Abstract
Endurance exercise training such as marathon can increase the ability of exercise performance. Muscle glycogen is associated with an exercise performance, because glycogen depletion is primary causes of muscle fatigue. This review summarizes the glycogen saving effect according to duration of endurance exercise training. Long-term endurance exercise-induced mitochondrial biogenesis contributes to glycogen saving effect that is reduced glycogen breakdown and lactate accumulation. Glycogen sparing is due to a smaller decrease in adenosine triphosphate and phosphocreatine and a smaller increase in inorganic phosphate in the working muscles. It takes required endurance exercise training for about 4 weeks or more. Single bout or short-term endurance exercise is not sufficient to bring an increase in functional mitochondria. But peroxisome proliferator-activated receptor-γ coactivator-1α (PGC-1α) increases rapidly after single bout of endurance exercise. PGC-1α downregulates glycogenolytic and glycolytic enzymes to reduce muscle glycogen breakdown and lactic acid accumulation after short-term endurance exercise.
References
2. Spriet LL, Soderlund K, Bergstrom M, Hultman E. Skeletal muscle glycogenolysis, glycolysis, and pH during electrical stimulation in men. J Appl Physiol (1985). 1987; 62:616–21.


3. Karlsson J, Saltin B. Diet, muscle glycogen, and endurance performance. J Appl Physiol. 1971; 31:203–6.


5. Felig P, Cherif A, Minagawa A, Wahren J. Hypoglycemia during prolonged exercise in normal men. N Engl J Med. 1982; 306:895–900.


6. Hultman E, Bergstrom J. Muscle glycogen synthesis in relation to diet studied in normal subjects. Acta Med Scand. 1967; 182:109–17.


7. Oscai LB, Holloszy JO. Biochemical adaptations in muscle. II. Response of mitochondrial adenosine triphosphatase, creatine phosphokinase, and adenylate kinase activities in skeletal muscle to exercise. J Biol Chem. 1971; 246:6968–72.
8. Hoppeler H, Luthi P, Claassen H, Weibel ER, Howald H. The ultrastructure of the normal human skeletal muscle: a morphometric analysis on untrained men, women and well-trained orienteers. Pflugers Arch. 1973; 344:217–32.
9. Hawley JA. Adaptations of skeletal muscle to prolonged, intense endurance training. Clin Exp Pharmacol Physiol. 2002; 29:218–22.


10. Smiles WJ, Camera DM. More than mitochondrial biogenesis: alternative roles of PGC-1α in exercise adaptation. J Physiol. 2015; 593:2115–7.


11. Puigserver P, Wu Z, Park CW, Graves R, Wright M, Spiegelman BM. A cold-inducible coactivator of nuclear receptors linked to adaptive thermogenesis. Cell. 1998; 92:829–39.


12. Wu Z, Puigserver P, Andersson U, et al. Mechanisms controlling mitochondrial biogenesis and respiration through the thermogenic coactivator PGC-1. Cell. 1999; 98:115–24.


13. Baar K, Wende AR, Jones TE, et al. Adaptations of skeletal muscle to exercise: rapid increase in the transcriptional coactivator PGC-1. FASEB J. 2002; 16:1879–86.


14. Michael LF, Wu Z, Cheatham RB, et al. Restoration of insulin-sensitive glucose transporter (GLUT4) gene expression in muscle cells by the transcriptional coactivator PGC-1. Proc Natl Acad Sci U S A. 2001; 98:3820–5.


15. Wende AR, Schaeffer PJ, Parker GJ, et al. A role for the transcriptional coactivator PGC-1alpha in muscle refueling. J Biol Chem. 2007; 282:36642–51.
16. Kim SH, Koh JH, Higashida K, Jung SR, Holloszy JO, Han DH. PGC-1α mediates a rapid, exercise-induced downregulation of glycogenolysis in rat skeletal muscle. J Physiol. 2015; 593:635–43.


17. Hermansen L, Hultman E, Saltin B. Muscle glycogen during prolonged severe exercise. Acta Physiol Scand. 1967; 71:129–39.


18. Holloszy JO. Biochemical adaptations to exercise: aerobic metabolism. Exerc Sport Sci Rev. 1973; 1:45–71.
19. Fitts RH, Booth FW, Winder WW, Holloszy JO. Skeletal muscle respiratory capacity, endurance, and glycogen utilization. Am J Physiol. 1975; 228:1029–33.


20. Costill DL, Coyle E, Dalsky G, Evans W, Fink W, Hoopes D. Effects of elevated plasma FFA and insulin on muscle glycogen usage during exercise. J Appl Physiol Respir Environ Exerc Physiol. 1977; 43:695–9.


21. Cartee GD, Farrar RP. Exercise training induces glycogen sparing during exercise by old rats. J Appl Physiol (1985). 1988; 64:259–65.


22. Holloszy JO, Kohrt WM. Regulation of carbohydrate and fat metabolism during and after exercise. Annu Rev Nutr. 1996; 16:121–38.


23. Favier RJ, Constable SH, Chen M, Holloszy JO. Endurance exercise training reduces lactate production. J Appl Physiol (1985). 1986; 61:885–9.


24. Baldwin KM, Winder WW, Terjung RL, Holloszy JO. Glycolytic enzymes in different types of skeletal muscle: adaptation to exercise. Am J Physiol. 1973; 225:962–6.


25. Schantz P, Henriksson J, Jansson E. Adaptation of human skeletal muscle to endurance training of long duration. Clin Physiol. 1983; 3:141–51.


26. Holloszy JO, Coyle EF. Adaptations of skeletal muscle to endurance exercise and their metabolic consequences. J Appl Physiol Respir Environ Exerc Physiol. 1984; 56:831–8.


27. Coggan AR, Spina RJ, Kohrt WM, Holloszy JO. Effect of prolonged exercise on muscle citrate concentration before and after endurance training in men. Am J Physiol. 1993; 264:E215–20.


28. Holloszy JO. Biochemical adaptations in muscle: effects of exercise on mitochondrial oxygen uptake and respiratory enzyme activity in skeletal muscle. J Biol Chem. 1967; 242:2278–82.
29. Booth FW, Holloszy JO. Cytochrome c turnover in rat skeletal muscles. J Biol Chem. 1977; 252:416–9.


30. Pilegaard H, Keller C, Steensberg A, et al. Influence of pre-exercise muscle glycogen content on exercise-induced transcriptional regulation of metabolic genes. J Physiol. 2002; 541:261–71.


31. Jornayvaz FR, Shulman GI. Regulation of mitochondrial biogenesis. Essays Biochem. 2010; 47:69–84.


32. Hood DA. Invited review: contractile activity-induced mitochondrial biogenesis in skeletal muscle. J Appl Physiol (1985). 2001; 90:1137–57.
33. Higashida K, Kim SH, Higuchi M, Holloszy JO, Han DH. Normal adaptations to exercise despite protection against oxidative stress. Am J Physiol Endocrinol Metab. 2011; 301:E779–84.


34. Kim SH, Jung SR, Kim KJ. Role of NT-PGC-1α on endurance exercise induced mitochondiral biogenesis in rat skeletal muscle. Exerc Sci. 2012; 21:435–43.
35. Voet D, Voet JG, Pratt CW. Fundamentals of biochemistry: life at the molecularlevel. 2nd ed.New York: John Wiley and Sons;2006.
36. Kim SH, Kim KJ, Jung SR, Koh JH. How long days it have to mature mitochondrial function after repetitive bouts of prolonged endurance exercise training. Korean J Physic Educ. 2015; 54:377–83.
37. Booth FW, Kirby CR. Control of gene expression in adult skeletal muscle by changes in the inherent level of contractile activity. Biochem Soc Trans. 1991; 19:374–8.


38. Baldwin KM, Klinkerfuss GH, Terjung RL, Mole PA, Holloszy JO. Respiratory capacity of white, red, and intermediate muscle: adaptative response to exercise. Am J Physiol. 1972; 222:373–8.


39. Kelley DE, Mandarino LJ. Fuel selection in human skeletal muscle in insulin resistance: a reexamination. Diabetes. 2000; 49:677–83.


40. Ren JM, Hultman E. Regulation of glycogenolysis in human skeletal muscle. J Appl Physiol (1985). 1989; 67:2243–8.


41. Constable SH, Favier RJ, McLane JA, Fell RD, Chen M, Holloszy JO. Energy metabolism in contracting rat skeletal muscle: adaptation to exercise training. Am J Physiol. 1987; 253:C316–22.


42. Phillips SM, Green HJ, Tarnopolsky MA, Heigenhauser GJ, Grant SM. Progressive effect of endurance training on metabolic adaptations in working skeletal muscle. Am J Physiol. 1996; 270(2 Pt 1):E265–72.


43. Holloszy JO. Regulation of mitochondrial biogenesis and GLUT4 expression by exercise. Compr Physiol. 2011; 1:921–40.


44. Chakravarthy MV, Booth FW. Eating, exercise, and "thrifty" genotypes: connecting the dots toward an evolutionary understanding of modern chronic diseases. J Appl Physiol (1985). 2004; 96:3–10.


45. Costill DL, Bowers R, Branam G, Sparks K. Muscle glycogen utilization during prolonged exercise on successive days. J Appl Physiol. 1971; 31:834–8.


46. Kim SH, Ahn NY, Go JH, Kim KJ. Metabolic adaptation by acute and chronic endurance swim exercise in skeletal muscle. Exerc Sci. 2012; 21:331–8.
47. Terada S, Goto M, Kato M, Kawanaka K, Shimokawa T, Tabata I. Effects of low-intensity prolonged exercise on PGC-1 mRNA expression in rat epitrochlearis muscle. Biochem Biophys Res Commun. 2002; 296:350–4.


48. Wright DC, Han DH, Garcia-Roves PM, Geiger PC, Jones TE, Holloszy JO. Exercise-induced mitochondrial biogenesis begins before the increase in muscle PGC-1alpha expression. J Biol Chem. 2007; 282:194–9.
Fig. 1.
Glucose-fatty acid cycle (Randle cycle). G-6-P, glucose 6-phosphate; PFK, phosphofructokinase; PDH, pyruvate dehydrogenase.
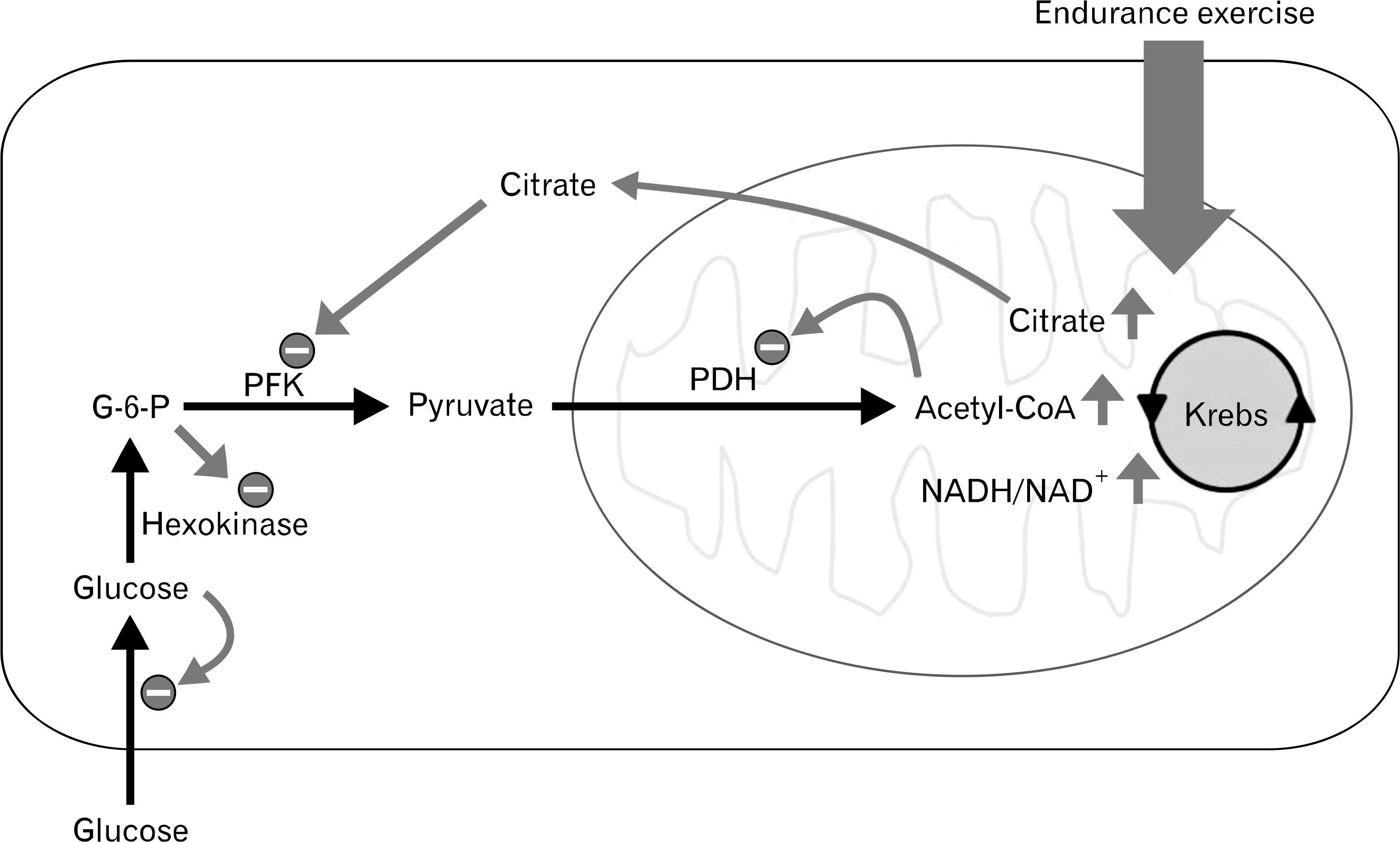
Fig. 2.
Inorganic phosphate (Pi) mediates the slowing of glycogenolysis and lactate production. G-6-P, glucose 6-phosphate; FFA, free fatty acids; ATP, adenosine triphosphate; ADP, adenosine diphosphate; PCr, phosphocreatine.
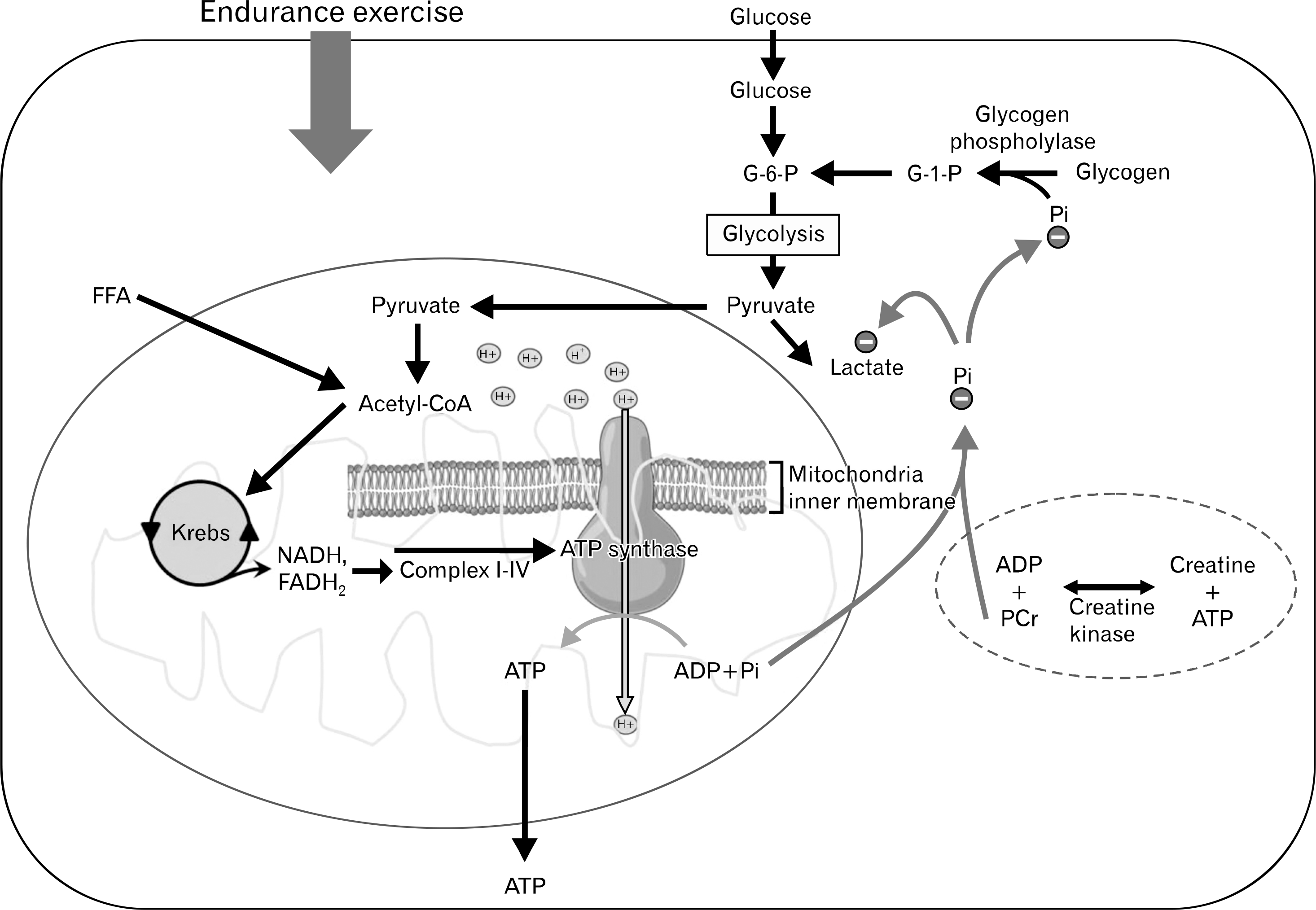
Fig. 3.
Peroxisome proliferator-activated receptor-γ coacti-vator-1α (PGC-1α) mediates downregulation of glycogenolytic and glycolytic enzymes to reduce glycogen breakdown and lactate production. Pi, Inorganic phosphate; ATP, adenosine triphosphate; ADP, adenosine diphosphate; G-1-P, glucose 1-phosphate; PFK, phosphofructokinase; GAPDH, glyceraldehyde 3-phosphate dehydrogenase; LDH, lactate dehydrogenase; PDH, pyruvate dehydrogenase.
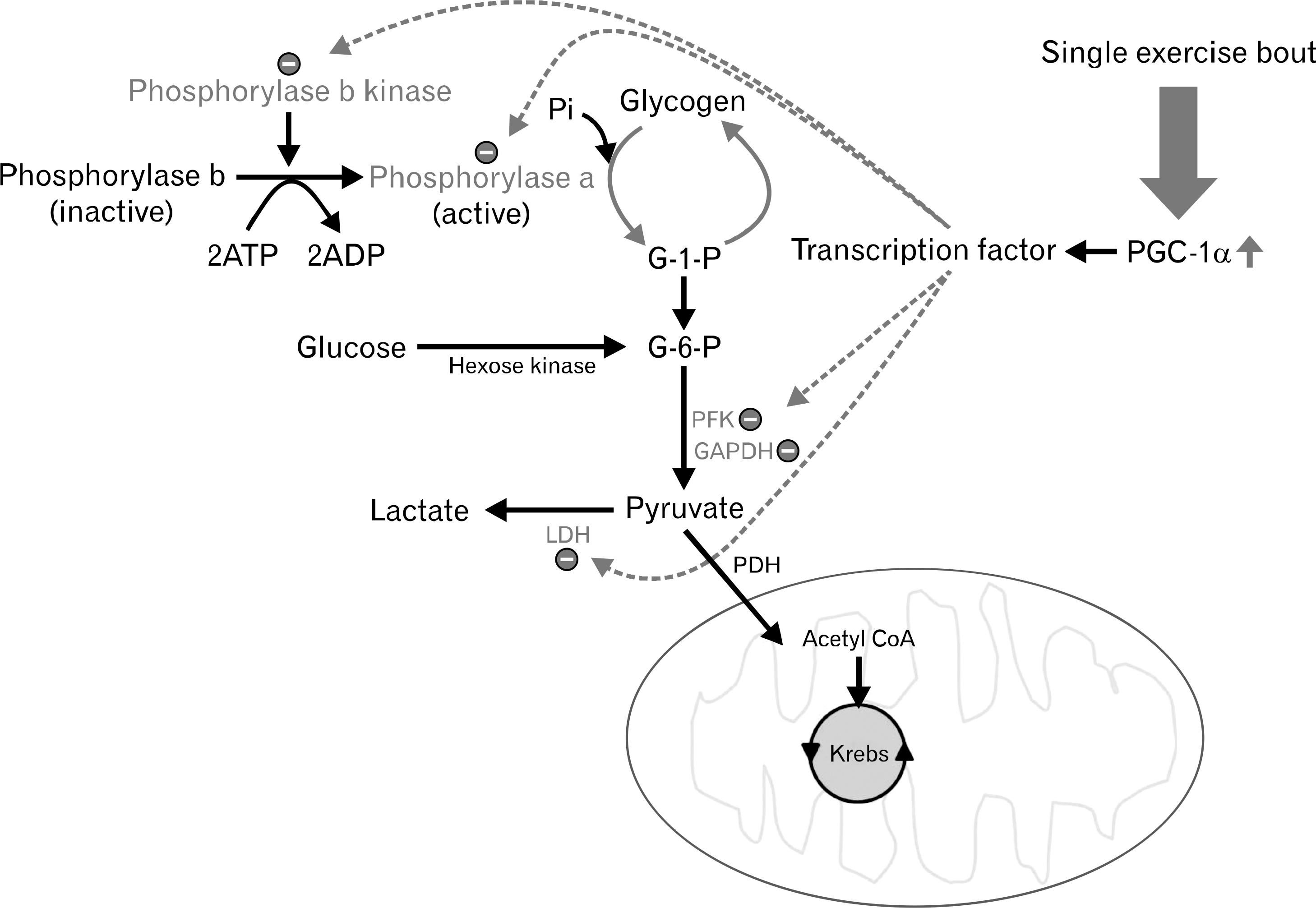