Abstract
The aim of this study was to compare the compositions and cytotoxicity of white ProRoot MTA (white mineral trioxide aggregate) and 3 kinds of Portland cements. The elements, simple oxides and phase compositions of white MTA (WMTA), gray Portland cement (GPC), white Portland cement (WPC) and fast setting cement (FSC) were measured by inductively coupled plasma atomic emission spectrometry (ICP-AES), X-ray fluorescence spectrometry (XRF) and X-ray diffractometry (XRD). Agar diffusion test was carried out to evaluate the cytotoxicity of WMTA and 3 kinds of Portland cements.
The results showed that WMTA and WPC contained far less magnesium (Mg), iron (Fe), manganese (Mn), and zinc (Zn) than GPC and FSC. FSC contained far more aluminum oxide (Al2O3) than WMTA, GPC, and WPC. WMTA, GPC, WPC and FSC were composed of main phases, such as tricalcicium silicate (3CaO·SiO2), dicalcium silicate (2CaO·SiO2), tricalcium aluminate (3CaO·Al2O3), and tetracalcium aluminoferrite (4CaO·Al2O3·Fe2O3). The significance of the differences in cellular response between WMTA, GPC, WPC and FSC was statistically analyzed by Kruskal-Wallis Exact test with Bonferroni's correction. The result showed no statistically significant difference (p > 0.05).
WMTA, GPC, WPC and FSC showed similar compositions. However there were notable differences in the content of minor elements, such as aluminum (Al), magnesium, iron, manganese, and zinc. These differences might influence the physical properties of cements.
Mineral trioxide aggregate (MTA) was developed in 1993 and has expanded its application widely. Because of its good biocompatibility1,2), physical property, and antibacterial effect3), it has been used in root end filling4-6), pulpotomy7), perforation repair8,9), coronal barrier10), and root canal filling.
In spite of these superior characteristics, the high cost of MTA limited its use in Korea. Recently many studies have been done to compare the compositions11-13), biocompatibility14-16), and physical properties17-19) of MTA and Portland cements. Many researchers suggested that Portland cement is as biocompatible and have as good physical properties as MTA.
But Dammaschke et al.20) reported that MTA contained significantly less iron (Fe) and manganese (Mn) than Portland cement and MTA cannot be simply replaced by cheaper Portland cement. But so far, only a little information about chemical compositions of Portland cement can be found in the literature20) and precise comparative study of the compositions of MTA and Portland cement is required.
The main drawback of MTA is its long setting time. The setting time of MTA was reported to be 2 h 45 min21). Experimentally, CaCl2 was added to shorten the setting time and improve the mechanical properties of MTA22,23). In this experiment, fast setting cement was used as future possible alternative for MTA. White Portland cement was also used in this experiment, which is known to be the material used for the production of white MTA24).
The purposes of this study were to investigate the compositions and evaluate the cytotoxicity of MTA (tooth colored formula, WMTA), gray Portland cement (GPC), white Portland cement (WPC), and fast setting cement (FSC).
We used GPC (Lafarge Halla cement Corp., Seoul, Korea), WPC (Union Corp., Seoul, Korea), FSC (SSangyong cement industrial Co., Seoul, Korea) and WMTA (ProRoot MTA, tooth colored formula, Dentsply Tulsa dental, Johnson city, TN, USA).
ICP-AES (ICPS-1000IV, Shimadzu, Kyoto, Japan) was used to investigate the elements that comprise WMTA, GPC, WPC and FSC. Argon Plasma (6000K) was used. Detection limit of ICP-AES was 1-10 ppb. Measurement was carried out in triplicate and the mean value was determined.
XRF (XRF-1700, Shimadzu, Kyoto, Japan) was used to investigate the relative proportions of simple oxides that comprise WMTA, GPC, WPC and FSC. We used Rh (rhodium) target and selected 40Kv and 30 mA for X-ray generator. Measurement was carried out in triplicate and the mean value was determined.
To investigate the large compounds and phases, XRD analysis (D8-advance, Bruker, Madison, WI, USA) was carried out. Phase identification was accomplished by the use of search-match software (Eva Version 9.0 Diffrac plus, Bruker, Madison, WI, USA). Target material was Cu. System capacity was 40kV and 40 mA.
XRF analysis was carried out in NCIRF (National Center for Inter-University Research Facilities, Seoul, Korea). XRD analysis was carried out in SNU DRI (Seoul National University Dental Research Institute, Seoul, Korea). ICP-AES analysis was carried out in NICEM (National Instrumentation center for environmental management, Seoul, Korea).
L929 mouse fibroblast cells were used. Cells were grown in minimum essential medium under standard cell culture conditions (37℃, 5% CO2). Experimental materials were sterilized with ethylene oxide gas. Cultured cells were seeded into 6-well plate at an initial density of 2 × 105 cells/well with 2 ml of medium and incubated for 24 hours. After 24 hours, 2 ml mixture of agar-medium was added into each well of 6-well plate and stained with Neutral Red solution. Freshly mixed MTA (water powder [w/p] ratio 1 : 1), GPC (w/p ratio 1 : 2), WPC (w/p ratio 1 : 2) and FSC (w/p ratio 1 : 2) were inserted in polyethylene tube (3 mm in inner diameter and 5 mm in height) and placed on cultured cells. For each material (WMTA, GPC, WPC, and FSC), agar diffusion test was carried out in triplicate and the mean value was determined.
6, 12, 18, and 24 hours after placing the samples, the width of decolorization zones was measured. Zinc oxide euginol (ZOE) paste and Teflon discs were used as positive and negative controls. The significance of the difference in cellular response between WMTA, GPC, WPC and FSC was statistically analyzed by Kruskal-Wallis Exact test with Bonferroni's correction.
The compositions of main elements were shown in Table 1. FSC showed remarkably high concentrations of aluminum (Al), sulfur (S), and titanium (Ti). WPC showed high concentration of Fluorine (F). The concentrations of iron, magnesium (Mg), manganese(Mn) and zinc (Zn) in WMTA and WPC were lower than those in GPC and FSC.
The results of XRF showed that calcium oxide (CaO) and silicate (SiO2) were two main simple oxides that comprise WMTA, GPC, WPC and FSC. Aluminum oxide (Al2O3) content in FSC was higher than those in WMTA, GPC and WPC. The compositions of main oxides that comprise WMTA and Portland cements were shown in Table 1.
The results of XRD analysis showed that main mineral phases comprising WMTA and Portland cements were similar (Figure 1). They were mainly tricalcicium silicate (3CaO·SiO2), dicalcium silicate (2CaO·SiO2), tricalcium aluminate (3CaO·Al2O3) and tetracalcium aluminoferrite (4CaO·Al2O3·Fe2O3). Other mineral phases such as calcium silicate (3CaO·SiO2), calcium aluminum oxide (3CaO·Al2O3), calcium aluminum oxide sulfate (3CaO·3Al2O3·CaSO4), magnesium oxide (MgO), cummingtonite ((Mg4.68 Fe2.32) Si8O22 (OH)2), magnesiocummingtonite ((Fe3.17 Mg3.83) (Si8O22(OH)2)), gehlenite (2CaO·Al2O3·SiO2) were also found in WMTA and Portland cements.
Dammaschke et al.20) analyzed the compositions of MTA and Portland cements by X-ray photoelectron spectroscopy (XPS), energy dispersive X-ray analysis (EDX), and inductively coupled plasma optical emission spectrometry (ICP-OES). Camilleri et al.25,26) studied the chemical composition with EDX and XRD. These studies were mainly focused in elemental analysis and phase identification.
MTA and Portland cement are complex compounds and they are composed of mineral phases. These mineral phases are composed of simple oxides and these simple oxides are composed of elements. So in order to investigate the chemical compositions of WMTA and Portland cements, compositions of elements that comprise simple oxides were investigated. And then, compositions of simple oxides that comprise mineral phases were measured (Figure 3). And finally, compositions of large phases that comprise WMTA and Portland cements were calculated.
There has been no study that investigated the compositions of WMTA and Portland cements from elemental level to mineral phase level. Knowing the compositions of WMTA and Portland cement from elemental level to mineral phase level can be helpful in understanding the physical properties of WMTA and Portland cement.
ICP-AES analysis showed that the concentrations of magnesium, iron, manganese and zinc in WMTA and WPC were remarkably lower than those in GPC and FSC. This explains the color differences between WPC and GPC because iron and manganese are well-known chromophores20). It was interesting that WPC had the highest fluorine content among 4 samples. We might expect some anticariogenic effect of WPC when it is used clinically. Bismuth was found only in WMTA. Bismuth was reported to decrease the mechanical strength of MTA27).
There has been few study that measured the compositions of simple oxides that comprise WMTA and Portland cements in unhydrated forms. By knowing the compositions of simple oxides, we can calculate the compositions of phases that comprise Portland cement. Two main oxides that compose WMTA and Portland cements were calcium oxide and silicate. Aluminum oxide content was remarkably high in fast setting cement, which might explain the short setting time of FSC. Bismuth oxide was known to be present in WMTA. However the amount of Bismuth oxide was not measured in this study due to technical difficulties.
The compositions of phases can be calculated from the compositions of CaO, SiO2, Al2O3, Fe2O3, and SO3 measured by XRF analysis. By Bogue's method28), the compositions of tricalcium oxide, dicalcium oxide, tricalcium aluminate and tetracalcium aluminoferrite which comprise GPC and WPC were calculated. Bogue's method was not applicable to the calculation of mineral phase of WMTA and FSC, because Bogue's method is based on the chemical assumption that Portland cement is composed entirely of CaO, SiO2, Al2O3, and Fe2O3 and this assumption does not apply to WMTA and FSC.
Min et al.29) reported that Portland cement was biocompatible and had the potential to be used in pulp capping. Cellular responses to WMTA, GPC, WPC, and FSC showed no statistically significant differences (p > 0.05).
The main elemental composition of WMTA and 3 Portland cement were similar. WMTA and WPC contained far less magnesium, iron, manganese, and zinc than GPC and FSC. WPC contained higher Fluorine than WMTA, GPC and FSC. FSC contained higher aluminum oxide than WMTA, GPC, and WPC. WMTA, GPC, WPC, and FSC were composed of tricalcicium silicate, dicalcium silicate, tricalcium aluminate, and tetracalcium aluminoferrite. The difference in cellular responses between WMTA, GPC, WPC and FSC was not statistically significant (p > 0.05). WPC showed remarkably lower heavy metal contents and higher fluorine content than GPC and FSC. This may contribute to good biocompatibility and anticariogenic effect.
Figures and Tables
Figure 1
X-ray diffraction (XRD) analysis of white MTA (a) and Portland cement (b) showing the main phase (2CaO·SiO2) present in the cement. (MTA: mineral trioxide aggregate) (Black : peaks made by specimen, Red : peaks of reference)
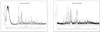
Figure 2
Cellular response to WMTA, GPC, WPC, and FSC, 6 hrs after placement of specimens.
(WMTA : white MTA, GPC : Gray Portland cement, WPC : white Portland cement, and FSC : Fast setting cement, ZOE : Zinc Oxide and Euginol)
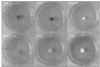
Figure 3
Schematic diagram showing the compositions of WMTA and Portland cements.
(WMTA : white mineral trioxide aggregate)
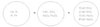
Table 1
Compositions of elements, simple oxides, and phases that comprise WMTA, GPC, WPC and FSC
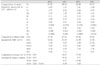
Silicon (Si) was not measured because of technical difficulty. Main phases that comprise MTA and FSC were identified by XRD analysis. But the phase compositions of MTA and FSC could not be calculated.
(WMTA: white mineral trioxide aggregate, GPC: gray Portland cement, WPC: white Portland cement, FSC: fast setting cement, XRD: X-ray diffractometry, ICP-AES: Inductively coupled plasma atomic emission spectrometry, XRF: X-ray fluorescence spectrometry)
References
1. Torabinejad M, Ford TR, Abedi HR, Kariyawasam SP, Tang HM. Tissue reaction to implanted root-end filling materials in the tibia and mandible of guinea pigs. J Endod. 1998. 24:468–471.


2. Koh ET, Torabinejad M, Pitt Ford TR, Brady K, McDonald F. Mineral trioxide aggregate stimulates a biological response in human osteoblasts. J Biomed Mater Res. 1997. 37:432–439.


3. Al-Hezaimi K, Al-Shalan TA, Naghshbandi J, Oglesby S, Simon JH, Rotstein I. Antibacterial effect of two mineral trioxide aggregate (MTA) preparations against Enterococcus faecalis and Streptococcus sanguis in vitro. J Endod. 2006. 32:1053–1056.


4. Torabinejad M, Watson TF, Pitt Ford TR. Sealing ability of a mineral trioxide aggregate when used as a root end filling material. J Endod. 1993. 19:591–595.


5. Torabinejad M, Rastegar AF, Kettering JD, Pitt Ford TR. Bacterial leakage of mineral trioxide aggregate as a root-end filling material. J Endod. 1995. 21:109–112.


6. Torabinejad M, Smith PW, Kettering JD, Pitt Ford TR. Comparative investigation of marginal adaptation of mineral trioxide aggregate and other commonly used root-end filling materials. J Endod. 1995. 21:295–299.


7. Torabinejad M, Chivian N. Clinical applications of mineral trioxide aggregate. J Endod. 1999. 25:197–205.


8. Lee SJ, Monsef M, Torabinejad M. Sealing ability of a mineral trioxide aggregate for repair of lateral root perforations. J Endod. 1993. 19:541–544.


9. Ford TR, Torabinejad M, McKendry DJ, Hong CU, Kariyawasam SP. Use of mineral trioxide aggregate for repair of furcal perforations. Oral Surg Oral Med Oral Pathol Oral Radiol Endod. 1995. 79:756–763.


10. Tselnik M, Baumgartner JC, Marshall JG. Bacterial leakage with mineral trioxide aggregate or a resin-modified glass ionomer used as a coronal barrier. J Endod. 2004. 30:782–784.


11. Song JS, Mante FK, Romanow WJ, Kim S. Chemical analysis of powder and set forms of Portland cement, gray ProRoot MTA, white ProRoot MTA, and gray MTA-Angelus. Oral Surg Oral Med Oral Pathol Oral Radiol Endod. 2006. 102:809–815.


12. Islam I, Chng HK, Yap AU. X-ray diffraction analysis of mineral trioxide aggregate and Portland cement. Int Endod J. 2006. 39:220–225.


13. Camilleri J, Montesin FE, Di Silvio L, Pitt Ford TR. The chemical constitution and biocompatibility of accelerated Portland cement for endodontic use. Int Endod J. 2005. 38:834–842.


14. De Deus G, Ximenes R, Gurgel-Filho ED, Plotkowski MC, Coutinho-Filho T. Cytotoxicity of MTA and Portland cement on human ECV 304 endothelial cells. Int Endod J. 2005. 38:604–609.


15. Ribeiro DA, Duarte MA, Matsumoto MA, Marques ME, Salvadori DM. Biocompatibility in vitro tests of mineral trioxide aggregate and regular and white Portland cements. J Endod. 2005. 31:605–607.


16. de Morais CA, Bernardineli N, Garcia RB, Duarte MA, Guerisoli DM. Evaluation of tissue response to MTA and Portland cement with iodoform. Oral Surg Oral Med Oral Pathol Oral Radiol Endod. 2006. 102:417–421.


17. Santos AD, Moraes JC, Araujo EB, Yukimitu K, Valerio Filho WV. Physico-chemical properties of MTA and a novel experimental cement. Int Endod J. 2005. 38:443–447.


18. Islam I, Chng HK, Yap AU. Comparison of the physical and mechanical properties of MTA and portland cement. J Endod. 2006. 32:193–197.


19. De-Deus G, Petruccelli V, Gurgel-Filho E, Coutinho-Filho T. MTA versus Portland cement as repair material for furcal perforations: a laboratory study using a polymicrobial leakage model. Int Endod J. 2006. 39:293–298.


20. Dammaschke T, Gerth HU, Zuchner H, Schafer E. Chemical and physical surface and bulk material characterization of white ProRoot MTA and two Portland cements. Dent Mater. 2005. 21:731–738.


21. Torabinejad M, Hong CU, McDonald F, Pitt Ford TR. Physical and chemical properties of a new root-end filling material. J Endod. 1995. 21:349–353.


22. Antunes Bortoluzzi E, Juarez Broon N, Antonio Hungaro Duarte M, de Oliveira Demarchi AC, Monteiro Bramante C. The use of a setting accelerator and its effect on pH and calcium ion release of mineral trioxide aggregate and white Portland cement. J Endod. 2006. 32:1194–1197.


23. Bortoluzzi EA, Broon NJ, Bramante CM, Garcia RB, de Moraes IG, Bernardineli N. Sealing ability of MTA and radiopaque Portland cement with or without calcium chloride for root-end filling. J Endod. 2006. 32:897–900.


24. Tay FR, Pashley DH, Rueggeberg FA, Loushine RJ, Weller RN. Calcium Phosphate Phase Transformation Produced by the Interaction of the Portland Cement Component of White Mineral Trioxide Aggregate with a Phosphate-containing Fluid. J Endod. 2007. 33:1347–1351.


25. Camilleri J, Montesin FE, Brady K, Sweeney R, Curtis RV, Ford TR. The constitution of mineral trioxide aggregate. Dent Mater. 2005. 21:297–303.


26. Camilleri J, Montesin FE, Curtis RV, Ford TR. Characterization of Portland cement for use as a dental restorative material. Dent Mater. 2006. 22:569–575.

