. Greffier J, Fernandez A, Macri F, Freitag C, Metge L, Beregi JP. Which dose for what image? Iterative reconstruction for CT scan. Diagn Interv Imaging. 2013; 94:1117–1121.


4. Hara AK, Paden RG, Silva AC, Kujak JL, Lawder HJ, Pavlicek W. Iterative reconstruction technique for reducing body radiation dose at CT: feasibility study. AJR Am J Roentgenol. 2009; 193:764–771.


5. Liu YJ, Zhu PP, Chen B, Wang JY, Yuan QX, Huang WX, et al. A new iterative algorithm to reconstruct the refractive index. Phys Med Biol. 2007; 52:L5–L13.


6. Moscariello A, Takx RA, Schoepf UJ, Renker M, Zwerner PL, O'Brien TX, et al. Coronary CT angiography: image quality, diagnostic accuracy, and potential for radiation dose reduction using a novel iterative image reconstruction technique-comparison with traditional filtered back projection. Eur Radiol. 2011; 21:2130–2138.


7. Winklehner A, Karlo C, Puippe G, Schmidt B, Flohr T, Goetti R, et al. Raw data-based iterative reconstruction in body CTA: evaluation of radiation dose saving potential. Eur Radiol. 2011; 21:2521–2526.


8. Wang R, Schoepf UJ, Wu R, Gibbs KP, Yu W, Li M, et al. CT coronary angiography: image quality with sinogram-affirmed iterative reconstruction compared with filtered back-projection. Clin Radiol. 2013; 68:272–278.


9. Kalra MK, Wittram C, Maher MM, Sharma A, Avinash GB, Karau K, et al. Can noise reduction filters improve low-radiation-dose chest CT images? Pilot study. Radiology. 2003; 228:257–264.


10. Kalra MK, Woisetschläger M, Dahlström N, Singh S, Digumarthy S, Do S, et al. Sinogram-Affirmed Iterative Reconstruction of Low-Dose Chest CT: Effect on Image Quality and Radiation Dose. AJR Am J Roentgenol. 2013; 201:W235–W244.


11. McNitt-Gray MF. AAPM/RSNA Physics Tutorial for Residents: Topics in CT. Radiation dose in CT. Radiographics. 2002; 22:1541–1553.


12. Lee TY, Chhem RK. Impact of new technologies on dose reduction in CT. Eur J Radiol. 2010; 76:28–35.


13. Prasad SR, Wittram C, Shepard JA, McLoud T, Rhea J. Standard-dose and 50%-reduced-dose chest CT: comparing the effect on image quality. AJR Am J Roentgenol. 2002; 179:461–465.


14. Kalra MK, Woisetschläger M, Dahlström N, Singh S, Lindblom M, Choy G, et al. Radiation dose reduction with Sinogram Affirmed Iterative Reconstruction technique for abdominal computed tomography. J Comput Assist Tomogr. 2012; 36:339–346.


15. Wang R, Schoepf UJ, Wu R, Reddy RP, Zhang C, Yu W, et al. Image quality and radiation dose of low dose coronary CT angiography in obese patients: sinogram affirmed iterative reconstruction versus filtered back projection. Eur J Radiol. 2012; 81:3141–3145.


16. Baumueller S, Winklehner A, Karlo C, Goetti R, Flohr T, Russi EW, et al. Low-dose CT of the lung: potential value of iterative reconstructions. Eur Radiol. 2012; 22:2597–2606.


17. Hwang HJ, Seo JB, Lee HJ, Lee SM, Kim EY, Oh SY, et al. Low-dose chest computed tomography with sinogram-affirmed iterative reconstruction, iterative reconstruction in image space, and filtered back projection: studies on image quality. J Comput Assist Tomogr. 2013; 37:610–617.


18. Wang H, Tan B, Zhao B, Liang C, Xu Z. Raw-data-based iterative reconstruction versus filtered back projection: image quality of low-dose chest computed tomography examinations in 87 patients. Clin Imaging. 2013; 37:1024–1032.


19. Yang WJ, Yan FH, Liu B, Pang LF, Hou L, Zhang H, et al. Can sinogram-affirmed iterative (SAFIRE) reconstruction improve imaging quality on low-dose lung CT screening compared with traditional filtered back projection (FBP) reconstruction? J Comput Assist Tomogr. 2013; 37:301–305.


20. Singh S, Kalra MK, Gilman MD, Hsieh J, Pien HH, Digumarthy SR, et al. Adaptive statistical iterative reconstruction technique for radiation dose reduction in chest CT: a pilot study. Radiology. 2011; 259:565–573.


21. Prakash P, Kalra MK, Ackman JB, Digumarthy SR, Hsieh J, Do S, et al. Diffuse lung disease: CT of the chest with adaptive statistical iterative reconstruction technique. Radiology. 2010; 256:261–269.


22. Leipsic J, Nguyen G, Brown J, Sin D, Mayo JR. A prospective evaluation of dose reduction and image quality in chest CT using adaptive statistical iterative reconstruction. AJR Am J Roentgenol. 2010; 195:1095–1099.


23. Singh S, Kalra MK, Hsieh J, Licato PE, Do S, Pien HH, et al. Abdominal CT: comparison of adaptive statistical iterative and filtered back projection reconstruction techniques. Radiology. 2010; 257:373–383.


24. Marin D, Nelson RC, Schindera ST, Richard S, Youngblood RS, Yoshizumi TT, et al. Low-tube-voltage, high-tube-current multi-detector abdominal CT: improved image quality and decreased radiation dose with adaptive statistical iterative reconstruction algorithm--initial clinical experience. Radiology. 2010; 254:145–153.

