Abstract
Early detection of carious lesions increases the possibility of treatment without the need for surgical intervention. Optical coherence tomography (OCT) is an emerging three-dimensional imaging technique that has been successfully used in other medical fields, such as ophthalmology for optical biopsy, and is a prospective candidate for early caries detection. The technique is based on low coherence interferometry and is advantageous in that it is non-invasive, does not use ionizing radiation, and can render three-dimensional images. A brief history of the development of this technique and its principles are discussed in this paper. There have been numerous studies on caries detection, which were mostly in vitro or ex vivo experiments. Through these studies, the feasibility of OCT for caries detection was confirmed. However, further research should be performed, including in vivo studies of OCT applications, in order to prove the clinical usefulness of this technique. In addition, some technological problems must be resolved in the near future to allow for the use of OCT in everyday practice.
References
1. Pereira AC, Verdonschot EH, Huysmans MC. Caries detection methods: can they aid decision making for invasive sealant treatment? Caries Res. 2001; 35:83–89.
2. Shimada Y, Sadr A, Burrow MF, Tagami J, Ozawa N, Sumi Y. Validation of swept-source optical coherence tomography (SS-OCT) for the diagnosis of occlusal caries. J Dent. 2010; 38:655–665.


3. Bader JD, Shugars DA, Bonito AJ. Systematic reviews of selected dental caries diagnostic and management methods. J Dent Educ. 2001; 65:960–968.


4. Featherstone JD. The continuum of dental caries-evidence for a dynamic disease process. J Dent Res. 2004; (83 Spec No C):C39–42.


5. Kidd EA, Fejerskov O. What constitutes dental caries? Histopathology of carious enamel and dentin related to the action of cariogenic biofilms. J Dent Res. 2004; (83 Spec No C):C35–38.


6. Featherstone JD. Prevention and reversal of dental caries: role of low level fluoride. Community Dent Oral Epidemiol. 1999; 27:31–40.


7. Jones RS, Darling CL, Featherstone JD, Fried D. Imaging artificial caries on the occlusal surfaces with polarization-sensitive optical coherence tomography. Caries Res. 2006; 40:81–89.


8. Popescu DP, Sowa MG, Hewko MD, Choo-Smith LP. Assessment of early demineralization in teeth using the signal attenuation in optical coherence tomography images. J Biomed Opt. 2008; 13:054053.


9. Health NIo. Diagnosis and manangement of dental caries throughout life; National Institutes of Health Consensus Development Conference statement Diagnosis and management of dental caries throughout life, March 26-28, 2001. J Am Dent Assoc. 2001; 132:1153–1161.
10. Bashkansky M, Reintjes J. Statistics and reduction of speckle in optical coherence tomography. Opt Lett. 2000; 25:545–547.


11. Popescu D. Speckle noise attenuation in optical coherence tomography by compounding images acquired at different positions of the sample. Opt Commun. 2006; 269:247–251.


12. Wojtkowski M. High-speed optical coherence tomography: basics and applications. Appl Opt. 2010; 49:D30–61.


13. Tomlins PH, Wang RK. Theory, developments and applications of optical coherence tomography. J Phys D Appl Phys. 2005; 38:2519–2535.


14. Fujimoto J. Introduction to optical coherence tomography. Drexler W, Fujimoto JG editors, editors. Optical coherence tomography. Springer;2008. p. p1–45.


15. Flournoy PA, McClure RW, Wyntjes G. White-light interferometric thickness gauge. Appl Opt. 1972; 11:1907–1915.


16. Li T, Wang A, Murphy K, Claus R. White-light scanning fiber Michelson interferometer for absolute position-distance measurement. Opt Lett. 1995; 20:785–787.


17. Maruyama H, Inoue S, Mitsuyama T, Ohmi M, Haruna M. Low-coherence interferometer system for the simultaneous measurement of refractive index and thickness. Appl Opt. 2002; 41:1315–1322.


18. Huang D, Swanson EA, Lin CP, Schuman JS, Stinson WG, Chang W, Hee MR, Flotte T, Gregory K, Puliafito CA, Fujimoto JG. Optical coherence tomography. Science. 1991; 254:1178–1181.


19. Colston BW Jr, Everett MJ, Da Silva LB, Otis LL, Stroeve P, Nathel H. Imaging of hard-and soft-tissue structure in the oral cavity by optical coherence tomography. Appl Opt. 1998; 37:3582–3585.
20. Tsai MT, Lee HC, Lu CW, Wang YM, Lee CK, Yang CC, Chiang CP. Delineation of an oral cancer lesion with swept-source optical coherence tomography. J Biomed Opt. 2008; 13:044012.


21. Tsai MT, Lee CK, Lee HC, Chen HM, Chiang CP, Wang YM, Yang CC. Differentiating oral lesions in different carcinogenesis stages with optical coherence tomography. J Biomed Opt. 2009; 14:044028.


22. Lee CK, Tsai MT, Lee HC, Chen HM, Chiang CP, Wang YM, Yang CC. Diagnosis of oral submucous fibrosis with optical coherence tomography. J Biomed Opt. 2009; 14:054008.


23. Tsai MT, Lee HC, Lee CK, Yu CH, Chen HM, Chiang CP, Chang CC, Wang YM, Yang CC. Effective indicators for diagnosis of oral cancer using optical coherence tomography. Opt Express. 2008; 16:15847–15862.
24. Wilder-Smith P, Osann K, Hanna N, El Abbadi N, Brenner M, Messadi D, Krasieva T. In vivo multiphoton fluorescence imaging: a novel approach to oral malignancy. Lasers Surg Med. 2004; 35:96–103.


25. Wilder-Smith P, Hammer-Wilson MJ, Zhang J, Wang Q, Osann K, Chen Z, Wigdor H, Schwartz J, Epstein J. In vivo imaging of oral mucositis in an animal model using optical coherence tomography and optical Doppler tomography. Clin Cancer Res. 2007; 13:2449–2454.


26. Wilder-Smith P, Krasieva T, Jung WG, Zhang J, Chen Z, Osann K, Tromberg B. Noninvasive imaging of oral premalignancy and malignancy. J Biomed Opt. 2005; 10:051601.


27. Na J, Lee BH, Baek JH, Choi ES. Optical approach for monitoring the periodontal ligament changes induced by orthodontic forces around maxillary anterior teeth of white rats. Med Biol Eng Comput. 2008; 46:597–603.


28. Baek JH, Na J, Lee BH, Choi E, Son WS. Optical approach to the periodontal ligament under orthodontic tooth movement: a preliminary study with optical coherence tomography. Am J Orthod Dentofacial Orthop. 2009; 135:252–259.


29. Simonsohn G. Die Verteilung des Brechungsindex in der Augenlinse. Optik. 1969; 29:81–86.
30. Rassow B. The retinal resolving power measured by laser interference fringes. Proc SPIE. 1978; 164:154–157.
31. Fercher A. In vivo Measurement of Fundus Pulsations by Laser Interferometry. IEEE J Qu El. 1984; 20:1469–1471.


33. Fercher AF, Mengedoht K, Werner W. Eye-length measurement by interferometry with partially coherent light. Opt Lett. 1988; 13:186–188.


34. Fercher A. Measurement of intraocular optical distances using partially coherent laser light. JMO. 1991; 38:1327–1333.


35. Huang D, Wang J, Lin CP, Puliafito CA, Fujimoto JG. Micron-resolution ranging of cornea anterior chamber by optical reflectometry. Lasers Surg Med. 1991; 11:419–425.


36. Santodomingo-Rubido J, Mallen EA, Gilmartin B, Wolffsohn JS. A new non-contact optical device for ocular biometry. Br J Ophthalmol. 2002; 86:458–462.


37. Goyal R, North RV, Morgan JE. Comparison of laser interferometry and ultrasound A-scan in the measurement of axial length. Acta Ophthalmol Scand. 2003; 81:331–335.


38. Hitzenberger CK. Optical measurement of the axial eye length by laser Doppler interferometry. Invest Ophthalmol Vis Sci. 1991; 32:616–624.
39. Drexler W, Findl O, Menapace R, Rainer G, Vass C, Hitzenberger CK, Fercher AF. Partial coherence interferometry: a novel approach to biometry in cataract surgery. Am J Ophthalmol. 1998; 126:524–534.


40. Fercher AF. Ophthalmic Interferometry. von Bally G, Khanna S editors, editors. Optics in Medicine, Biology and Environmental Research. Selected Contributions to the First International Conference on Optics Within Life Sciences (OWLS I). Garmisch-Partenkirchen;Germany: 12-16 August 1990 (ICO-15 SAT). Amsterdam, London, New York, Tokyo. Elsevier; 1993. p221-228.
41. Fercher AF, Hitzenberger CK, Drexler W, Kamp G, Sattmann H. In vivo optical coherence tomography. Am J Ophthalmol. 1993; 116:113–114.


42. Swanson EA, Izatt JA, Hee MR, Huang D, Lin CP, Schuman JS, Pulliafito CA, Fujimoto JG. In vivo retinal imaging by optical coherence tomography. Opt Lett. 1993; 18:1864–1866.


43. Tearney GJ, Boppart SA, Bouma BE, Brezinski ME, Weissman NJ, Southern JF, Fujimoto JG. Scanning single-mode fiber optic catheter-endoscope for optical coherence tomography. Opt Lett. 1996; 21:543–545.


45. Sivak M. High-resolution endoscopic imaging of the GI tract using optical coherence tomography. Gastrointestin Endosc. 2001; 54:474–479.
47. Murphy B. The Evolution of Spectral Domain OCT, Ophthalmology Management. In: Ophthalmology Management. Lippincott Williams & Wilkins VisionCare Group;2008.
48. Smolka G. Optical Coherence Tomograph: technology, markets, and applications 2008-12. In: Biooptics World. Tulsa: PennWell Corp;2007.
49. Hee MR, Puliafito CA, Wong C, Duker JS, Reichel E, Schuman JS, Swanson EA, Fujimoto JG. Optical coherence tomography of macular holes. Ophthalmology. 1995; 102:748–756.


50. Wojtkowski M, Leitgeb R, Kowalczyk A, Bajraszewski T, Fercher AF. In vivo human retinal imaging by Fourier domain optical coherence tomography. J Biomed Opt. 2002; 7:457–463.


51. Yun S, Tearney G, Bouma B, Park B, de Boer J. Highspeed spectral-domain optical coherence tomography at 1.3 mum wavelength. Opt Express. 2003; 11:3598–3604.
52. Wojtkowski M, Srinivasan V, Fujimoto JG, Ko T, Schuman JS, Kowalczyk A, Duker JS. Three-dimensional retinal imaging with high-speed ultrahigh-resolution optical coherence tomography. Ophthalmology. 2005; 112:1734–1746.


53. Wojtkowski M, Srinivasan V, Ko T, Fujimoto J, Kowalczyk A, Duker J. Ultrahigh-resolution, highspeed, Fourier domain optical coherence tomography and methods for dispersion compensation. Opt Express. 2004; 12:2404–2422.


54. Nassif N, Cense B, Park B, Pierce M, Yun S, Bouma B, Tearney G, Chen T, de Boer J. In vivo high-resolution video-rate spectral-domain optical coherence tomography of the human retina and optic nerve. Opt Express. 2004; 12:367–376.


55. Vaarkamp J, ten Bosch JJ, Verdonschot EH. Light propagation through teeth containing simulated caries lesions. Phys Med Biol. 1995; 40:1375–1387.


56. Van de Rijke JW, Ten Bosch JJ. Optical quantification of caries-like lesions in vitro by use of a fluorescent dye. J Dent Res. 1990; 69:1184–1187.


57. Hee MR, Huang D, Swanson EA, Fujimoto JG. Polarization-Sensitive Low-Coherence Reflectometer for Birefringence Characterization and Ranging. J Opt Soc Am B Opt Phys. 1992; 9:903–908.


58. de Boer JF, Milner TE, van Gemert MJ, Nelson JS. Two-dimensional birefringence imaging in biological tissue by polarization-sensitive optical coherence tomography. Opt Lett. 1997; 22:934–936.


59. Bohren CF, Nevitt TJ. Absorption by a sphere: a simple approximation. Appl Opt. 1983; 22:774–775.


60. Chinn SR, Swanson EA, Fujimoto JG. Optical coherence tomography using a frequency-tunable optical source. Opt Lett. 1997; 22:340–342.


61. Colston B, Sathyam U, Dasilva L, Everett M, Stroeve P, Otis L, Dental OCT. Opt Express. 1998; 3:230–238.
62. Amaechi BT, Higham SM, Podoleanu AG, Rogers JA, Jackson DA. Use of optical coherence tomography for assessment of dental caries: quantitative procedure. J Oral Rehabil. 2001; 28:1092–1093.


63. Amaechi BT, Podoleanu A, Higham SM, Jackson DA. Correlation of quantitative light-induced fluorescence and optical coherence tomography applied for detection and quantification of early dental caries. J Biomed Opt. 2003; 8:642–647.


64. Amaechi BT, Podoleanu AG, Komarov G, Higham SM, Jackson DA. Quantification of root caries using optical coherence tomography and microradiography: a correlational study. Oral Health Prev Dent. 2004; 2:377–382.
65. Fried D, Xie J, Shafi S, Featherstone JD, Breunig TM, Le C. Imaging caries lesions and lesion progression with polarization sensitive optical coherence tomography. J Biomed Opt. 2002; 7:618–627.


66. Jones RS, Staninec M, Fried D. Imaging artificial caries under composite sealants and restorations. J Biomed Opt. 2004; 9:1297–1304.


67. Ngaotheppitak P, Darling CL, Fried D. Measurement of the severity of natural smooth surface (interproximal) caries lesions with polarization sensitive optical coherence tomography. Lasers Surg Med. 2005; 37:78–88.


68. Jones RS, Darling CL, Featherstone JD, Fried D. Remineralization of in vitro dental caries assessed with polarization-sensitive optical coherence tomography. J Biomed Opt. 2006; 11:014016.


69. Jones RS, Fried D. Remineralization of enamel caries can decrease optical reflectivity. J Dent Res. 2006; 85:804–808.


70. Chong SL, Darling CL, Fried D. Nondestructive measurement of the inhibition of demineralization on smooth surfaces using polarization-sensitive optical coherence tomography. Lasers Surg Med. 2007; 39:422–427.


71. Can AM, Darling CL, Ho C, Fried D. Non-destructive assessment of inhibition of demineralization in dental enamel irradiated by a lambda=9.3-microm CO2 laser at ablative irradiation intensities with PS-OCT. Lasers Surg Med. 2008; 40:342–349.
72. Hsu DJ, Darling CL, Lachica MM, Fried D. Nondestructive assessment of the inhibition of enamel demineralization by CO2 laser treatment using polarization sensitive optical coherence tomography. J Biomed Opt. 2008; 13:054027.
73. Lee C, Darling CL, Fried D. Polarization-sensitive optical coherence tomographic imaging of artificial demineralization on exposed surfaces of tooth roots. Dent Mater. 2009; 25:721–728.


74. Manesh SK, Darling CL, Fried D. Nondestructive assessment of dentin demineralization using polarization-sensitive optical coherence tomography after exposure to fluoride and laser irradiation. J Biomed Mater Res B Appl Biomater. 2009; 90:802–812.


75. Manesh SK, Darling CL, Fried D. Polarization-sensitive optical coherence tomography for the nondestructive assessment of the remineralization of dentin. J Biomed Opt. 2009; 14:044002.


76. Wu J, Fried D. High contrast near-infrared polarized reflectance images of demineralization on tooth buccal and occlusal surfaces at lambda = 1310-nm. Lasers Surg Med. 2009; 41:208–213.
77. Hirasuna K, Fried D, Darling CL. Near-infrared imaging of developmental defects in dental enamel. J Biomed Opt. 2008; 13:044011.


78. Tao YC, Fried D. Near-infrared image-guided laser ablation of dental decay. J Biomed Opt. 2009; 14:054045.


79. Le MH, Darling CL, Fried D. Automated analysis of lesion depth and integrated reflectivity in PS-OCT scans of tooth demineralization. Lasers Surg Med. 2010; 42:62–68.


80. Kang H, Jiao JJ, Lee C, Le MH, Darling CL, Fried D. Nondestructive Assessment of Early Tooth Demineralization Using Cross-Polarization Optical Coherence Tomography. IEEE journal of selected topics in quantum electronics: a publication of the IEEE Lasers Electro-opt Soc. 2010; 16:870–876.
81. Baumgartner A, Dichtl S, Hitzenberger CK, Sattmann H, Robl B, Moritz A, Fercher AF, Sperr W. Polarization-sensitive optical coherence tomography of dental structures. Caries Res. 2000; 34:59–69.


82. Feldchtein F, Gelikonov V, Iksanov R, Gelikonov G, Kuranov R, Sergeev A, Gladkova N, Ourutina M, Reitze D, Warren J. In vivo OCT imaging of hard and soft tissue of the oral cavity. Opt Express. 1998; 3:239–250.


83. Wang XJ, Milner TE, de Boer JF, Zhang Y, Pashley DH, Nelson JS. Characterization of dentin and enamel by use of optical coherence tomography. Applied opt. 1999; 38:2092–2096.


84. Everett MJBWC, Sathyam US, Silva BD, Fried D, Featherstone JD. Non-invasive diagnosis of early caries with polarization sensitive optical coherence tomography (PS-OCT) Laser in Dentistry V. SPIE;San Jose, CA: 1999. p. p177–183.


85. Otis LL, Colston BW Jr, Everett MJ, Nathel H. Dental optical coherence tomography: a comparison of two in vitro systems. Dentomaxillofac Radio. 2000; 29:85–89.


86. Ko AC, Choo-Smith LP, Hewko M, Leonardi L, Sowa MG, Dong CC, Williams P, Cleqhorn B. Ex vivo detection and characterization of early dental caries by optical coherence tomography and Raman spectroscopy. J Biomed Opt. 2005; 10:031118.


87. Choo-Smith LP, Dong CC, Cleghorn B, Hewko M. Shedding new light on early caries detection. J Can Dent Assoc. 2008; 74:913–918.
88. Sowa MG, Popescu DP, Werner J, Hewko M, Ko AC, Payette J, Dong CC, Cleqhorn B, Choo-Smith LP. Precision of Raman depolarization and optical attenuation measurements of sound tooth enamel. Anal Bioanal Chem. 2007; 387:1613–1619.


89. Li J, Bowman C, Fazel-Rezai R, Hewko M, Choo-Smith LP. Speckle reduction and lesion segmentation of OCT tooth images for early caries detection. Conf Proc IEEE Eng Med Biol Soc. 2009; 2009:1449–1452.


90. Chen Y, Otis L, Piao D, Zhu Q. Characterization of dentin, enamel, and carious lesions by a polarization-sensitive optical coherence tomography system. Appl Opt. 2005; 44:2041–2048.


91. Meng Z, Yao XS, Yao H, Liang Y, Liu T, Li Y, Wang G, Lan S. Measurement of the refractive index of human teeth by optical coherence tomography. J Biomed Opt. 2009; 14:034010.


92. Maia AM, Fonseca DD, Kyotoku BB, Gomes AS. Characterization of enamel in primary teeth by optical coherence tomography for assessment of dental caries. Int J Paediatr Dent. 2010; 20:158–164.


Figure 1.
The general scheme of an interferometric OCT setup. The linear polarizer and the polarizing beam splitter in parenthesis are equipped in PS-OCT. OCT, optical coherence tomography; PS-OCT, polarization-sensitive OCT. This illustration was partly modified with permission from the original one of Wojkowski12 by courtesy of Optical Society.
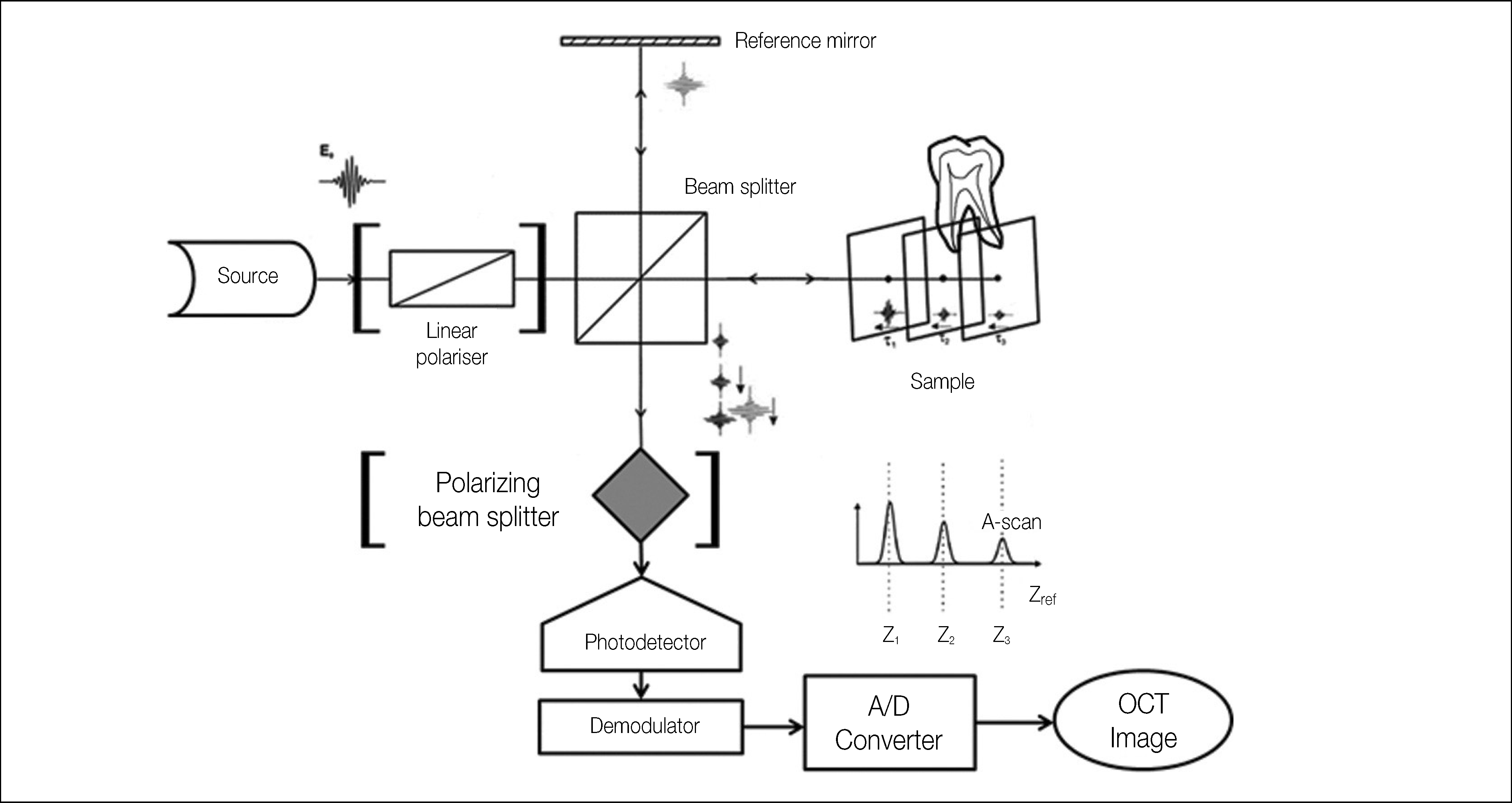