Abstract
PURPOSE
The aims of the study were to evaluate the fracture load of zirconia core material after dipping in coloring liquid at different time intervals and to compare the color of dipped blocks with that of prefabricated shaded blocks.
MATERIALS AND METHODS
3-unit bridge frameworks were designed digitally. Sixty frameworks were fabricated using uncolored zirconia blocks by CAD/CAM and divided into 4 groups randomly (n = 15). Group 2 (G2) was subjected to coloring liquids for 2 minutes, Group 4 (G4) for 4 minutes, and Group 6 (G6) for 6 minutes. CFS group was not subjected to any coloring procedure. After coloring, color differences between the test groups and a prefabricated shaded zirconia group (CPZ, n = 15) were evaluated by using a spectrophotometer. Fracture test was conducted immediately after shade evaluation with a Testometric test device at a cross-head speed of 1 mm/sec. Statistical analysis for evaluating color and fracture load was performed by using one way ANOVA followed by Tukey HSD test (P ≤ .05). Weibull analysis was conducted for distribution of fracture load.
RESULTS
There was no difference in terms of fracture load and color between CFS (1176.681 N) and G2 (985.638 N) group and between CPZ (81.340) and G2 (81.140) group, respectively. Fracture load values of G4 (779.340 N) and G6 (935.491 N) groups were statistically significantly lower than that of CFS group (P ≤ .005). The color values of G4 (79.340) and G6 (79.673) groups were statistically different than that of CPZ group (P ≤ .005).
The importance of esthetics of dental restorations have surpassed the mechanical advantages of metal-ceramic restorations.1 Thus, clinicians are forced to use all ceramic restorations in rehabilitations of patients even in the cases of implant retained fixed partial restorations.2 Since all ceramic restorations have relatively lower mechanical properties, the treatment options are very limited. To overcome this problem, manufacturers have introduced reinforced dental porcelains like lithium disilicate, aluminum oxide, zirconia (zirconium oxide) to expend treatment options.345 Among the new materials, zirconia has superior mechanical properties and is the material of choice for restorations especially in high stress bearing area.6 The increasing popularity of CAD/CAM technologies due to many advantages over conventional procedures allowed milled zirconia to be used in dental practice. High strength prefabricated zirconia blocks have been widely used for all ceramic restorations, which were milled using CAD/CAM Technologies.
The prefabricated zirconia blocks are white and chalk-like with very low or no translucency. Zirconia restorations are fabricated with zirconia core layered or pressed with feldspathic porcelain. It is well documented that this bi-layered structures were prone to mechanical failures like porcelain chipping.78910111213 Thus anatomically-contoured zirconia restorations without veneering porcelain have been introduced in dental practice to minimize mechanical failure.14 However, the elimination of layering porcelain inevitably resulted in decreased esthetic properties. Two main methods have been developed for coloring zirconia restorations to overcome the esthetic problem.15 In one of the methods, pigments are added to the zirconia powder before pressing the milling blocks. In this method, the color of the zirconia blocks is determined at the time of block fabrication and, hence, called pre-colored zirconia. In the other method, zirconia restorations are immersed in coloring liquids before sintering to achieve desired shade.16
The oxides like CeO2, Fe2O3, and Bi2O3 are added to the coloring liquids by the manufacturers to obtain different shades.17 The oxides penetrate into the pores of the milled, green zirconia and, after the sintering process, they give the restoration the desired shade. There are some studies in the literature about the effects of coloring liquids on the mechanical properties of zirconia. A study was published by Sedda et al.18 about the effect of coloring procedures on the flexural strength of zirconia blocks, claiming no negative effect in terms of the flexural characteristics of the tested zirconia. Pittayachawan et al.19 reported similar results, claiming color shading had no effect on the flexural strength of zirconia. However Shah et al.20 reported a significant decrease in flexural strength of zirconia after zirconia was subjected to coloring liquids. An interesting study reported by Hjerppe et al.17 stated that although there was a reduction in terms of flexural strength when zirconia was subjected to coloring liquids, some shades like D4 did not result in a significant reduction in flexural strength.
The literature about the effect of the coloring procedures on the mechanical properties of zirconia is limited and, obviously, there is no consensus about its effect. While there are a few studies in the literature regarding the effect of coloring liquids on the flexural strength, there is no study regarding how changes in dipping time affect the color of zirconia ceramics.
Therefore, the aims of the study were to evaluate the fracture load of zirconia after dipping in coloring liquids at different time intervals and to compare the color (E) of dipped blocks with that of zirconia precolored at industrial stage. The null hypothesis was that there would be no color difference between the prefabricated shaded blocks and the blocks shaded with liquids and that coloring liquids would have no effect on the fracture load of zirconia.
Using polyvinyl siloxane type A impression material (Elite HD, 232115, Zhermack, Ohlmuhle, Germany), an acrylic jaw (Ivoclar Vivadent, Schaan, Lichtenstein) containing a standard chamfer preparation was duplicated with plaster (Fujirock EP, 890222, GC, Chicago, IL, USA). The master base metal working model was made from the plaster duplicate model by conventional casting method (Fig. 1). A digital impression of the master model was taken using an intraoral scanner (Cerec Omnicam, Sirona Dental Systems, Bensheim, Germany). A 3-unit bridge framework was designed digitally with Cerec In Lab SW 15.0 with connectors of the dimensions 6 mm2 on both sides of the pontic. The connectors were circular and located at the central proximal areas. A small depression was also designed on the functional cusp of the pontic for housing the testometric indenter. A power analyses were conducted for determination of the number of test specimens in each group. Then, sixty zirconia frameworks were fabricated using uncolored zirconia blocks (Rainbow Zirconia Block, Genoss, Gyeonggido, Korea) by CAD/CAM system (Cerec InLab MCX5, Sirona Dental Systems, Bensheim, Germany) and divided into 4 groups randomly (n = 15). The first group (G2) was subjected to coloring liquid (Rainbow Coloring Liquid A2, Dentium, Gyeonggi-do, Korea) for 2 minutes, the second group (G4) for 4 minutes, and the third group (G6) for 6 minutes. The control group (CFS) was not subjected to any coloring procedures. The lot numbers of the zirconia blocks and the coloring liquid were listed in Table 1. After immersion in coloring liquid, test samples were fired in a zirconia sintering furnace (InFire HTC Speed, Sirona Dental Systems, Bensheim, Germany). The test samples were inspected for surface defects, and the connector dimensions were assured with a digital caliper (Mitutoyo Corp, Tokyo, Japan). For the comparison of color between precolored zirconia (Rainbow Shade Block A2, Genoss, Gyeonggi-do, Korea) and zirconia colored by immersion in coloring liquid, the same digital data 3 unit bridge frameworks were fabricated with precolored zirconia blocks as well (n = 15). This group was the control group (CPZ) for color comparisons. The coloring and sintering procedures were given in Table 2. After zirconia was subjected to coloring liquids, color and spectral distributions were evaluated by using Spectroshade Micro spectrophotometer (Version 2.4, MHT S.p.a, Verona, Italy). Commission Internationale de l'Eclairage(CIE) 1976 L*a*b* co-ordinates were recorded for color evaluation. L*a*b* coordinates were measured in five different areas of each specimen including the center, which was specifically designed flat on the occlusal surface of frameworks (Fig. 2). Average L* a* b* values of five measurements were used to calculate the color (E) of each test sample. The average E values of 15 specimens represented the color of the group. The color (E) of each test specimen was determined by the following equation.
Fracture test was conducted immediately after color evaluation with a universal testing device (M500-25KN; Testometric, Rochdale, UK) at a cross-head speed of 1 mm/sec. The test samples were cemented on the metal master model with a dual cure resin cement (Panavia V5, 000011, Kuraray Medical, Tokyo, Japan) prior to fracture test. The test samples were seated on the master model with finger pressure; excessive resin material was meticulously removed; an air barrier was applied; and light polymerization (Bluephase, Ivoclar Vivadent, Schaan, Liechtenstein) was performed at 450 mW/cm2 for 20 seconds on each side. Each fracture test was conducted on the same metal master model (Fig. 3).
Statistical analysis for evaluating color (E) and fracture load were performed by using one way ANOVA followed by Tukey HSD test (P ≤ .05). Weibull analysis was also conducted and Weibull modulus was calculated for each group using SPSS 21.0 (Fig. 4).
The means and standard deviations of test groups for color comparisons were given in Table 3. ANOVA analysis showed no color difference between CPZ and G2 groups (P ≥ .907). G4 and G6 showed statistically significant color differences from CPZ and G2 group (P ≤ .05) but showed no difference between them (P = .678).
The mean values of fracture load according to immersion time in coloring liquids were given in Table 4. According to ANOVA analysis, there was no statistically significant difference between CFS and G2 in terms of fracture load (P = .113). CFS group showed the highest fracture load compared to other groups (1176.681 N). G4 and G6 groups were statistically different from CFS and G2 group (P ≤ .05). Immersion of the test samples in coloring liquids for prolonged time negatively affected the fracture load of the test groups significantly (P ≤ .05). G4 groups showed the lowest fracture load (779.340 N); however, there was no significant difference between G4 and G6 groups (P = .274). The pre-colored zirconia group (CPZ) showed statistically lower fracture load than CFS, showing the values 875.203 N and 1176.681 N, respectively (P = .002); however, there was no significant difference between CPZ and G2 and between G4 and G6 test groups in terms of fracture load (P ≥ .05)
Since the fracture data was not normally distributed, Weibull Analysis was conducted. The highest Weibull modulus was observed for G6 group (7.16) followed by G2 (5.88), CFS (3.67), G4 (3.65), and CPZ (3.52) groups as shown in Fig. 4.
The current study was performed in order to evaluate changes in color and fracture load of zirconia material when subjected to coloring liquid before sintering. This has been of great importance since esthetics and long term success of the restoration are highly dependent on this procedure. The null hypothesis was that there would be no difference in terms of color between prefabricated shaded blocks and blocks shaded with liquids and that coloring liquids would have no effect on the fracture load of zirconia. In the study, the null hypothesis was rejected.
The results of the study revealed that the immersion of the zirconia to coloring liquids negatively affected the fracture load of the test specimens, emphasizing the importance of manufacturer's instructions. Although there was a decrease in fracture load when zirconia was subjected to coloring liquids for 2 minutes, which was the manufacturer's recommended time for coloring, the decrease was not statistically significant. The prolonged immersion time further decreased the fracture load of the test specimens. The results of the current study were in accordance with the study of Hjeroppe et al.,17 which reported a significant decrease in fracture strength as a result of prolonged coloring time. The studies about the effect of coloring liquids to the mechanical properties of zirconia emphasized the relationship between the inclusion of CeO2, Fe2O3, and Bi2O3 to the green zirconia and their effect on the mechanical properties.1721 Ardlin21 investigated the effects of coloring liquid on the mechanical properties of zirconia and hypothesized that the inclusion of CeO2, Fe2O3, and Bi2O3 increased the flexural strength of zirconia material. On the other hand, Wen et al.22 reported a 30% decrease in flexural strength when CeO2 and Er2O3 were added to zirconia. Sedda et al.18 and Shah et al.20 stated that by using coloring liquids at low concentration, it was possible to change the color of the zirconia to the desired shade without any significant change in mechanical properties. It seems that, by all means, using coloring liquid is very critical.
Prolonged coloring time influenced not only the fracture load but also the color of the zirconia material. In the current study, the color of the zirconia material after coloring procedures was compared to the colored zirconia at the industrial stage. The results revealed no difference between pre-colored zirconia and G2. However, when the immersion time was extended, the color of the zirconia was shifted from the desired shade. Vita shade A2 was chosen as the control color in the current study, as this color was the most common shade used in our clinic. Hjerppe et al.17 showed a reduction in fracture strength of zirconia, although not significant, when zirconia was subjected to D4 coloring liquid compared to the other shades; Still, further studies are needed for the mechanisms.
Various methods can be used for investigating the fracture strength of dental ceramics. Ball-on-ring test, piston on 3 ball test, and ring on ring test are preferred for testing the fracture strength of zirconia ceramics.23 All these test methods were well understood and preferred by researchers in their studies.24 However, the major drawback of the test methods was insufficiency in simulating clinical conditions. Disc shape ceramic test samples were located on different numbers of ball shape bearings according to test method and loaded at the center. Thus fracture strength can be calculated. However, loading the test specimens on ball supports induce significant friction stress as the supports cannot rotate during loading and the disc specimen loses its contact with the support. This may lead to premature failures in test specimens.23 Also, 3-point flexural strength test with a bar shape can be used for testing the strength of dental ceramics. However, flaws on the specimen edge concerns the reliability of the test. The 3-point bending test is largely dependent on the surface finish of the edges of the specimen. To eliminate edge failures and to simulate clinical conditions better, test specimens were prepared as 3-unit fixed partial dentures in the current study. The specimens were fixed with resin cement meticulously on the metal master model before fracture test. The margins of the restoration were checked with a brand new explorer for complete seating prior to curing. Although speculations may arise for the validity of the test method used in the current study, highly standard test samples were fabricated by using CAD/CAM. Cementation with resin cement stabilized the test specimen during fracture test, resulting in better simulation of clinical practice. Moreover, the fracture test was conducted with indenter of testometric located exactly on the same location of every test specimen by the depression prepared on the functional cusp for more standardized test assembly. Relatively higher standard deviations from fracture test in the study would be attributed to the brittle nature of the zirconia ceramics. The micro-cracks in zirconia ceramics might have caused unexpected failures, resulting in higher standard deviations irrespective of quality of test specimens.
The connectors are the weakest portions of the fixed partial dentures. Their dimensions should be adjusted for long-term survival of the restoration. There are studies suggesting various connector sizes primarily depending on the number of adjoining pontics in the restoration.25 Generally the higher the pontic number, the larger the size of the connector.26 In the current study, 6 mm2 circular connector was designed since this was the minimum connector dimensions for three unit fixed partial dentures recommended in literature.26
In several studies, it was shown that the fracture generally began at the gingival portion of the connector since tensile type stresses was occurred at this site.25 However, compression type stresses were occurred simultaneously on the opposite side of the connector. This might lead to failures in calculating fracture strength of the test specimens. Thus the author decided to use maximum fracture load in comparison. All the test samples in the current study were fractured from connector and fracture was initiated from gingival portion.
Since fracture data of brittle materials are not normally distributed, Weibull analysis is commonly used for strength data analysis of brittle materials.27 Lower Weibull modulus indicate more spread data and higher variability among measurements. A higher mean Weibull modulus for G6 group indicated a less spread distribution (lower variability) compared to the other groups with higher reliability. The Weibull modulus of the current study was in accordance with the study of Sedda et al.,18 which reported a higher Weibull value for colored specimens than for non-colored specimens and the lowest Weibull value for pre-colored zirconia specimens.
One limitation of the current study was that the test samples were not subjected to aging cycle prior to test procedures. Aging could change the results of the study. However, this would lead to complexity in analyzing the results, thus aging with thermal cycle was avoided.
Within the limitations of the current study, it was concluded that prolonged immersion of zirconia in A2 coloring liquids not only negatively affected the fracture load of the zirconia being tested in the current study but also affected the shade of the restoration. The study showed the importance of following manufacturer's instructions for coloring zirconia with coloring liquids. Clinical studies are needed for investigating the long-term color stability of the zirconia colored with coloring liquids.
Figures and Tables
Fig. 3
Testing of specimens with Testometric. The indenter was located on the depression of the functional cusp.
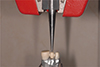
Table 1
Lot numbers of zirconia blocks and coloring liquid used in the study
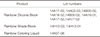
Product | Lot numbers |
---|---|
Rainbow Zirconia Block | 14A17-02, 14A02-02,14B05-02, 14A16-02,14B05-02, 14A16-01, 14A17-02,14A17-02 |
Rainbow Shade Block | 14H13-02, 14H13-01 |
Rainbow Coloring Liquid | 14K07-06 |
Table 2
The coloring and sintering procedures of test specimens
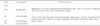
Table 3
The mean and standard deviation of color measurements
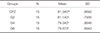
Groups | N | Mean | SD |
---|---|---|---|
CPZ | 15 | 81.340a* | .8692 |
G2 | 15 | 81.140a | .7935 |
G4 | 15 | 79.340b | .8846 |
G6 | 15 | 79.673b | .6943 |
References
1. Isgrò G, Pallav P, van der Zel JM, Feilzer AJ. The influence of the veneering porcelain and different surface treatments on the biaxial flexural strength of a heat-pressed ceramic. J Prosthet Dent. 2003; 90:465–473.
2. Cardelli P, Manobianco FP, Serafini N, Murmura G, Beuer F. Full-arch, Implant-supported monolithic zirconia rehabilitations: Pilot clinical evaluation of wear against natural or composite teeth. J Prosthodont. 2015; 10. 05.
3. O'Brien WJ. Dental materials and their selection. 3rd ed. Chicago: Quintessence;2002. p. 210–224.
4. Piconi C, Maccauro G. Zirconia as a ceramic biomaterial. Biomaterials. 1999; 20:1–25.
5. Guazzato M, Albakry M, Quach L, Swain MV. Influence of surface and heat treatments on the flexural strength of a glass-infiltrated alumina/zirconia-reinforced dental ceramic. Dent Mater. 2005; 21:454–463.
6. Guazzato M, Albakry M, Ringer SP, Swain MV. Strength, fracture toughness and microstructure of a selection of all-ceramic materials. Part II. Zirconia-based dental ceramics. Dent Mater. 2004; 20:449–456.
7. Denry I, Kelly JR. State of the art of zirconia for dental applications. Dent Mater. 2008; 24:299–307.
8. Kosmac T, Oblak C, Jevnikar P, Funduk N, Marion L. The effect of surface grinding and sandblasting on flexural strength and reliability of Y-TZP zirconia ceramic. Dent Mater. 1999; 15:426–433.
9. Hannink RHJ, Kelly PM, Muddle BC. Transformation toughening in zirconia-containing ceramics. J Am Ceram Soc. 2000; 83:461–487.
10. White SN, Miklus VG, McLaren EA, Lang LA, Caputo AA. Flexural strength of a layered zirconia and porcelain dental all-ceramic system. J Prosthet Dent. 2005; 94:125–131.
11. Sailer I, Fehér A, Filser F, Lüthy H, Gauckler LJ, Schärer P, Franz Hämmerle CH. Prospective clinical study of zirconia posterior fixed partial dentures: 3-year follow-up. Quintessence Int. 2006; 37:685–693.
12. Sailer I, Fehér A, Filser F, Gauckler LJ, Lüthy H, Hämmerle CH. Five-year clinical results of zirconia frameworks for posterior fixed partial dentures. Int J Prosthodont. 2007; 20:383–388.
13. Roediger M, Gersdorff N, Huels A, Rinke S. Prospective evaluation of zirconia posterior fixed partial dentures: fouryear clinical results. Int J Prosthodont. 2010; 23:141–148.
14. Lee WF, Feng SW, Lu YJ, Wu HJ, Peng PW. Effects of two surface finishes on the color of cemented and colored anatomic-contour zirconia crowns. J Prosthet Dent. 2016; 116:264–268.
15. Tuncel İ, Özat P, Eroğlu E. Effects of coloring procedures on zirconia/veneer ceramics bond strength. J Adv Prosthodont. 2014; 6:451–455.
16. Kim HK, Kim SH. Effect of the number of coloring liquid applications on the optical properties of monolithic zirconia. Dent Mater. 2014; 30:e229–e237.
17. Hjerppe J, Närhi T, Fröberg K, Vallittu PK, Lassila LV. Effect of shading the zirconia framework on biaxial strength and surface microhardness. Acta Odontol Scand. 2008; 66:262–267.
18. Sedda M, Vichi A, Carrabba M, Capperucci A, Louca C, Ferrari M. Influence of coloring procedure on flexural resistance of zirconia blocks. J Prosthet Dent. 2015; 114:98–102.
19. Pittayachawan P, McDonald A, Petrie A, Knowles JC. The biaxial flexural strength and fatigue property of Lava Y-TZP dental ceramic. Dent Mater. 2007; 23:1018–1029.
20. Shah K, Holloway JA, Denry IL. Effect of coloring with various metal oxides on the microstructure, color, and flexural strength of 3Y-TZP. J Biomed Mater Res B Appl Biomater. 2008; 87:329–337.
21. Ardlin BI. Transformation-toughened zirconia for dental inlays, crowns and bridges: chemical stability and effect of low-temperature aging on flexural strength and surface structure. Dent Mater. 2002; 18:590–595.
22. Wen N, Yi Y, Zhang W, Liu H, Tian J. Properties of dental alumina ceramics infiltrated with colored glass. Rare Metal Mater Eng. 2007; 36:82–84.
23. Shetty DK, Rosenfield AR, McGuire P, Duckworth WH. Biaxial flexure test for ceramics. American Ceram Soc Bulletin. 1980; 59:1193–1197.
24. Mijoska A, Popovska M. Evaluation of different in vitro testing methods for mechanical properties of veneer ceramics. Pril (Makedon Akad Nauk Umet Odd Med Nauki). 2015; 36:225–230.
25. Gargari M, Gloria F, Cappello A, Ottria L. Strength of zirconia fixed partial dentures: review of the literature. Oral Implantol (Rome). 2010; 3:15–24.
26. Studart AR, Filser F, Kocher P, Gauckler LJ. In vitro lifetime of dental ceramics under cyclic loading in water. Biomaterials. 2007; 28:2695–2705.
27. Quinn JB, Quinn GD. A practical and systematic review of Weibull statistics for reporting strengths of dental materials. Dent Mater. 2010; 26:135–147.