Abstract
PURPOSE
To explore the influence of different surface conditionings on surface changes and the influence of surface treatments and aging on the bond strengths of composites to non-aged and aged resin nanoceramics.
MATERIALS AND METHODS
Rectangular-shaped non-aged and aged (5000 thermocycles) resin nanoceramic specimens (Lava Ultimate) (n=63, each) were divided into 3 groups according to surface treatments (untreated, air abrasion, or silica coating) (n=21). The surface roughness was measured and scanning electron microscopy was used to examine one specimen from each group. Afterwards, the specimens were repaired with a composite resin (Filtek Z550) and half were sent for aging (5000 thermocycles, n=10, each). Shear bond strengths and failure types were evaluated. Roughness and bond strength were investigated by two- and three-way analysis of variance, respectively. The correlation between the roughness and bond strength was investigated by Pearson's correlation test.
RESULTS
Surface-treated samples had higher roughness compared with the untreated specimens (P=.000). For the non-aged resin nanoceramic groups, aging was a significant factor for bond strength; for the aged resin nanoceramic groups, surface treatment and aging were significant factors. The failures were mostly adhesive after thermal cycling, except in the non-aged untreated group and the aged air-abraded group, which had mostly mixed failures. Roughness and bond strength were positively correlated (P=.003).
As computer-aided design and computer-aided manufacturing (CAD/CAM) technology has become more reliable and widespread in dentistry, an increasing amount of effort is being devoted to the development and marketing of millable restorative materials.1 The design and manufacturing of the dental restoration can be performed either in the dental laboratory or in the dental office.2
The earliest restorative material for CAD/CAM was dental ceramic. Generally, traditional ceramic blocks can produce esthetic restorations, and previous studies34 reported high fracture resistance. Despite the outstanding advantages of dental ceramics, the incidence of failure and potential abrasive effect on opposing dentition5 still constitute big challenges.
Modern alternatives for chairside CAD/CAM materials have progressed and nowadays consist of different materials such as ceramics, polymer blocks, composite resins, and acrylic resins.16 Due to their favorable mechanical and optical characteristics, as well as their lower abrasive effect on the opposing tooth, polymeric resin blocks can be considered equivalent to dental ceramics.7 Manufacturers have been creating new materials for chairside CAD/CAM that mix the advantageous features of ceramics, such as durability and color stability, with those of composite resins, such as improved flexural properties and low abrasiveness.8 A recent development in composite resin technology is the implementation of novel nanocomposites to restorative materials. CAD/CAM-processed composite resins were introduced as alternatives to ceramic blocks. Lava Ultimate is composed of resin and nanofillers (silica and zirconia nanomers), known as resin nanoceramics, and is a commercial material for CAD/CAM processing.9
In previous studies,101112 Lava Ultimate displayed a significantly higher fracture resistance than glass ceramics. Mörmann et al.13 reported that the two-body wear of Lava Ultimate did not vary significantly from that of human enamel and was identical to that of other CAD/CAM materials. Therefore, because of their mechanical features and less enamel wear, resin nanoceramics provide further benefits over glass ceramics.10111213 However, temperature differences, complex forces of mastication, and even the effects of saliva are factors that can influence the actual performance and longevity.14
In clinical practice, the fracture of CAD/CAM-produced resin nanoceramic restorations may occur during milling or wear due to trauma, parafunctional habits, or intraceramic defects of the block.15 Depending on the fracture size, intraoral adhesive repair procedures may be used. For adhesively cemented restorations in particular, the removal of the restoration may cause trauma to the pulp, tooth, and surrounding tissues. In these circumstances, repair of the restorations should be chosen over replacement because it is less costly and leads to reduced chair time for both patient and dentist.16
Repairing of a restoration surface requires both mechanical and chemical treatments. Compared to the intraoral repair of ceramic systems, the repair of resin nanoceramics does not require the use of hydrofluoric acid, which simplifies the repair procedure.17
Some authors1819 have reported the efficacy of surface treatments and aging for the repair bond strength of aged resin nanoceramic. Güngör et al.20 reported the efficacy of different surface conditionings for the bond strength of non-aged and aged resin nanoceramic. However, there is no information on the impact of both surface treatments and aging on the repair bond strength of non-aged and aged resin nanoceramic.
The goal of this research was to explore the effect of surface conditionings on the morphology and roughness of non-aged and aged resin nanoceramic, and the effect of surface conditionings and aging on the bond strengths of composites to non-aged and aged resin nanoceramics.
The research hypotheses were:
Surface conditionings affect the morphology and roughness of non-aged and aged resin nanoceramics.
Surface conditionings and aging affect the bond strengths of composites to non-aged resin nanoceramics.
Surface conditionings and aging affect the bond strengths of composite to aged resin nanoceramics.
One-hundred and twenty-six resin nanoceramic specimens (Lava Ultimate, 3M ESPE, St. Paul, MN, USA) (LU) were sliced under water cooling using a low speed slicing machine (Secotom 10, Struers A/S, Ballerup, Denmark) (15 × 14.5 × 1.5 mm). Afterwards, they were inserted into self-cure acrylic resin (Meliodent, Heraeus Kulzer GmbH, Hanau, Germany), with the upper surfaces exposed. Before the experiment, the LU samples were split into two groups [non-aged and thermally aged (5000 cycles between 5℃ and 55℃ with a transfer time of 5 s and a dwell time of 30 seconds)] (n = 63). Then, each group was split into three subgroups according to the surface treatment type (n = 21):
Untreated: Surface treatment was not performed in this group.
Air abrasion: The specimens were abraded with 50 µm Al2O3 (Korox, Bego, Bremen, Germany) particles from a distance of 10 mm at a pressure of 3 bar for 20 seconds with an intraoral air abrasion device (Microetcher IIA, Danville Materials, San Ramon, CA, USA).
Silica coating: The specimens were abraded with 30 µm SiO2 (Cojet Sand, 3M ESPE AG, Seefeld, Germany) particles from a distance of 10 mm at a pressure of 3 bar for 20 seconds with an intraoral air abrasion device (Microetcher IIA, Danville Materials, San Ramon, CA, USA).
After the surface conditionings, all samples were ultrasonically cleaned in distilled water in an ultrasonic cleaner (Sultan 600 ProSonic 600-MTH, Mexico) for 5 seconds. One specimen from each subgroup (n = 6) was examined by scanning electron microscope analysis (SEM) (LEO 440; Zeiss, Cambridge, UK) to observe the topographic effects of the surface conditionings. These specimens were only used for SEM analysis and not used in other experiments of this study. SEM images were taken at ×700 magnification.
The roughness of the specimens was measured with a profilometer (Mitutoyo Surftest SV-2100, Mitutoyo Corporation, Minatoku, Japan). Three measurements [Ra value (in µm)] were performed at the center of each specimen; the average was calculated and recorded as the roughness value.
After the roughness measurement, a repair procedure was performed. For the repair of LU material, an adhesive (Single Bond Universal Adhesive, 3M ESPE, Neuss, Germany) and nanohybrid universal composite (Filtek Z550, 3M ESPE, St. Paul, MN, USA) were used (Table 1). To limit the bonding area, an adhesive tape with a diameter of 5 mm was placed at the center of each specimen. The material's surface was brushed with adhesive, gently air dried for 5 seconds, and light polymerized for 10 seconds. Next, a separable special teflon mold (radius: 5 mm, height: 2 mm) (Fig. 1) was placed on the bonding area. Composite material was packed into the mold and polymerized for 40 seconds using a light curing unit (Elipar S10, 3M ESPE, St. Paul, MN, USA).
Then, all specimens were stored in distilled water at 37℃ for 24 hours in a dental incubator (Stardental 320S, İstanbul, Turkey) for the complete polymerization of the resin composite material. All repaired materials were then allocated into the following two groups (n = 10):
Control: In this group, repaired specimens were stored in distilled water at 37℃ for 24 hours in an incubator.
Thermal cycling: Repaired samples underwent thermal aging in a thermal cycling machine (MTE-101, Mod Dental, Ankara, Turkey) for 5000 cycles between 5℃ and 55℃ with a transfer time of 5 seconds and a dwell time of 30 seconds.
The bond strengths of the repaired resin-nanoceramic specimens were evaluated by a shear bond strength test. A universal testing machine (MIN 100, Esetron, Ankara, Turkey) was used for this process, with a crosshead speed of 1 mm/min until debonding was observed at the interface. The bond strength data were obtained in Newtons (N), then divided by the surface area (19.625 mm2) and expressed as megapascals (MPa).
After the bond strength test, the fractured samples were examined under a stereomicroscope (Olympus BX51M, Tokyo, Japan) at ×10 magnification. The fracture modes were categorized as adhesive (A) (between the resin nanoceramic and composite), cohesive (C) (within the resin nanoceramic or composite), or mixed (M) (adhesive and cohesive fractures formed at the same time). The results of the failure type analysis are given as percentage values.
The roughness and bond strength data were investigated by two-way analysis of variance (ANOVA) and three-way ANOVA, respectively. Post-hoc analysis was conducted using Tukey's honest significant difference (HSD) test. The correlation between roughness and bond strength values was analyzed by Pearson's correlation test. P < .05 was considered significant.
SEM images of the surface-treated LU specimens are given in Figure 2. The effect of surface treatment on non-aged LU was more prominent than that on aged LU. Unlike the untreated specimens (Fig. 2A, Fig. 2B), microretentive surface topography could be seen in the air-abraded and silica-treated specimens (Fig. 2C – 2F). In addition, there were more small holes on the surface of the untreated aged LU (Fig. 2B) than on the untreated non-aged LU (Fig. 2A).
According to the two-way ANOVA, only the type of surface treatment had an effect on the roughness of the LU specimens (P = .000). The material condition before repair (non-aged and aged) and the interaction between material condition before repair and surface treatment were not significant. The surface-treated specimens showed higher roughness values than the untreated group (P = .000), but there was no significant difference between air-abraded and silica-treated specimens (Table 2).
For bond strength analysis, according to three-way ANOVA, surface treatment and aging were found to be significant factors (P = .000). In addition, the interactions between surface treatment and material, and between surface treatment and aging were found to be significant (P = .001). Therefore, each factor was investigated by one-way ANOVA and Tukey's HSD test (Table 3).
For non-aged LU, surface treatment had no impact on bond strength values. In the air-abraded group of non-aged LU, bond strength was decreased after thermal cycling (P = .040), as compared to the untreated and silica-treated groups.
For aged LU, surface treatment affected the bond strength results (P < .005). In the control groups of aged LU, both the silica- and air-abraded groups presented higher bond strength than the untreated group (P ≤ .001). In the thermally cycled group of aged LU, the air-abraded group displayed higher bond strength than the untreated and silica-treated groups (P < .05). The bond strength of the silica-treated group decreased after thermal cycling (P = .002).
A significant positive correlation was also found between roughness and bond strength values (P = .003; r = 0.265), which indicated that when roughness increases, bond strength increases.
The data on failure types are presented in Table 4. In the thermally cycled groups of both non-aged and aged LU materials, mostly adhesive failures were seen. For the non-aged LU material, among the control groups and those that underwent thermal cycling, only the untreated group presented mostly mixed failure type. For the aged LU material, only the air-abraded group presented mostly mixed failure type in both the control and the thermally cycled groups.
The fracture of CAD/CAM restorations may result from intraceramic defects of the block, the milling process, trauma, clinical wear, parafunctional habits, or a combination of these factors.21 Although LU has a higher inorganic content, once cracks are formed, they propagate slowly, resulting in failure of the restoration over time.22 Small fractures or material loss of CAD/CAM restorations must be repairable to allow prolonged clinical use of the restoration.19 The effect of surface conditionings on surface changes was examined in the present study, as well as the effect of surface treatments and aging on the bond strength of composite to non-aged and aged LU because fractures can occur on new or on aged restorations in clinical practice.
The bond strengths of CAD/CAM resin nanoceramics can be increased by surface pretreatment and the use of additional adhesive.18 Özcan and Volpato23 reported that silanization significantly improved the long-term bond strength of Lava Ultimate. The Single Bond Universal Adhesive system (3M ESPE) is recommended for repairing resin nanoceramics. It contains a silane coupling agent and an MDP component that chemically primes the inorganic component of the CAD/CAM resin nanoceramic material.232425 In this study, surface treatments (air abrasion and silica coating) were used to obtain micromechanical retention, and the repair of the LU material was carried out according to the manufacturer's instructions.
The roughness analysis revealed that air abrasion and silica treatments resulted in higher roughness, compared to the untreated group (P = .000). The SEM images (Fig. 2) verified these findings. In the SEM images, the effect of surface treatment in the non-aged LU specimens (Fig. 2A, Fig. 2C, Fig. 2E) was more prominent than in the aged LU specimens (Fig. 2B, Fig. 2D, Fig. 2F). In the untreated group of aged LU material (Fig. 2B), the number of holes on the material surface was greater than that in the untreated group of non-aged LU material (Fig. 2A). This could be due to hydrolytic degradation of the LU material that occurred owing to aging before the repair procedure. Based on the roughness and SEM results, either surface treatment (air abrasion or silica) can be used for roughening non-aged and aged LU material. Therefore, the first hypothesis was accepted.
Dental restorations are subjected to intraoral thermal variations resulting from the daily routines of eating, drinking, and breathing.26 All of these factors have an effect on the performance of restorations. Restorations typically fail after being aged in a humid and thermally dynamic oral environment.27 In vitro studies2728 use thermocycling to mimic the oral environment because it applies a standardized and reproducible stress. Therefore, in this in vitro experiment, the thermal cycling method (5000 cycles) was used for aging the specimens. This cycle corresponds to a 6-month period of service.28
There are two distinct mechanisms by which thermal cycling can influence the bond strength of materials. On one hand, the mechanical stress in the interface can cause volumetric alterations, which may cause cracks and reduce bond strength.29 Alternatively, it was reported that thermocycling might raise the bond strength by enhancing the post-polymerization of the adhesive interface, such as that between CAD/CAM materials and adhesive resins.30 In the current study, for both non-aged and aged LU (except in the untreated groups), bond strength was decreased after thermal cycling. This decrease may be explained by the water sorption of the material due to thermal cycling. However, this decrease was significant only in the air-abraded nonaged LU (P = .040) and silica-treated aged LU groups (P = .002) (Table 3).
According to the bond strength analysis for non-aged LU, although the surface treatment type had no effect on the bond strength data (P > .05), aging affected the bond strength of the non-aged air-abraded LU group, which was reduced after thermal cycling (P = .040) (Table 3). Therefore, the second hypothesis was partially rejected.
For aged LU, surface treatment had a significant effect on the bond strength results (P < .005). In the control group of aged LU, the air-abraded and silica-treated groups had a higher bond strength than the untreated group (P ≤ .001); in the thermally cycled group of aged LU, the airabraded group presented a higher bond strength than the untreated group (P = .015). In addition, in the silica-treated group of aged LU, aging was found to affect the bond strength, which was found to be lower after thermal cycling (P = .002) (Table 3). Therefore, the third hypothesis was accepted.
In the literature, different studies16181920 investigated the repair bond strength of resin nanoceramic materials. Some of the studies1819 assessed the influence of surface treatments and aging on the repair bond strength of aged resin nanoceramic. Stawarczyk et al.18 reported that air-abrasion led to better bond strength results than other surface treatments and Wiegand et al.19 reported that roughened, airabraded, and silica-coated resin nanoceramics showed a higher bond strength, compared to untreated specimens. These results1819 are in accordance with our findings.
On the other hand, Duzyol et al.16 evaluated the influence of surface treatments on the repair bond strength of resin nanoceramics and Güngör et al.20 assessed the impact of surface conditioning on the bond strength of resin composites bonded to thermocycled and non-thermocycled CAD/CAM resin ceramic hybrid materials. Duzyol et al.16 reported that surface treatments reduced the bond strength of the resin nanoceramic group as compared to the control group. Güngör et al.20 pointed out that the bond strength values of untreated LU samples were significantly lower compared with those of the other surface conditioning groups. These findings1620 conflict with the results of the present study. This might be attributed to the use of different thermal cycling periods, different parameters, surface treatments, and the type of bond strength test.
Analysis of failure types can aid the interpretation of the bond strength results. In this study, the failure type findings corresponded with the bond strength values. Therefore, the findings of the SEM images, roughness, bond strength, and failure type results suggest that non-aged LU restorations do not require surface treatment before repair. However, for the repair of aged LU restorations, air abrasion should be recommended as a surface treatment method. Our findings also suggest that material condition before repair (non-aged or aged) and aging factors are more important than the selection of the surface treatment method. Therefore, clinicians should consider these factors when repairing a restoration.
The limitations of this study were the short aging period (corresponding to a 6-month service period) and method of aging (thermal cycling) used. In future studies, combined aging protocols including both thermal and loading conditions, and the long-term effect on the bond strength of resin nanoceramics should be investigated.
Air-abraded or silica-treated specimens presented rougher surfaces than untreated surfaces (P = .000). Aging affected the repair bond strength of non-aged LU; on the other side, both the surface treatment type and aging factors affected the repair bond strength of aged LU. Therefore, surface treatment is not required for the repair of non-aged LU; for aged LU restorations, air abrasion is recommended as a surface treatment method.
Figures and Tables
Fig. 2
Representative images from scanning electron microscopy of surface-treated non-aged and aged LU. (A) Non-aged untreated LU. (B) Aged untreated LU. (C) Non-aged air-abraded LU. (D) Aged air-abraded LU. (E) Non-aged silica-treated LU. (F) Aged silica-treated LU (magnification, ×700).
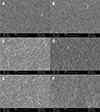
Table 1
Materials used

MDP: 10-methacryloxydecyl dihydrogen phosphate, HEMA: hydroxyethylmethacrylate, Bis-GMA: bisfenol A diglicidil ether dimethacrylate; Bis-EMA: bisfenol A polyethylene glycol dietherdimethacrylate; UDMA: urethane dimethacrylate; TEGMA: triethyleneglycoldimethacrylate; PEGDMA: polyethylene glycol dimethacrylate
Table 2
Mean roughness values (in µm) of surface treated non-aged and aged specimens
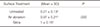
Surface Treatment | (Mean ± SD) | P |
---|---|---|
Untreated | 0.21 ± 0.13a | |
Air abrasion | 0.97 ± 0.21b | .000 |
Silica | 1 ± 0.15b |
Table 3
Statistical results of shear bond strength values (in MPa) of different groups

Table 4
Failure percentages of the groups
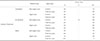
ACKNOWLEDGEMENTS
The authors thank 3M ESPE for supplying the materials (Lava Ultimate, Single Bond Universal Adhesive, Filtek Z550) of this study. Other experiments that conducted in this study were self-funded. The authors also thank Dr. İhsan Akşit (Erciyes University Technology Research and Application Center, Kayseri) for performing SEM analysis, İshak Elmas (Electric-electronic engineer, Esetron, Ankara) for using the roughness, thermal cycling and universal testing machine devices, and Erman Toktay (Statistician) for performing the statistical analysis of this study.
References
1. Giordano R. Materials for chairside CAD/CAM-produced restorations. J Am Dent Assoc. 2006; 137:14S–21S.
2. Miyazaki T, Hotta Y, Kunii J, Kuriyama S, Tamaki Y. A review of dental CAD/CAM: current status and future perspectives from 20 years of experience. Dent Mater J. 2009; 28:44–56.
3. Kois DE, Isvilanonda V, Chaiyabutr Y, Kois JC. Evaluation of fracture resistance and failure risks of posterior partial coverage restorations. J Esthet Restor Dent. 2013; 25:110–122.
4. Zahran M, El-Mowafy O, Tam L, Watson PA, Finer Y. Fracture strength and fatigue resistance of all-ceramic molar crowns manufactured with CAD/CAM technology. J Prosthodont. 2008; 17:370–377.
5. Sripetchdanond J, Leevailoj C. Wear of human enamel opposing monolithic zirconia, glass ceramic, and composite resin: an in vitro study. J Prosthet Dent. 2014; 112:1141–1150.
6. Fasbinder DJ. Materials for chairside CAD/CAM restorations. Compend Contin Educ Dent. 2010; 31:702–704. 706708–709.
7. Belli R, Geinzer E, Muschweck A, Petschelt A, Lohbauer U. Mechanical fatigue degradation of ceramics versus resin composites for dental restorations. Dent Mater. 2014; 30:424–432.
8. Coldea A, Swain MV, Thiel N. Mechanical properties of polymer-infiltrated-ceramic-network materials. Dent Mater. 2013; 29:419–426.
9. Acar O, Yilmaz B, Altintas SH, Chandrasekaran I, Johnston WM. Color stainability of CAD/CAM and nanocomposite resin materials. J Prosthet Dent. 2016; 115:71–75.
10. El-Damanhoury HM, Haj-Ali RN, Platt JA. Fracture resistance and microleakage of endocrowns utilizing three CAD-CAM blocks. Oper Dent. 2015; 40:201–210.
11. Krämer N, Kunzelmann KH, Taschner M, Mehl A, Garcia-Godoy F, Frankenberger R. Antagonist enamel wears more than ceramic inlays. J Dent Res. 2006; 85:1097–1100.
12. Magne P, Knezevic A. Simulated fatigue resistance of composite resin versus porcelain CAD/CAM overlay restorations on endodontically treated molars. Quintessence Int. 2009; 40:125–133.
13. Mörmann WH, Stawarczyk B, Ender A, Sener B, Attin T, Mehl A. Wear characteristics of current aesthetic dental restorative CAD/CAM materials: two-body wear, gloss retention, roughness and Martens hardness. J Mech Behav Biomed Mater. 2013; 20:113–125.
14. Alt V, Hannig M, Wöstmann B, Balkenhol M. Fracture strength of temporary fixed partial dentures: CAD/CAM versus directly fabricated restorations. Dent Mater. 2011; 27:339–347.
15. Ozcan M, Niedermeier W. Clinical study on the reasons for and location of failures of metal-ceramic restorations and survival of repairs. Int J Prosthodont. 2002; 15:299–302.
16. Duzyol M, Sagsoz O, Polat Sagsoz N, Akgul N, Yildiz M. The Effect of Surface Treatments on the Bond Strength Between CAD/CAM Blocks and Composite Resin. J Prosthodont. 2016; 25:466–471.
17. Hickel R, Brüshaver K, Ilie N. Repair of restorations-criteria for decision making and clinical recommendations. Dent Mater. 2013; 29:28–50.
18. Stawarczyk B, Krawczuk A, Ilie N. Tensile bond strength of resin composite repair in vitro using different surface preparation conditionings to an aged CAD/CAM resin nanoceramic. Clin Oral Investig. 2015; 19:299–308.
19. Wiegand A, Stucki L, Hoffmann R, Attin T, Stawarczyk B. Repairability of CAD/CAM high-density PMMA- and composite-based polymers. Clin Oral Investig. 2015; 19:2007–2013.
20. Güngör MB, Nemli SK, Bal BT, Ünver S, Doğan A. Effect of surface treatments on shear bond strength of resin composite bonded to CAD/CAM resin-ceramic hybrid materials. J Adv Prosthodont. 2016; 8:259–266.
21. Quinn GD, Giuseppetti AA, Hoffman KH. Chipping fracture resistance of dental CAD/CAM restorative materials: part I-procedures and results. Dent Mater. 2014; 30:e99–e111.
22. Ornaghi BP, Meier MM, Rosa V, Cesar PF, Lohbauer U, Braga RR. Subcritical crack growth and in vitro lifetime prediction of resin composites with different filler distributions. Dent Mater. 2012; 28:985–995.
23. Özcan M, Volpato CÂ. Surface Conditioning and Bonding Protocol for Nanocomposite Indirect Restorations: How and Why? J Adhes Dent. 2016; 18:82.
24. Amaral M, Belli R, Cesar PF, Valandro LF, Petschelt A, Lohbauer U. The potential of novel primers and universal adhesives to bond to zirconia. J Dent. 2014; 42:90–98.
25. Wahsh MM, Ghallab OH. Influence of different surface treatments on microshear bond strength of repair resin composite to two CAD/CAM esthetic restorative materials. Tanta Dent J. 2015; 12:178–184.
26. Palmer DS, Barco MT, Billy EJ. Temperature extremes produced orally by hot and cold liquids. J Prosthet Dent. 1992; 67:325–327.
27. Elsaka SE. Repair bond strength of resin composite to a novel CAD/CAM hybrid ceramic using different repair systems. Dent Mater J. 2015; 34:161–167.
28. Gale MS, Darvell BW. Thermal cycling procedures for laboratory testing of dental restorations. J Dent. 1999; 27:89–99.
29. Torstenson B, Brännström M. Contraction gap under composite resin restorations: effect of hygroscopic expansion and thermal stress. Oper Dent. 1988; 13:24–31.
30. Bähr N, Keul C, Edelhoff D, Eichberger M, Roos M, Gernet W, Stawarczyk B. Effect of different adhesives combined with two resin composite cements on shear bond strength to polymeric CAD/CAM materials. Dent Mater J. 2013; 32:492–501.