Abstract
PURPOSE
The purpose of this study was to evaluate the influence of acid etching treatment on surface characteristics and biological response of glass-infiltrated zirconia.
MATERIALS AND METHODS
A hundred zirconia specimens were divided into four groups depending on surface treatments: untreated zirconia (group Z); acid-etched zirconia (group ZE); glass-infiltrated zirconia (group ZG); and glass-infiltrated and acid-etched zirconia (group ZGE). Surface roughness, surface topography, surface morphology, and Vickers hardness of specimens were evaluated. For biological response test, MC3T3-E1 cell attachment and proliferation on surface of the specimens were examined. The data were statistically analyzed using one-way ANOVA and Tukey's HSD test at a significance level of 0.05.
Recently, zirconia has been widely used as dental implant substrate due to its excellent properties. Zirconia offers high flexural strength and high fracture toughness,1 optimal esthetics,2 and high biocompatibility.3
When biomaterial is introduced into human body, the interaction between the cells and the material surface contributes to osseointegration.4 A number of experiments demonstrated that rough surface zirconia (Ra > 1 µm) yielded better behavior of osteoblast cells than smooth surface.45 Therefore, several surface treatments have been put forward to improve the surface roughness, as well as other surface properties of zirconia such as topography and hardness.6
The cell response has been proved to be affected not only by the surface roughness and topography, but also by the surface chemistry.7 Bioactive glass coatings were proposed to lessen the recovery time of zirconia implant following implantation.8 Bioglass45S5 (45% SiO2, 24.5% CaO, 24.5% Na2O, and 6% P2O5 in wt%), for instance, is one of the bioactive glass which was used for zirconia coating. Its phosphorus and calcium proportion was considered equal to that of the human bone. Therefore, such glass can produce a strong chemical bond between the implant and the bone.9 Nonetheless, the coefficient of thermal expansion (CTE) of this glass does not match CTE of zirconia.10 Therefore, its use is not proper on zirconia implant.
Glass infiltration, in which glass was infiltrated into the zirconia substrate, significantly increases the strength of zirconia.11 The resultant structure involves an outer glass layer, which was followed by a graded glass-zirconia layer and a dense zirconia interior. The CTE of the glass also needs to match that value of zirconia to prevent the development of the long-range thermal stress in graded structure.12
Acid etching has been considered for a long time as an effective method to create surface roughness of titanium dental implant.13 However, zirconia is bioinert and resistant to ordinary etching techniques because it has a polycrystalline structure and lacks silica composition.1415 Meanwhile, previous studies reported that hydrofluoric acid (HF) treatment can react and eliminate the silica content of silica-based glass-ceramics, creating surface roughness.161718
This study was designed to investigate the effect of acid etching treatment on surface characteristics and biological response of glass-infiltrated zirconia.
A hundred zirconia specimens were classified into four groups depending on surface treatments (n = 25): untreated zirconia (group Z); acid-etched zirconia (group ZE); glass-infiltrated zirconia (group ZG); and glass-infiltrated and acid-etched zirconia (group ZGE). Pre-sintered zirconia (Kuwotech Co., Ltd., Gwangju, Korea) disks with 20 mm in diameter and 1.2 mm in thickness were used in this study. Untreated or as-sintered zirconia was prepared by fully sintering the pre-sintered zirconia disks at 1450℃ for 2 hours in a high temperature furnace (KaVoTherm, KaVo Dental GmbH, Biberach, Germany). Glass infiltration was conducted by dipping the pre-sintered zirconia disks in a solution of distilled water and glass powder. The specimens were then air dried and fully sintered at 1450℃ for 2 hours to make the glass infiltration and densification carried out simultaneously. The glass used in this study had the coefficient of thermal expansion close to that of zirconia and mainly consisted of SiO2, Al2O3, and Na2O (92 wt%). Acid etching treatment was carried out by immersing fully-sintered specimens in 20% HF for 1 hour at 50℃. The specimens were then neutralized by calcium oxide and ultrasonically rinsed in acetone, alcohol, and distilled water for 20 minutes each to remove any remnants.
The mean roughness (Ra, in µm) was measured at three locations on each specimen using a surface profilometer (Diavite DH-7, Asmeto AG, Basel, Switzerland). The three-dimensional surface topography of the specimens was evaluated using an atomic force microscope (AFM, Nanoscope III; Digital Instruments, Santa Barbara, CA, USA). The surface morphology of the specimens was observed using field-emission scanning electron microscopy (FE-SEM, JSM-7500F, JEOL, Tokyo, Japan).
The Vickers hardness of ten specimens per group was measured using a microhardness tester (Microwizard HM-122, Mitutoyo, Kangawa, Japan). Six Vickers indentations were made on each specimen using a load of 1 kgf for 10 seconds. Vickers hardness (VH) was calculated according to equation: where P (in kgf) is the indentation load and d (in mm) is the arithmetic mean of two diagonals. The imprint created by the indentation was observed using FE-SEM.
Mouse osteoblast-like cells MC3T3-E1 (ATCC, Manassas, VA, USA) were used in the cell experiments. The cells were grown in α-modified minimum essential medium (α-MEM, Moregate Biotech, Bulimba, QLD, Australia) containing 10% fetal bovine serum (FBS, Moregate Biotech, Bulimba, QLD, Australia) and 1% Penicilin/Streptomycin (Lonza, Walkersville, MD, USA) in a humidified atmosphere of 5% CO2 at 37℃. The cells were seeded onto the test specimens in 24-well tissue culture plates (Iwaki, Tokyo, Japan) at a density of 5 × 104 cells/well. Cell attachment was evaluated at 4 and 24 hours of incubation. At each time point, cells that were attached onto the specimens were fixed with 2.5% glutaraldehyde for 2 hours. Following dehydration in graded concentrations of ethanol, the specimens were dried in desiccator overnight and then stored at room temperature until FE-SEM evaluation was conducted. Cell proliferation at 24 and 72 hours of incubation (five specimens per group) was assessed using XTT assay (EZ-Cytox, Daeil Lab Service, Seoul, Korea). At each time point, XTT assay was added to each well and incubation was continued at 37℃ in 5% CO2 for 25 minutes. The absorbance of produced formazan was measured at a wavelength of 450 nm with the subtraction of the 630 nm background using a plate reader (ELx 800UV, BIO-TEK Instrument Inc., St. Winooski, VT, USA). Experiments were repeated three times.
IBM SPSS Statistics 20 (IBM SPSS Inc., Chicago, IL, USA) was used for statistical analysis. Thefv one-way ANOVA and Tukey's HSD test were used to make comparison among the groups. A P value < .05 was considered statistically significant.
The surface roughness values were found to arrange from the highest to the lowest value for group ZGE, ZG, ZE, and Z, respectively (Table 1). Significant differences were detected among groups (P < .05), except between group Z and group ZE. The roughness value of group ZGE, reaching 1.54 µm, was significantly higher than those of other groups (P < .05). This value was about three times greater than that of group ZG and seven times greater than that of group Z and that of group ZE.
The topography pictures of the specimens are exposed in Fig. 1. The pictures proved that the roughest surface was successfully produced on ZGE specimens.
The surface morphology images of specimens are shown in Fig. 2. The specimens of group Z had identical grain structure and closed inter-grain pores. Meanwhile, glass covered and infiltrated along the boundaries of zirconia grains in ZG specimens. For ZE and ZGE specimens, HF treatment led to the dislodgment of outer grains, uneven grain structure and extension of inter-grain spaces. Apparently, ZGE specimens had more irregular and rougher surface than ZE specimens.
The Vickers hardness values were ordered from the highest to the lowest value for group Z, ZE, ZGE, and ZG, respectively (Table 1). Statistical analysis indicated significant differences in Vickers hardness between groups (P < .05).
The imprints produced by Vickers indentation on surface of the specimens are shown in Fig. 3. The indentation patterns on Z, ZE, and ZGE surfaces were relatively alike with radial cracks emanating from the indent edges. On the other hands, no radial crack was observed on ZG surface.
The images of MC3T3-E1 cells on the specimens at 4 and 24 hours after incubation are presented in Fig. 4. Various cell-cell connections were observed on all surfaces. The number of adherent cells increased over the period of time on all the specimens and seemed to be greater on ZGE surface.
The XTT assay results of MC3T3-E1 cells at 24 and 72 hours after incubation were shown in Fig. 5. Over the observation period, the number of cells increased significantly on all surfaces. Noticeably, the cell count on ZGE surface at each time point was significantly higher than those on other groups (P < .05). The density of cells was lowest on ZG surface (P < .05).
This study evaluated the effect of acid etching treatment on glass-infiltrated zirconia's surface characteristics and biological response.
Based on the literature, the implant surfaces with Ra ≤ 1 µm are described as smooth and those with Ra > 1 µm are expressed as rough.19 In this study, group Z, ZE, and ZG showed the smooth surfaces, with the average surface roughness Ra 0.21, 0.23, and 0.50 µm, respectively. No significant difference was observed between the roughness values of groups Z and ZE. The result indicated that HF etching treatment didn't seem to produce any alteration in roughness of zirconia due to its acid inertness. This finding was in agreement with a previous study.20 On the other hand, the rough surface was only made on group ZGE (Ra = 1.54 µm), which was prepared by acid etching following glass infiltration. This roughness value of group ZGE was dramatically greater than those of the other groups. This result could be explained by the fact that HF can dissolve the silica component of glass-infiltrated zirconia and generate surface roughness.161718
Vickers hardness value of group Z was significantly higher than those of the other groups. The decrease in the hardness values of group ZE and ZGE could be explained by the relationship between surface roughness and hardness, in which the hardness was decreased as the result of the increasing roughness.2122 The unevenness of the surface morphology may be another reason for reducing hardness of such groups. Furthermore, we suggest that the greater decline in the hardness of group ZGE compared with group ZE could be assigned to the surface chemical alteration because group ZGE was introduced to the glass infiltration before acid etching process.
When the surface of a brittle material is indented with a Vickers indenter, radial cracks often generate around the indentation impression as a result of residual tensile stress that develops during the removal of the indenter.23 In the present study, FE-SEM images of the indentation impressions showed that cracks were emanated from the indentation imprints on all the surfaces, except on ZG surface. This result revealed that ZG surface seemed to be more resistant to crack initiation during the indenter removal compared with other surfaces. Actually, FE-SEM surface morphology images indicated that through glass infiltration process, glass penetrated outer zirconia grain boundaries in ZG specimens. Some previous studies reported that composite glass-zirconia structure in glass-infiltrated zirconia could reduce and transfer the residual tensile stress from the outer surface into the interior.2425 We suggested that the decrease of surface tensile stress, due to the effect of glass-zirconia structure as mentioned above, might be a possible explanation for apparently better resistance to crack generation of group ZG compared with the other groups during the removal of Vickers indenter.
The adhesion of osteoblast cells on biomaterials is strongly influenced by surface roughness.26 A few studies reported that rough surfaces favor the attachment of osteoblasts.2728 In this study, the difference in cellular attachment among the groups was examined. From the results of FE-SEM, ZGE specimens with the rough surface showed the highest cell adhesion after 4 and 24 hours of culture. We suggest that rough surface may enhance the attachment of osteoblasts compared to the smooth surface.
In the present study, we witnessed the significantly higher value of cell proliferation on ZGE surface than on the other surfaces. This finding was in accordance with the previous studies, which reported the higher proliferation rate of osteoblasts on rough zirconia surface than on smooth one.45 We suggest that the surface roughness is able to influence the proliferation of cell on zirconia in the way that rough surface may improve the proliferation of osteoblasts.
Rough surface, which was created on group ZGE, takes advantages of the osteoblastic response. However, the greater surface roughness may also lead to the higher rate of the attachment of microorganisms around the implant.29 Therefore, more experiments will be conducted in the near future to evaluate the influence of such surface treatment on the behavior of bacteria on zirconia implants.
Surface chemical modification through glass infiltration process may also affect the behavior of osteoblasts. Cell experiments showed that osteoblast proliferation was significantly lower in group ZG compared with the other groups. Actually, bioactive glass, based on silicate content, attached to the bone through the formation of a silica-rich layer on which hydroxyapatite was formed.30 High content of Al2O3 may diminish the bioactivity of the glass due to the formation of Si-O-Al linkages, which reduce the release of Si and reduce the formation of hydroxyapatite layer on material surface.31 In fact, Al2O3 is one of the main compositions of the glass used in this study. This could explain the worst cell behavior of group ZG. The glass used in this study, therefore, may need to be further modified to improve the bioactivity.
A limitation of the present study is that study examined the attachment and proliferation of cell in a short period of time. More comprehensive outcomes would be achieved with long-term evaluation. Further studies are needed to perform the osteoblastic response in vivo. Likewise, glass infiltration and acid etching can be a promising solution for zirconia surface treatments and requires more inclusive studies.
References
1. Kelly JR, Denry I. Stabilized zirconia as a structural ceramic: an overview. Dent Mater. 2008; 24:289–298. PMID: 17624420.


2. Zarone F, Russo S, Sorrentino R. From porcelain-fused-to metal to zirconia: clinical and experimental considerations. Dent Mater. 2011; 27:83–96. PMID: 21094996.
3. Uo M, Sjogren G, Sundh A, Watari F, Bergman M, Lerner U. Cytotoxicity and bonding property of dental ceramics. Dent Mater. 2003; 19:487–492. PMID: 12837396.


4. Yamashita D, Machigashira M, Miyamoto M, Takeuchi H, Noguchi K, Izumi Y, Ban S. Effect of surface roughness on initial responses of osteoblast-like cells on two types of zirconia. Dent Mater J. 2009; 28:461–470. PMID: 19721284.


5. Bächle M, Butz F, Hübner U, Bakalinis E, Kohal RJ. Behavior of CAL72 osteoblast-like cells cultured on zirconia ceramics with different surface topographies. Clin Oral Implants Res. 2007; 18:53–59. PMID: 17224024.


6. Ewais OH, Al AF, Ghoneim MM, Aboushelib MN. Novel zirconia surface treatments for enhanced osseointegration: laboratory characterization. Int J Dent. 2014; 2014:203940. PMID: 25349610.


7. Schwartz Z, Boyan BD. Underlying mechanisms at the bone-biomaterial interface. J Cell Biochem. 1994; 56:340–347. PMID: 7876327.


8. Krajewski A, Malavolti R, Piancastelli A. Albumin adhesion on some biological and non-biological glasses and connection with their Z-potentials. Biomaterials. 1996; 17:53–60. PMID: 8962948.


10. Kristen A, Hausmann A, Weber M, Fisher J, Fisher H. Bioactive and thermally compatible glass coating on zirconia dental implants. J Dent Res. 2015; 94:297–303. PMID: 25421839.


11. Srikanth R, Kosmac T, Bona AD, Yin L, Zhang Y. Effects of cementation surface modifications on fracture resistance of zirconia. Dent Mater. 2015; 31:435–442. PMID: 25687628.


12. Suresh S. Graded materials for resistance to contact deformation and damage. Science. 2001; 292:2447–2451. PMID: 11431558.


13. Iwaya Y, Machigashira M, Kanbara K, Miyamoto M, Noguchi K, Izumi Y, Ban S. Surface properties and biocompatibility of acid-etched titanium. Dent Mater J. 2008; 27:415–421. PMID: 18717170.


14. Thompson JY, Stoner BR, Piascik JR, Smith R. Adhesion/cementation to zirconia and other non-silicate ceramics: where are we now? Dent Mater. 2011; 27:71–82. PMID: 21094526.


15. Blatz MB, Sadan A, Kern M. Resin-ceramic bonding: a review of the literature. J Prosthet Dent. 2003; 89:268–274. PMID: 12644802.


16. Canay S, Hersek N, Ertan A. Effect of different acid treatments on a porcelain surface. J Oral Rehabil. 2001; 28:95–101. PMID: 11298915.
17. Borges GA, Sophr AM, de Goes MF, Sobrinho LC, Chan DC. Effect of etching and airborne particle abrasion on the microstructure of different dental ceramics. J Prosthet Dent. 2003; 89:479–488. PMID: 12806326.


18. Pisani-Proenca J, Erhardt MC, Valandro LF, Gutierrez-Aceves G, Bolanos-Carmona MV, Del Castillo-Salmeron R, Bottino MA. Influence of ceramic surface conditioning and resin cements on microtensile bond strength to a glass ceramic. J Prosthet Dent. 2006; 96:412–417. PMID: 17174658.


19. Sykaras N, Iacopino AM, Marker VA, Triplett RG, Woody RD. Implant materials, designs, and surface topographies: their effect on osseointegration. A literature review. Int J Oral Maxillofac Implants. 2000; 15:675–690. PMID: 11055135.
20. Bona A, Donassollo TA, Demarco FF, Barrett AA, Mecholsky JJ Jr. Characterization and surface treatment effects on topography of a glass-infiltrated alumina/zirconia-reinforced ceramic. Dent Mater. 2007; 23:769–775. PMID: 17112579.
21. Flury S, Peuzfeldt A, Lussi A. Influence of surface roughness on mechanical properties of two computer-aided design/computer-aided manufacturing (CAD/CAM) ceramic materials. Oper Dent. 2012; 37:617–624. PMID: 22616923.


22. Keshavarz M, Idris MH, Ahmad N. Mechanical properties of stabilized zirconia nanocrystalline EB-PVD coating evaluated by micro and nanoindentation. J Adv Ceram. 2013; 2:333–340.
23. Jamie JK, Robert OR. Determining the toughness of ceramics from Vickers indentation using the crack-opening displacements: an experimental study. J Am Ceram Soc. 2003; 86:1433–1436.
24. Zhang Y, Ma L. Optimizaion of ceramic strength using elastic gradients. Acta Mater. 2009; 57:2721–2729. PMID: 20161019.
25. Zhang Y, Sun MJ, Zhang DZ. Designing functionally graded materials with superior load bearing properties. Acta Biomater. 2012; 8:1101–1108. PMID: 22178651.
27. Carlsson L, Rostlund T, Albrektsson B, Albrektsson T, Branemark PI. Osseointegration of titanium implants. Acta Orthop Scand. 1986; 57:285–289. PMID: 3788488.


28. Bowers KT, Keller JC, Randolph BA, Wick DG, Michaels CM. Optimization of surface micromorphology for enhanced osteoblast responses in vitro. Int J Oral Maxillofac Implants. 1992; 7:302–310. PMID: 1289255.
29. Teughels W, Van AN. Effect of material characteristics and/surface topography on biofilm development. Clin Oral Implants Res. 2006; 17:68–81. PMID: 16968383.
30. Abbasi Z, Bahrololoom ME, Shariat MH, Bagheri R. Bioactive glasses in dentistry: a review. J Dent Biomater. 2015; 2:1–9.
Fig. 3
FE-SEM images of Vickers indentation impression in the outer surface of specimens. (A) Z, (B) ZE, (C) ZG, (D) ZGE. The arrows indicate radial cracks emanating from the indent edges.
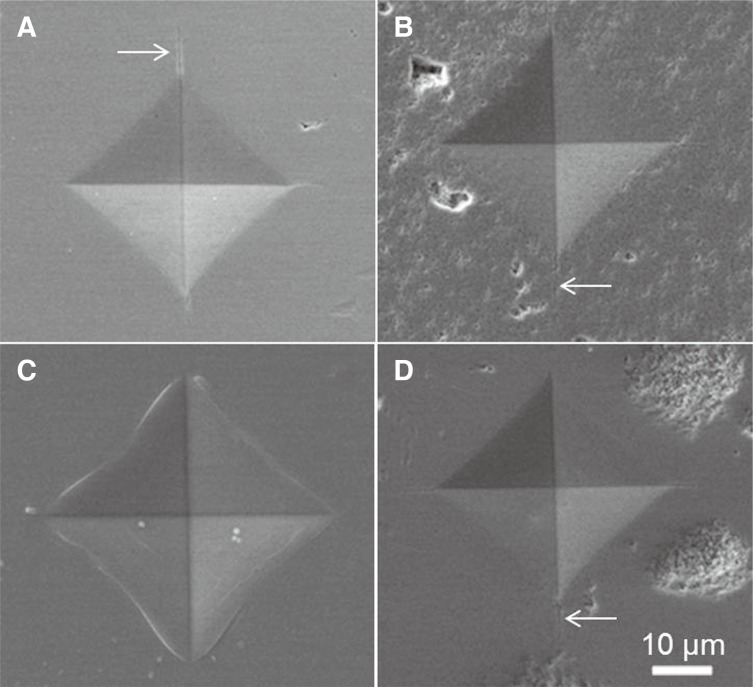
Fig. 4
FE-SEM images (×300 magnification) of MC3T3-E1 on specimens at 4 hours (A) and 24 hours (B) after incubation.
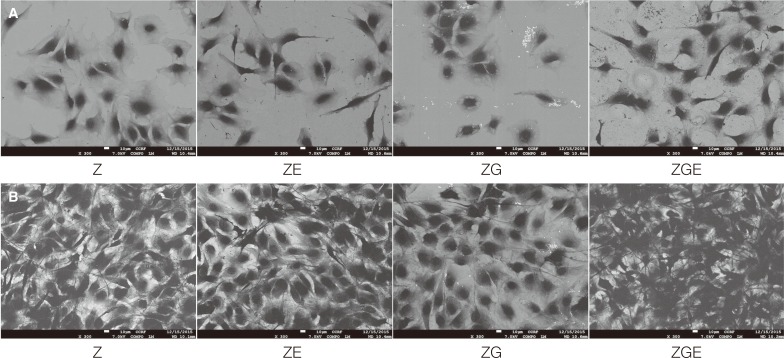
Fig. 5
Evaluation of MC3T3-E1 cell proliferation by XTT assay. Different letters mean significant difference (P < .05).
