Abstract
PURPOSE
The purpose of this study was to investigate the in vitro cytotoxicity of thermoplastic denture base resins and to identify the possible adverse effects of these resins on oral keratinocytes in response to hot water/food intake.
MATERIALS AND METHODS
Six dental thermoplastic resin materials were evaluated: three polyamide materials (Smile tone, ST; Valplast, VP; and Luciton FRS, LF), two acrylic materials (Acrytone, AT; and Acryshot, AS), and one polypropylene resin material (Unigum, UG). One heat-polymerized acrylic resin (Vertex RS, RS) was chosen for comparison. After obtaining extracts from specimens of the denture resin materials (Φ=10 mm and d=2 mm) under different extraction conditions (37℃ for 24 hours, 70℃ for 24 hours, and 121℃ for 1 hour), the extracts (50%) or serial dilutions (25%, 12.5%, and 6.25%) in distilled water were co-cultured for 24 hours with immortalized human oral keratinocytes (IHOKs) or mouse fibroblasts (L929s) for the cytotoxicity assay described in ISO 10993.
Esthetics is important for patients receiving dental treatment and for those using removable partial dentures (RPDs). Therefore, esthetic demands must be considered to ensure patient satisfaction when using RPD clasps, which are conventionally constructed of metal to stabilize and retain RPDs in the oral cavity using the remaining teeth. In response to this demand, non-metal clasp dentures (NMCDs) were developed in the United States during the 1950s as an alternative; these dentures used polyamide, a thermoplastic denture resin product of the company Valplast. Since then, some RPDs using metal clasps have been replaced with RPDs using various thermoplastic polymers due to their flexibility and fracture resistance for esthetic reasons.12
Together with the aforementioned advantages, the lower elastic modulus and softer surface of the materials compared with acrylic resin render NMCDs more comfortable and lighter.345 In addition, NMCDs without metal compartments can eliminate concerns about metal allergies. Consequently, NMCDs with or without metal compartments (depending on patient indications) are used for patients who need interim or spare dentures to avoid metal allergies and for patients who place esthetics or comfort as their top priority.4
Among various types of thermoplastic polymers, polyamide, polypropylene, and thermoplastic acrylic resin have been widely used in commercially available NMCDs and are approved for use in clinical settings. Recently, NMCDs using polyethylene terephthalate, polycarbonate, and polyester have also been developed due to their enhanced mechanical properties compared with other thermoplastic polymers.4 Polyamide is used to synthesize nylons with repeating units that are linked by amide bonds.6 The thermoplastic polymer polypropylene is used in a wide variety of applications including packaging and the making of plastic parts and reusable equipment, due to its low melting point (~130℃) and consequent ease of use.7 Thermoplastic acrylic resin was developed to overcome the disadvantages of other thermoplastic polymers, specifically, the difficulty of treatments involving relining or repairs due to their weak bonding to self-curing resins and artificial teeth.8
Many studies have been performed to evaluate the mechanical properties of these materials, including flexural strength, elastic modulus, hardness and impact strength.491011 Color stability and water resorption were also evaluated because these properties have long-term effects on the esthetics, unfavorable odor generation, and dimensional instability of NMCDs.12 Few studies have examined biocompatibility due to the acceptable biocompatibility of NMCDs in clinical settings. Researchers have evaluated short-term and long-term cytotoxicity using indirect or direct contact tests involving L929s (mouse fibroblasts) or human amino fibroblasts.121314 However, cytotoxicity has not been tested under high-temperature conditions, which would reflect the exposure of NMCDs in the oral cavity to hot foods and beverages, using human oral keratinocytes, which are indirectly or directly affected by NMCDs as a major cell type of the outer epithelium of oral mucosa. In addition, according to ISO 7405 for the evaluation of the biocompatibility of medical devices used in dentistry and ISO 10993 for the biological evaluation of medical devices, 37, 50, 70, or 121℃ are suggested for extraction conditions on the basis of clinical applications and an appropriate exaggeration of product use.1516 In this study, 37℃ for 24 hours, 70℃ for 24 hours, and 121℃ for 1 hour were chosen as extraction conditions to mimic body temperature, hot food/beverage temperature, and a highly exaggerated thermal condition around/over the glass-transition temperature (Tg) of NMCDs.1517
In this study, cytotoxicity tests were performed using immortalized human oral keratinocytes (IHOKs) to identify the possible cytotoxicity of thermoplastic denture base resins that are used in NMCDs to oral mucosa under exposure to hot liquid/food uptake. L929 cells, which are suggested by the ISO standard for use in cytotoxicity tests, were also used to ensure the accuracy of the cytotoxicity results.18 The null hypothesis in this study was that the effects of the tested ‘thermoplastic polymers for NMCDs’ on cell viability are not influenced by the incubation temperature (37, 70, and 121℃).
In this study, the following four types of NMCD products were used: 1) polyamide resin-based products: Smile tone (ST, Korea Engineering Plastics Co., Seoul, Korea), Valplast (VP, Valplast) and Luciton FRS (LF, Dentsply Trubyte); 2) thermoplastic acrylic resin-based products: Acrytone (AT, High Dental) and Acryshot (AS, High Dental); 3) polypropylene-based products: Unigum (UG, Weldenz); and 4) conventional heat-polymerized acrylic resin-based products: Vertex RS (RS, Vertex). Vertex was selected to represent conventional heat-polymerized acrylic resin products for comparison with the NMCD products. Detailed information regarding these materials is summarized in Table 1.
Thermoplastic disc specimens (diameter, 10 mm; thickness, 2 mm) were prepared according to the methods and procedures specified by the manufacturers. Details of the drying/melting time, drying/melting temperature, and injection pressure are summarized in Table 1. The heat-polymerized resin was fabricated by mixing 21.5 g of powder with 10 mL of liquid and heat-polymerizing under the conditions recommended by the manufacturer. All specimens were polished using 220- to 2400-grit SiC sandpaper to obtain a highly polished surface, which is required for the denture resin outer surface to inhibit bacterial attachment and discoloration. The expiration dates of all samples were examined, and the samples were stored according to the manufacturers' recommendations throughout the experiment. Prior to in vitro cytotoxicity tests, the specimens were sterilized according to the manufacturer's suggested protocol for 5 hour under 1.0 kgf/cm2 using an ethylene oxide sterilization machine (PERSON-EO50; Person Medical, Gunpo, Korea) and gas comprising 20% ethylene oxide and 80% CO2, followed by exposure to air for 48 hours to eliminate any remaining gas.19
Extracts were obtained at a ratio of 3 cm2/mL following ISO 10339-12 recommendations.15 The specimen surface area was 2.2 cm2; therefore, the samples were immersed in 0.733 mL of distilled water (DW). To prepare each extract, three specimens were extracted into a total of 2.2 mL of DW. Extracts were divided into three groups according to the incubation temperature. The specimens immersed in DW were incubated for 24 hours at 37℃ or 70℃. The specimens exposed to high temperature were autoclaved at 121℃ for 1 hour (3041 VD autoclave, Shinhung, Korea). All procedures were performed on a clean bench to prevent contamination. The collected extracts were filtered using sterile syringe filters (0.20 µm, Corning, Corning, NY, USA). Extracts were obtained from freshly fabricated specimens for each of the following cytotoxicity tests with triplicate experiments.
IHOKs (55 – 60 passages), which are oral gingival keratinocytes that have been immortalized by human papillomavirus and confirmed to express epithelial markers over 350 passages,20 and L929 mouse fibroblast cells (5 – 10 passages) from (USA) were cultured in DMEM/F-12 3:1 mixture (Welgene, Daegu, Korea) and RPMI 1640 (Welgene), respectively, containing 10% fetal bovine serum (Gibco, Carlsbad, CA, USA) and 1% penicillin/streptomycin (Invitrogen, Carlsbad, CA, USA). The cells were incubated under a humidified atmosphere containing 5% CO2 at 37℃ during the experiments.
Cell viability tests were performed according to ISO 10093-5.18 Briefly, 100 µL of cell suspension (density 1 × 105 cells/mL) in supplemented medium was added to each well of a 96-well plate (SPL Life Sciences, Pocheon, Gyeonggi-do, Korea) for 24 hours. After washing with PBS, the cells were exposed to the original extract or serial dilutions of the extract in extract vehicle (DW) containing 2X supplemented medium. The final volume percentages of the extracts in the culture medium were 50%, 25%, 12.5%, and 6.25%. A mixture of 50 µL of medium and 50 µL of 2× supplemented medium was used as a blank control and exhibited 100% cell viability. Phenol (Sigma; 1% in DW) was used as a positive control to confirm the effectiveness of the cytotoxicity test.
Cell viability was assessed using the MTS assay (CellTiter 96 Aqueous One Solution Cell Proliferation Assay, Promega, Madison, WI) according to the manufacturer's protocol, and the results were expressed as the optical density percentage of each test group compared with each blank control group (n = 6). Sample size (n = 6) was determined to minimize the cell culture time (24 hours) gap among differently diluted groups in each test product to remove any cell culture time-induced bias, along with other considerations from the literature.2122 In addition, to check the repeatability of the results, triplicate analyses were performed independently. Optical absorbance was measured using a microplate reader (SpectraMax M2e, Molecular Devices, Sunnyvale, CA, USA) at a wavelength of 490 nm, normalized to control (0%) and expressed as a percentage, which was shown as cell viability. To confirm the cell viability tests, live/dead analysis was performed via confocal microscopy (Zeiss LSM700, Carl Zeiss, Thornwood, NY, USA). After washing with PBS, calcein AM (0.5 µM) and an ethidium homodimer-1 (4 µM) (Molecular Probes, Eugene, OR, USA) were simultaneously added and incubated for 30 miniutes to stain live (green, Ex/Em = 494/517 nm) and dead (red, Ex/Em = 517/617 nm) cells. All analyses were independently performed in triplicate to confirm the repeatability of the results, and representative means ± standard deviations or images are shown.
Measurements were performed according to the manufacturer's instructions (DSC 8231, Rigaku, Japan). After specimens were dried in a vacuum chamber and ground up, powder (8 ± 0.8 mg) from each specimen was heated from - 50℃ to 300℃ at a rate of 10℃/min under 100% N2 gas. To obtain precise Tg values, after primary heating to 300℃ and cooling to room temperature, the Tg value was obtained as the inflection point in the heat flow (in mW) and time (in min) curve from the secondary heating process, which was automatically calculated using the installed software. Tg values from 20℃ to 300℃ only were determined.
The data obtained in this study were analyzed using SPSS PASW version 23.0 (SPSS Inc., Chicago, IL, USA). Statistical analysis was performed using one-way ANOVA; Tukey's method was also used. Results were considered statistically significant at P < .05.
The MTS cytotoxicity assay results are shown in panel (a) of Figs. 1, 2, 3, 4, 5, 6, 7. In this study, the toxic effects on IHOKs were determined for six thermoplastic denture base resins and one type of heat-polymerized acrylic resin under three extraction conditions: 37℃, 70℃, and 121℃. Overall, cell viability was greater than 70% in all groups and under all extraction conditions tested. However, in the 50% extract co-culture condition, VP extracts prepared at 70℃ and AT extracts prepared at 121℃ resulted in significantly lower cell viability than in the control (0% extract, P < .05). The cell viabilities measured for the 25, 12.5, and 6.25% extracts of all tested samples were not significantly different from those measured for the control (0% extract, P > .05). RS, a heat-polymerized acrylic resin, did not exhibit any cytotoxicity compared with the control under all extraction conditions and at all incubation percentages (Fig. 7A).
The cytotoxicity test results were confirmed by visualizing live and dead cells after incubation with the 50% extracts. The results are shown in panels (b) to (e) of Figs. 1, 2, 3, 4, 5, 6, 7. Viable cells (green) mostly appeared without dead cells (red). VP extracted at 70℃ and AT extracted at 121℃ presented different numbers of cells compared with the control. The other extracts showed similar numbers of live cells to those of the control. In terms of the cell shapes in response to the different temperature condition, no obvious differences were observed.
The cytotoxicity test results obtained using L929s are shown in Table 2. Cell viability was greater than 70% in all groups under all tested extraction conditions. However, under the 50% extract co-culture condition, LF extracts prepared at 37℃, VP at 70℃, and AT at 121℃ showed significantly lower cell viability than the control (0% extract, P < .05). Interestingly, the 50%, 37℃ LF extract yielded 72.7 ± 4.3% cell viability. The cell viabilities observed for the 25%, 12.5%, and 6.25% sample extracts under all conditions tested were not significantly different compared with the control, with the exception of the 25% LF extract at 37℃ (0% extract, P > .05).
Tg was detected in all groups, ranging from 104℃ to 170℃. AT had the lowest Tg (104℃), while VP had the highest (170℃) among the tested groups. The remaining Tg values are listed in ascending order: AS (115℃), RS (119℃), UG (125℃), LF (138℃), and ST (150℃).
Cytotoxicity testing is a fundamental step in evaluating the biocompatibility of dental materials because it minimizes the need for in vivo or human tests.1823 In this experiment, cytotoxicity tests were performed using extracts prepared from three types of thermoplastic polymers used in NMCDs and approved by the national food and drug administration. Six commercial thermoplastic polymer materials and a conventional heat-polymerized acrylic resin were extracted under the following three conditions: 37℃ for 24 hours, 70℃ for 24 hours, and 121℃ for 1 hour. These temperatures and incubation times followed conditions suggested in ISO 10993-12.15
The choice of extraction conditions was based on clinical practice with an appropriate exaggeration of product use. Generally, extraction at 37℃ for 24 hours in an appropriate vehicle (DW or culture medium) is acceptable for cytotoxicity testing of dental materials because, when positioned in the oral cavity, denture resins are generally exposed to body temperature (37℃). Increased temperature can be used to mimic clinical use because extraction is a complex process that is influenced by temperature and other factors such as time, the surface-area-to-volume ratio, the extraction vehicle used and the phase equilibrium of the material.2425 Generally, increased temperature can cause the degradation or release of products that are not found in the final product under normal use conditions.18 Therefore, exposing the resin material to hot food or drink during eating can accelerate the extraction of various substances. Therefore, a temperature of 70℃ was selected to simulate the above clinical settings. Although the hot environment provided by a temperature of 121℃ is not realistic in the oral cavity, the ISO specification for medical devices demands a high-temperature condition to evaluate the Tg of polymer materials, which indicates the starting point of increased polymer chain mobility, the diffusion rate in the phase, and the consequent release of toxic chemicals.15 The Tg values of polymers for NMCDs vary from 0℃ to 105℃ depending on the polymer types and their networks.17 Therefore, the extraction condition of 121℃ for 1 hour was included as an initial test.
During the development of cytotoxicity tests for use with dental materials, many attempts have been made to mimic or properly accelerate the conditions to which these materials are exposed in the oral cavity in clinical practice. The cytotoxicity levels of elastomeric impression materials, root canal sealers, resin adhesives, and resin-reinforced glass ionomer cements have been evaluated during and after the setting of dental materials, and the results all showed higher cytotoxicity during setting/polymerization than with set/polymerized materials.26272829 Different extraction temperatures have also been used to mimic or properly accelerate clinical conditions, resulting in different cytotoxicities for polymers, dental alloys, commercially pure titanium, and latex gloves, which were used as a positive control in cytotoxicity tests.3031 The cytotoxicity of nanoparticles was also evaluated to determine their expected biocompatibility at different temperatures, and in vitro cell viability differed depending on the extraction temperature.32
Overall, the null hypothesis that the effects of polymers for NMCDs on cell viability do not depend on the incubation temperature (37, 70, and 121℃) was rejected based on the significantly different (lower) cell viability in some tested thermoplastic denture base resin extracts from different incubation conditions. However, all of the studied thermoplastic denture base resin extracts prepared under all studied extraction conditions resulted in greater than 70% cell viability when assayed against IHOKs and L929s; 70% cell viability is the reference point for cytotoxicity according to ISO 10993-5.18 Therefore, the six different thermoplastic denture base resin products used in NMCDs were not cytotoxic according to the ISO standard. By contrast, extracts of a representative conventional heat-polymerized acrylic resin (RS) resulted in approximately 100% cell viability for both cell lines, consistent with previously reported cytotoxicity results for heat-polymerizing acrylic resins.3334
However, the cytotoxicity of thermoplastic denture base resin cannot be ignored because the cell viability results from VP and AT were dependent on the extraction temperature. There were no differences in cell viability compared with the control (0% extract) when IHOKs were cultured with extracts prepared at 37℃. However, culturing IHOKs with extracts that were prepared at 70℃ (VP) and 121℃ (AT) resulted in significantly lower cell viability than that of the control (0% extract, P < .05), as confirmed by analyzing live and dead cells. Similarly, the cell viability results for L929s differed depending on the extraction temperature. Extracts prepared from VP at 70℃ and AT at 121℃ resulted in a significant loss of cell viability. Consequently, because the viability of both cell lines was compromised by extracts produced at high temperatures in VP (70℃) and AT (121℃), some thermoplastic denture resins may pose safety concerns, including allergic reactions, to the oral mucosa by releasing chemicals when exposed to temperature-accelerated clinical conditions such as those experienced during hot food and beverage intake.
All three types of six thermoplastic denture resin base products used in NMCDs showed no or acceptable cytotoxicity when extracted at different temperatures. Among the three tested types of thermoplastic polymers, the polyamide product (VP) and the polymethyl methacrylate (AT) compromised the cell viability of IHOKs after high-temperature incubation. The decrease in cell viability might be partially explained by Tg. Tg indicates the starting point of increased polymer chain mobility, the diffusion rate in the phase, and the consequent release of toxic chemicals.17 The Tg values of polypropylene, polyamide, and polymethyl methacrylate are 0–10, 47 – 60, and 105℃, respectively, according to the chemical library.35 However, along with copolymerization with other polymers and polymer crystallization, Tg was differently determined in thermoplastic resins (104 – 170℃) by DSC. Interestingly, AT, which only significantly compromised cell viability in both IHOKs and L929s with the extract at 121℃, had the lowest Tg (104℃) among the resins. However, AS and RS, which also had Tg values under 121℃ (115 – 119℃), did not compromise cell viability, possibly because of the short incubation time (1 hour) at 121℃. ST, VP, LF, and UG, which showed Tg values over 121℃ (125 – 170℃), sustained cell viability with the extract at 121℃. The dependence of cytotoxicity on the Tg value will be studied further under more accelerated conditions using 121℃ extraction for 24 hour to further clarify these observations. For VP, only the extract at 70℃, which is below the Tg of VP (170℃), resulted in compromised cell viability in both cell lines, which cannot be explained by Tg. Toxic chemicals may have been released under high thermal conditions at 70℃ for 24 hour regardless of Tg. However, VP extraction at 121℃ for 1 hour might not be enough to release toxic chemicals due to the short incubation time or may render toxic components non-toxic due to further polymerizations or chemical interactions.1836 Significantly lower L929s viability in LF only at 37℃, and not at 70 and 121℃, may also be explained by the above chemical transformations.
To date, the extractable (releasable) cytotoxic component in thermoplastic denture resin has not been identified. Because thermoplastic resins are fabricated by addition polymerization involving long linear chains held together by weak van der Waals forces, the long linear chains can move freely at high temperature without experiencing degradation. However, conventional heat-polymerized acrylic resins are three-dimensional networks that are generated by condensation polymerization and thus degrade at high temperatures. Therefore, thermoplastic polymers are considered a more biocompatible biomaterial (compared with conventional heat-polymerized acrylic resin) due to their basic structure and the polymerization method used (e.g., addition polymerization). The present study is the first to reveal the possible cytotoxicity of thermoplastic denture resin at high temperatures (especially over the Tg). Further analysis of the cytotoxic chemicals in the extracts at high temperature is needed, along with the detection of toxic substances from polymerized denture resins that can cause toxic reactions, irritation, inflammation, or allergic responses in oral tissues.37
In addition, long-term accelerated cytotoxicity (or biocompatibility) tests are necessary for thermoplastic polymers to further reveal the clinical safety of each polymer at high temperature using thermocycling conditions (5 to 55℃) or long-term (24 hour) incubation above the Tg. Cytotoxicity tests using independent cell lines, such as those performed in this study, cannot fully simulate the complex immune response and reaction of the oral mucosa, which comprises multiple cell types and extracellular matrix; this is one limitation of this study. Therefore, additional in vivo (hamster cheek pouch model) or clinical studies are required to predict clinical responses, although acceptable cytotoxicity with minor concerns was observed under high-temperature extraction.38
Interestingly, extracts prepared from LF at 37℃ resulted in a significant loss of cell viability only in L929s but not in IHOKs. The results of the cytotoxicity tests depend on the cell types used and their origins.3940 In international standards, established cell lines such as L929s are preferred for obtaining reliable cytotoxicity results; therefore, L929s cells were also used in this study regardless of their mismatched origin and cell type.1841 After consideration of the clinical application of denture resins, IHOKs were primarily studied to investigate cytotoxicity against the oral mucosa. When tissue-specific sensitivity is required, the use of primary cultured or immortalized cells derived from target tissues is recommended. In this study, IHOKs originating from human oral mucosa were used for cytotoxicity tests due to their easy maintenance and biological similarity to primary cultured oral keratinocytes.42 The use of human cells instead of other mammalian cells is also required for cytotoxicity investigations due to their differing biological responses to toxic components.4344 Other cytotoxicity evaluation studies using different cell types (cell origins) with the same dental materials have reported discrepancies in cytotoxicity results.4546 The permeability of cell membranes, intracellular availability, and extracellular environmental interactions with the various components released from the tested materials might result in considerable variations in the cytotoxicity results.45 According to previous reports, the cell viabilities of IHOKs and L929s differ depending on the released chemical types.2147 Therefore, even though LF at 37℃ resulted in significantly lower cell viability in L929s, the cytotoxicity of thermoplastic denture resins against oral cells may be limited, as evidenced by the nearly 100% cell viability of IHOKs exposed to LF at 37℃.
We used polished specimens because extracts from polished surfaces affect the oral mucosa when dentures are placed in the oral cavity. Direct cytotoxicity testing using assays, such as the agar diffusion test and the artificial oral mucosa barrier test, is necessary to evaluate the interaction between the inner surface of unpolished thermoplastic dentures and the oral mucosa.48 Studies of the cytotoxicity of thermoplastic denture resins have revealed negligible influences on the viability (90 – 100%) of human gingival or amnion fibroblasts before or after polishing; consequently, these materials are considered non-cytotoxic.1213 However, when thermoplastic denture base resins used in NMCDs were aged in artificial saliva for long periods without polishing, they showed severe cytotoxicity (as low as 40% cell viability), as did conventional acrylic resin; these findings reveal the potential risks of aged denture resin materials to oral tissue, regardless of the thermoplastic polymer type.13 Although the present study showed that the materials from polished specimens were biocompatible (greater than 70% cell viability) at all incubation temperatures with positive (0.1% phenol, 5 ± 0.8% cell viability) and negative controls (0% extract, 100% cell viability), in vivo and clinical studies are necessary to ensure the biocompatibility of thermoplastic denture resins when exposed to high temperatures.
Within the limitations of this study, we can conclude that severe cytotoxicity (less than 70%) was not detected in any tested thermoplastic denture resin bases used in NMCDs when IHOKs and L929s were subjected to extracts obtained after incubation at different temperatures (37℃, 70℃, and 121℃). However, compromised IHOK viability was detected in some thermoplastic resins following incubation at high temperatures (70 and 121℃). Therefore, to support the safe use of these materials in clinical situations under various temperatures (especially high temperature), additional in vitro or preclinical animal studies are needed to verify long-term oral biocompatibility.
Figures and Tables
Fig. 1
Cell viability results (A) and confocal microscopy images of live and dead cells (B to E) for thermoplastic Smiletone (ST) according to extraction condition (37℃ for 24 hours, 70℃ for 24 hours, and 121℃ for 1 hour) and extract percentage in co-culture with IHOKs (0, 6.25, 12.5, 25, and 50%). Different letters indicate significant differences among the extraction conditions (P < .05). Live cells (green) and dead cells (red) were observed by confocal microscopy. The red line indicates 70% cell viability. Representative means ± standard deviation (n = 6) and images are shown; the experiments were independently performed in triplicate.
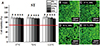
Fig. 2
Cell viability results (A) and confocal microscopy images of live and dead cells (B to E) for thermoplastic Valplast (VP) according to extraction condition (37℃ for 24 hours, 70℃ for 24 hours, and 121℃ for 1 hour) and extract percentage in co-culture with IHOKs (0, 6.25, 12.5, 25, and 50%). Different letters indicate significant differences among the extraction conditions (P < .05). Live cells (green) and dead cells (red) were observed by confocal microscopy. The red line indicates 70% cell viability. Representative means ± standard deviation (n = 6) and images are shown; the experiments were independently performed in triplicate.
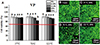
Fig. 3
Cell viability results (A) and confocal microscopy images of live and dead cells (B to E) for thermoplastic Luciton FRS (LF) according to extraction condition (37℃ for 24 hours, 70℃ for 24 hours, and 121℃ for 1 hour) and extract percentage in co-culture with IHOKs (0, 6.25, 12.5, 25, and 50%). Different letters indicate significant differences among the extraction conditions (P < .05). Live cells (green) and dead cells (red) were observed by confocal microscopy. The red line indicates 70% cell viability. Representative means ± standard deviation (n = 6) and images are shown; the experiments were independently performed in triplicate.
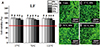
Fig. 4
Cell viability results (A) and confocal microscopy images of live and dead cells (B to E) for thermoplastic Acrytone (AT) according to extraction condition (37℃ for 24 hours, 70℃ for 24 hours, and 121℃ for 1 hour) and extract percentage in co-culture with IHOKs (0, 6.25, 12.5, 25, and 50%). Different letters indicate significant differences among the extraction conditions (P < .05). Live cells (green) and dead cells (red) were observed by confocal microscopy. The red line indicates 70% cell viability. Representative means ± standard deviation (n = 6) and images are shown; the experiments were independently performed in triplicate.
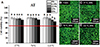
Fig. 5
Cell viability results (A) and confocal microscopy images of live and dead cells (B to E) for thermoplastic Acryshot (AS) according to extraction condition (37℃ for 24 hours, 70℃ for 24 hours, and 121℃ for 1 hour) and extract percentage in co-culture with IHOKs (0, 6.25, 12.5, 25, and 50%). Different letters indicate significant differences among the extraction conditions (P < .05). Live cells (green) and dead cells (red) were observed by confocal microscopy. The red line indicates 70% cell viability. Representative means ± standard deviation (n = 6) and images are shown; the experiments were independently performed in triplicate.
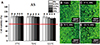
Fig. 6
Cell viability results (A) and confocal microscopy images of live and dead cells (B to E) for thermoplastic Unigum (UG) according to extraction condition (37℃ for 24 hours, 70℃ for 24 hours, and 121℃ for 1 hour) and extract percentage in co-culture with IHOKs (0, 6.25, 12.5, 25, and 50%). Different letters indicate significant differences among the extraction conditions (P < .05). Live cells (green) and dead cells (red) were observed by confocal microscopy. The red line indicates 70% cell viability. Representative means ± standard deviation (n = 6) and images are shown; the experiments were independently performed in triplicate.
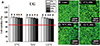
Fig. 7
Cell viability results (A) and confocal microscopy images of live and dead cells (B to E) for conventional heat-polymerized acrylic resin (Vertex RS: RS) according to extraction condition (37℃ for 24 hours, 70℃ for 24 hours, and 121℃ for 1 hour) and extract percentage in co-culture with IHOKs (0, 6.25, 12.5, 25, and 50%). Different letters indicate significant differences among the extraction conditions (P < .05). Live cells (green) and dead cells (red) were observed by confocal microscopy. The red line indicates 70% cell viability. Representative means ± standard deviation (n = 6) and images are shown; the experiments were independently performed in triplicate.
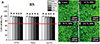
Table 1
Denture resin materials tested in this study and their manufacturing conditions
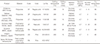
Table 2
Cell viability results for L929s after incubation with extracts prepared at different temperatures
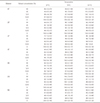
References
1. Sasaki H, Hamanaka I, Takahashi Y, Kawaguchi T. Effect of reinforcement on the flexural properties of injection-molded thermoplastic denture base resins. J Prosthodont. 2015; 12. 18.
2. Fueki K, Ohkubo C, Yatabe M, Arakawa I, Arita M, Ino S, Kanamori T, Kawai Y, Kawara M, Komiyama O, Suzuki T, Nagata K, Hosoki M, Masumi S, Yamauchi M, Aita H, Ono T, Kondo H, Tamaki K, Matsuka Y, Tsukasaki H, Fujisawa M, Baba K, Koyano K, Yatani H. Clinical application of removable partial dentures using thermoplastic resin. Part II: Material properties and clinical features of non-metal clasp dentures. J Prosthodont Res. 2014; 58:71–84.
3. Wada J, Fueki K, Yatabe M, Takahashi H, Wakabayashi N. A comparison of the fitting accuracy of thermoplastic denture base resins used in non-metal clasp dentures to a conventional heat-cured acrylic resin. Acta Odontol Scand. 2015; 73:33–37.
4. Fueki K, Ohkubo C, Yatabe M, Arakawa I, Arita M, Ino S, Kanamori T, Kawai Y, Kawara M, Komiyama O, Suzuki T, Nagata K, Hosoki M, Masumi S, Yamauchi M, Aita H, Ono T, Kondo H, Tamaki K, Matsuka Y, Tsukasaki H, Fujisawa M, Baba K, Koyano K, Yatani H. Clinical application of removable partial dentures using thermoplastic resin-part I: definition and indication of non-metal clasp dentures. J Prosthodont Res. 2014; 58:3–10.
5. Ucar Y, Akova T, Aysan I. Mechanical properties of polyamide versus different PMMA denture base materials. J Prosthodont. 2012; 21:173–176.
6. Basavaraj E, Ramaraj B, Lee JH. Polyamide 6/carbon black/molybdenum disulphide composites: Friction, wear and morphological characteristics. Mater Chem Phys. 2013; 138:658–665.
7. Xu X, He Z, Lu S, Guo D, Yu J. Enhanced thermal and mechanical properties of lignin/polypropylene wood-plastic composite by using flexible segment-containing reactive compatibilizer. Macromol Res. 2014; 22:1084–1089.
8. Kim JH, Choe HC, Son MK. Evaluation of adhesion of reline resins to the thermoplastic denture base resin for nonmetal clasp denture. Dent Mater J. 2014; 33:32–38.
9. Hamanaka I, Takahashi Y, Shimizu H. Mechanical properties of injection-molded thermoplastic denture base resins. Acta Odontol Scand. 2011; 69:75–79.
10. Takabayashi Y. Characteristics of denture thermoplastic resins for non-metal clasp dentures. Dent Mater J. 2010; 29:353–361.
11. Iwata Y. Assessment of clasp design and flexural properties of acrylic denture base materials for use in non-metal clasp dentures. J Prosthodont Res. 2016; 60:114–122.
12. Jang DE, Lee JY, Jang HS, Lee JJ, Son MK. Color stability, water sorption and cytotoxicity of thermoplastic acrylic resin for non metal clasp denture. J Adv Prosthodont. 2015; 7:278–287.
13. Uzun IH, Tatar A, Hacimuftuoglu A, Saruhan F, Bayindir F. In vitro evaluation of long-term cytotoxic response of injection-molded polyamide and polymethyle metacrylate denture base materials on primary fibroblast cell culture. Acta Odontol Scand. 2013; 71:1267–1272.
14. Sahin O, Ozdemir AK, Turgut M, Boztug A, Sumer Z. Investigation of flexural strength and cytotoxicity of acrylic resin copolymers by using different polymerization methods. J Adv Prosthodont. 2015; 7:98–107.
15. ISO 10993-12. Biological evaluation of medical devices-part 12: sample preparation and reference materials. 3rd ed. Geneva, Switzerland: International Organization for Standardization;2012.
16. ISO 7405. Dentistry-evaluation of biocompatibility of medical devices used in dentistry. 3rd ed. Geneva, Switzerland: International Organization for Standardization;2008.
17. Black J, Hastings G. Handbook of biomaterial properties. New York, NY: Springer Science & Business Media;2013.
18. ISO 10993-5. Biological evaluation of medical devices-part 5: tests for in vitro cytotoxicity. 3rd ed. Geneva, Switzerland: International Organization for Standardization;2009.
19. Lee JH, Kwon JS, Moon SK, Uhm SH, Choi BH, Joo UH, Kim KM, Kim KN. Titanium-silver alloy miniplates for mandibular fixation: In vitro and in vivo study. J Oral Maxillofac Surg. 2016; 74:1622.
20. Oda D, Bigler L, Lee P, Blanton R. HPV immortalization of human oral epithelial cells: a model for carcinogenesis. Exp Cell Res. 1996; 226:164–169.
21. Lee JH, Lee HH, Kim KN, Kim KM. Cytotoxicity and antiinflammatory effects of zinc ions and eugenol during setting of ZOE in immortalized human oral keratinocytes grown as three-dimensional spheroids. Dent Mater. 2016; 32:e93–e104.
22. Lee JH, Lee HH, Kim HW, Yu JW, Kim KN, Kim KM. Immunomodulatory/anti-inflammatory effect of ZOE-based dental materials. Dent Mater. 2017; 33:e1–e12.
23. Malkoç MA, Demİr N, Şengün A, Bozkurt ŞB, Hakki SS. Cytotoxicity of temporary cements on bovine dental pulp-derived cells (bDPCs) using realtime cell analysis. J Adv Prosthodont. 2015; 7:21–26.
24. Nishi C, Nakajima N, Ikada Y. In vitro evaluation of cytotoxicity of diepoxy compounds used for biomaterial modification. J Biomed Mater Res. 1995; 29:829–834.
25. Liu X, Xi T, Zheng Y. Influence of the extraction parameters on the cytotoxicity test results of Mg materials. Progress Natural Sci. 2014; 24:507–515.
26. dos Santos RL, Pithon MM, Martins FO, Romanos MT, Ruellas AC. Evaluation of cytotoxicity and degree of conversion of glass ionomer cements reinforced with resin. Eur J Orthod. 2012; 34:362–366.
27. Miletić I, Devcić N, Anić I, Borcić J, Karlović Z, Osmak M. The cytotoxicity of RoekoSeal and AH plus compared during different setting periods. J Endod. 2005; 31:307–309.
28. Cavalcanti BN, Rode SM, Marques MM. Cytotoxicity of substances leached or dissolved from pulp capping materials. Int Endod J. 2005; 38:505–509.
29. Kwon JS, Lee SB, Kim CK, Kim KN. Modified cytotoxicity evaluation of elastomeric impression materials while polymerizing with reduced exposure time. Acta Odontol Scand. 2012; 70:597–602.
30. Baek HS, Yoo JY, Rah DK, Han DW, Lee DH, Kwon OH, Park JC. Evaluation of the extraction method for the cytotoxicity testing of latex gloves. Yonsei Med J. 2005; 46:579–583.
31. Faria AC, Rodrigues RC, Antunes RP, de Mattos Mda G, Rosa AL, Ribeiro RF. Effect of temperature variation on the cytotoxicity of cast dental alloys and commercially pure titanium. J Appl Oral Sci. 2009; 17:421–426.
32. Das GK, Chan PP, Teo A, Loo JS, Anderson JM, Tan TT. In vitro cytotoxicity evaluation of biomedical nanoparticles and their extracts. J Biomed Mater Res A. 2010; 93:337–346.
33. Goiato MC, Freitas E, dos Santos D, de Medeiros R, Sonego M. Acrylic resin cytotoxicity for denture base-literature review. Adv Clin Exp Med. 2015; 24:679–686.
34. Hensten-Pettersen A, Wictorin L. The cytotoxic effect of denture base polymers. Acta Odontol Scand. 1981; 39:101–106.
35. Wilkes CE, Summers JW, Daniels CA, Berard MT. PVC handbook. Cincinnati, OH: Hanser Publications;2005.
36. Vihola H, Laukkanen A, Valtola L, Tenhu H, Hirvonen J. Cytotoxicity of thermosensitive polymers poly(N-isopropylacrylamide), poly(N-vinylcaprolactam) and amphiphilically modified poly(N-vinylcaprolactam). Biomaterials. 2005; 26:3055–3064.
37. Akin H, Tugut F, Polat ZA. In vitro comparison of the cytotoxicity and water sorption of two different denture base systems. J Prosthodont. 2015; 24:152–155.
38. Browne RM. The in vitro assessment of the cytotoxicity of dental materials-does it have a role? Int Endod J. 1988; 21:50–58.
39. O'Brien J, Wilson I, Orton T, Pognan F. Investigation of the Alamar Blue (resazurin) fluorescent dye for the assessment of mammalian cell cytotoxicity. Eur J Biochem. 2000; 267:5421–5426.
40. Coulter JA, Jain S, Butterworth KT, Taggart LE, Dickson GR, McMahon SJ, Hyland WB, Muir MF, Trainor C, Hounsell AR, O'Sullivan JM, Schettino G, Currell FJ, Hirst DG, Prise KM. Cell type-dependent uptake, localization, and cytotoxicity of 1.9 nm gold nanoparticles. Int J Nanomedicine. 2012; 7:2673–2685.
41. Borenfreund E, Puerner JA. Toxicity determined in vitro by morphological alterations and neutral red absorption. Toxicol Lett. 1985; 24:119–124.
42. Lee JH, Seo SH, Lee SB, Om JY, Kim KM, Kim KN. Cytotoxicity and terminal differentiation of human oral keratinocyte by indium ions from a silver-palladium-gold-indium dental alloy. Dent Mater. 2015; 31:123–133.
43. Lee TC, Ho IC. Differential cytotoxic effects of arsenic on human and animal cells. Environ Health Perspect. 1994; 102:101–105.
44. Lovschall H, Eiskjaer M, Arenholt-Bindslev D. Formaldehyde cytotoxicity in three human cell types assessed in three different assays. Toxicol In Vitro. 2002; 16:63–69.
45. Schweikl H, Schmalz G. Toxicity parameters for cytotoxicity testing of dental materials in two different mammalian cell lines. Eur J Oral Sci. 1996; 104:292–299.
46. Kwon JS, Illeperuma RP, Kim J, Kim KM, Kim KN. Cytotoxicity evaluation of zinc oxide-eugenol and non-eugenol cements using different fibroblast cell lines. Acta Odontol Scand. 2014; 72:64–70.
47. Chen F, Wu T, Cheng X. Cytotoxic effects of denture adhesives on primary human oral keratinocytes, fibroblasts and permanent L929 cell lines. Gerodontology. 2014; 31:4–10.
48. Wataha JC, Rueggeberg FA, Lapp CA, Lewis JB, Lockwood PE, Ergle JW, Mettenburg DJ. In vitro cytotoxicity of resincontaining restorative materials after aging in artificial saliva. Clin Oral Investig. 1999; 3:144–149.