Abstract
PURPOSE
Polyamide polymers do not provide sufficient bond strength to auto-polymerized resins for repairing fractured denture or replacing dislodged denture teeth. Limited treatment methods have been developed to improve the bond strength between auto-polymerized reline resins and polyamide denture base materials. The objective of the present study was to evaluate the effect of surface modification by acetic acid on surface characteristics and bond strength of reline resin to polyamide denture base.
MATERIALS AND METHODS
84 polyamide specimens were divided into three surface treatment groups (n=28): control (N), silica-coated (S), and acid-treated (A). Two different auto-polymerized reline resins GC and Triplex resins were bonded to the samples (subgroups T and G, respectively, n=14). The specimens were subjected to shear bond strength test after they were stored in distilled water for 1 week and thermo-cycled for 5000 cycles. Data were analyzed with independent t-test, two-way analysis of variance (ANOVA), and Tukey's post hoc multiple comparison test (α=.05).
RESULTS
The bond strength values of A and S were significantly higher than those of N (P<.001 for both). However, statistically significant difference was not observed between group A and group S. According to the independent Student's t-test, the shear bond strength values of AT were significantly higher than those of AG (P<.001).
In recent years, various thermoplastic materials have been developed for the fabrication of no-clasp removable prostheses and complete dentures. The flexural and impact strength of polyamide polymers are higher than those of heat-cured denture base materials.12345 Nevertheless, the repair strength of auto-polymerized resins to polyamide polymers is too weak, and the repair mostly requires laboratory procedures.6789
Denture base repair or relining is mandatory for the prolonged service life of the prosthesis. Auto-polymerized reline resins are based on poly (methyl methacrylate) (PMMA) or poly (ethyl methacrylate) (PEMA) copolymer, which can effectively bond to heat-cured denture base materials due to the relatively similar chemical compositions.10111213 Polyamide is a thermoplastic material produced by a condensation reaction between a diamine and a dibasic acid.714 The chemical resistance of polyamide polymers is due to their high degree of crystallinity; the penetration of monomer molecules and other resin primers into the polymers is difficult.3815 Therefore, nylon polymers do not provide sufficient bond strength to auto-polymerized resins for repairing fractured denture or replacing dislodged denture teeth.89
Despite the different existing surface treatment methods used to improve the bond strength of auto-polymerized reline materials to common heat-cured denture base resins, researches dealing with the creation of new polyamide polymers with an improved adhesion properties continue to be active.11011121316 Previous studies89 indicated that silica coating followed by silanization increased the bond strength between auto-polymerized resin and polyamide polymers. In air blast, the collision of the accelerated silica-coated alumina particles with the substrate results in the kinetic energy transfer and microscopic melting of the substrate, leading to particle penetration and retention by adhesive force.17 Although, the initial bond is reported to exhibit satisfying strength, it isn't reliable since the strength is decreased after aging procedures.918 Therefore, it is essential to find a broadly applicable and cost-effective approach to overcome such problems and to promote bonding.
The solvent-assisted bonding is another method for bonding two substrates that has been widely studied and utilized for repairing thermosetting acrylic resins.11016 Although polyamide is a particularly chemical-resistant material, the presence of amide groups (-NHCO-) makes it prone to absorb water or other solvents and form hydrogen bonds.1920 It is well recognized that the plasticization of polyamide matrix occurs in the presence of small amounts of polar molecules capable of hydrogen bonding.2122 The softening enhances the chain mobility and disrupts the hydrogen bond network of polyamides.151923 Although acetic acids, propionic acids, and butyric acids with low dissociation constant are non-solvents, they promote the reaction through hydrogen bonding. In addition, they cause hydrolysis that breaks the cross-links and provides swelling generally similar to those of solvents.24
The hydrolytic degradation of polyamide in different environments has been extensively studied.20222526 Acidic conditions have been observed to facilitate amide hydrolysis. The chemical effects that acid has on the hydrolysis of polyamide are amine scavenging and acid catalysis. According to previous studies,2027 polyamide hydrolysis reaches an equilibrium molecular status in the absence of oxygen. Serpe et al.22 and Chaupart et al.28 showed that significant degradation was achieved in strong acids, but the presence of oxygen was uncontrollable. Moreover, no equilibrium was observed in the hydrolysis of polyamide chain leading to less swelling. The reports by Hocker et al.24 showed that the hydrolysis of polyamide caused by small organic acids was accelerated significantly. Therefore, hydrolysis was possible without severe damage to the structure.
The objective of the present study was to evaluate the effect of surface treatment with acetic acid on the bond strength of auto-polymerized resin to polyamides. The null hypothesis was that the application of acetic acid would not affect the bond strength of auto-polymerized resin to polyamide denture base material.
Table 1 summarizes the materials used in this study. A thermoplastic denture base material, a hard chairside reline resin, and an auto-polymerized acrylic resin were selected.
84 polyamide cylinders with the dimensions of 15 mm diameter and 5 mm height were processed, following the manufacturer's instructions, using an injection method at 254℃. The surface of the samples was finished successively using 600, 800, 1000, and 1200 grit silicon carbide papers (Shanghai Hangli Co., Shanghai, China), ultrasonically cleaned, and stored in distilled water at 37℃ for a week. The samples were randomly divided into three groups (n = 28) according to the type of surface treatment:
Tribochemical silica coating was performed using Rocatec Plus (110-µm grain size) under a pneumatic pressure of 0.28 MPa at a distance of 10 mm for 10 seconds. The silica-coated specimens were further treated with a silane coupling agent for 60 seconds. Chemical modification was performed with acetic acid. The samples were treated with a solution of 5% acetic acid in aqueous ethanol (30/70, v/v). After 10 minutes, the samples were prepared for bonding to auto-polymerized resin.
The surface roughness and topographical characterization of the samples were evaluated by atomic force microscopy (Nanosurf Mobile S; Liestal, Switzerland) operated in non-contact mode. Data were acquired on a 100 µm linear scanner using high resonance frequency (F0 = 260 kHz) cantilever with a silicon probe having 47 N/m force constant.
ATR-FTIR was performed to detect the functional groups formed on the surface of the acid treated polyamide. The infrared spectra of the treated and untreated polyamide samples were recorded in the region 400-4000 cm-1 on a Shimadzu FTIR-8400S spectrophotometer (Shimadzu FTIR-8400S; Shimadzu, Japan) at a spectral resolution of 4 cm-1.
After preparing the surface, the polyamide samples were embedded in tubes filled with auto-polymerized resin (Elite SC Tray; Zhermack, Badia Polesine, Italy). According to the type of autopolymerizing resin materials, each group (N, S, and A) was further divided into two subgroups (G for GC reline resin, T for Triplex reline resin; n = 14). Then, the samples were prepared for bonding the auto-polymerized resins to the polyamide samples. A layer of GC resin primer was applied on the polyamide surfaces in NG, SG, and AG groups. However, the samples in NT, ST, and AT groups were prepared for bonding without further modification. A piece of double sided adhesive tape with a central hole of 3 mm diameter was utilized to mark the bonding area. A stainless steel cylinder with an inner diameter of 3 mm was placed on the demarcated bonding area and the auto-polymerized resin was built up within the cylinder by using paint-on-brush technique. The samples were stored in distilled water at 37℃ for one week and then subjected to 5000 thermal cycles at 5 - 55℃ with a 15-seconds dwell time to simulate thermal fluctuations in the oral environment.
The samples were mounted on a universal testing machine (Hounsfield Test Equipment; Model H5-KS, Surrey, UK), and then shear force was applied at a crosshead speed of 0.5 mm/min until failure (Fig. 1). The maximum failure loads were recorded in newtons (N) and converted into megapascals (MPa) by dividing the values (in N) by the bonding areas (in mm2).
Statistical analysis was performed using SPSS statistical software (version 21). The Kolmogorov-Smirnov test was used for the normality of the bond strength values. The means and standard deviations (SD) of shear bond strength were analyzed with a two-way analysis of variance (ANOVA) followed by Tukey's multiple comparison test. The significance level was set at 5%.
The bond strength values of surface treatment samples were compared with those of untreated groups. According to the two-way ANOVA, surface treatments, auto-polymerized resin types, and their interactions were statistically significant (P < .001) (Table 2). The mean shear bond strength values, standard deviations, and statistical differences between surface treatment and resin groups are presented in Table 3. The bond strength values of A and S were significantly higher than those of N (P < .001 for both). Although the bond strength values of group A was higher than those of group S, the difference was not statistically significant (P = .234).
AT yielded the highest bond strength value (33.20 ± 7.11 MPa), which was significantly higher than that observed in subgroup AG (P < .001). Comparison of the results using independent Student's t-test revealed no significant difference between ST and SG groups (P = .119) and between NT and NG (P = .502).
Surface morphology and root mean square roughness (RMS) of the treated specimens were assessed by atomic force microscopy (AFM). AFM topographic images of the surfaces of the specimens are shown in Fig. 2. The colored intensities represent the vertical profile of the surface. The darker contrasts correspond to the lower areas of the surface and the lighter contrasts correspond to the higher parts. It is reasonable that a rough surface indicates a large effective surface area. AFM revealed that surface roughness was increased after the treatment of polyamide. The RMS roughness of group N was 51.354 nm, which was increased to 92.637 nm and 259.96 nm after acid acetic treatment and blasting, respectively.
Fig. 3 represents the IR spectra of the original and acid treated polyamide. The broad peak around 3000 - 3500 cm-1 indicates the existence of hydroxyl stretching vibration (-OH), which confirms the hydrolysis and the abundance of carboxylic groups. The peak at ~1650 cm-1 is attributed to the C=O stretching vibration of carboxylic and amide group. The peaks at ~1100 and 1400 cm-1 represents the C-O and C-N stretching vibrations, respectively.
The present study evaluated the bond strength of auto-polymerized resin to thermoplastic denture base materials with surface modification by acetic acid. The application of acid treatment prior to bonding increased the shear bond strength between auto-polymerized resins and thermoplastic denture base materials; thus, the null hypothesis was rejected. Various laboratory test methods have been used to evaluate the bond strength between reline resins and denture base materials, and bond strength characteristics differ in accordance with the applied methods.29 However, the shear test is a widely adopted test to assess the bond interface and simulates the clinical environments better than tensile force.1112
In consistence with previous studies,89 silica coating and subsequent application of silane coupling agent increased the bond strength between thermoplastic and auto-polymerized resins. The polyamide polymers are easily affected by heat due to their low thermal characteristics.2314 The high collision energy of accelerated silica-coated alumina particles softens the surface of thermoplastic polymer and causes the silica particles to be embedded into the surface of polymer.17 Therefore, silica coating provides not only micromechanical retention but also sites for chemical reaction, enhancing the bond strength of the relining material to the silanized polyamide substrate.1530 However, it is noteworthy to mention that nylon polymer is more flexible and softer than metal or ceramics. Thus, blasting the small surface area leads to substantial substance loss.18 Moreover, covering the large and complex surface areas tribochemically is not practical in most cases and results in the formation of a non-continuous silica layer on the surface.1831 Consequently, the distribution of siloxane (Si-O-Si) bonds created by silane application is heterogeneous and concentrated in local regions.31
The results of the present study revealed that surface modification by acetic acid increased the bond strength of both of the auto-polymerized resins compared with the control group. It has been reported that the bond strength between a reline resin and denture base material is influenced by the nature of the two resins. The auto-polymerized reline resin easily bonds to the thermosetting denture base materials due to an almost similar chemical composition.101112 The resin monomers are bound to the remaining functional groups, and the covalent bond forms between the freshly added reline resin and the existing heat-cure denture base material. Previous studies1101216 have provided evidence that chemical surface treatment of heat polymerized resin materials with conditioning liquids disintegrated the bonding surface, improved monomer diffusion, and finally increased the bond strength of repaired resin materials. However, thermoplastic polymer belongs to the class of polyamides with a semi-crystal structure in which the amide groups are intercalated along linear alkane chains.22432 The polymer has significant chain-chain interaction arising from hydrogen bonds between the adjacent amide groups.23 It is generally accepted that the polyamides are strongly resistant to chemical agents due to high degree of crystallinity.222528 Thus, they are hard to react with monomers and resin primers of relining materials. This is proved with the observation of adhesive failures between auto-polymerized resins and polyamide.8
The ability of a solvent or a mixture of solvents in dissolving polyamide is strongly affected by the presence of polar amide groups (-CONH-).19 Molecular dissolution of polyamide requires a strong interaction between a solvent and the amide groups, which would lead to the disruption of the inter-amide hydrogen bonding. Concurrently, the methylene sequences loosen as a consequence of polymer swelling.192123 The hydrolytic breakdown of amide bonds in strong acids is problematic since it would lead to relative decrease in molecular weight of the polymer, severe destruction, and loss of surface integrity.2228 Moreover, the rate constant of acid hydrolysis depends on acid concentration; in the presence of concentrated acids, the reaction rate is decreased due to reduced water activity.202225 Hocker et al.24 showed that the rate of polyamide hydrolysis in solutions containing small weak organic acid was twice that of a water hydrochloric acid solution at the same pH. It was observed that the rates of the hydrolysis were accelerated as the acid strength decreased. Measuring the concentration of acid in polyamide revealed that polyamide has preferential tendency towards small organic acids.202224 Therefore, in the present study, dilute acetic acid solution was applied to induce polyamide hydrolysis.
The acidic hydrolysis of polyamide is a bimolecular reaction of oxygen protonized amide with water and the formation of the tetrahedral intermediate.2022242526 Mechanical degradation leads to the formation of the radicals affecting the structure of the amide groups and accelerating hydrolysis.26 Random chain scissions of amide bonds that are accordingly positioned between adjacent chains enable higher chain mobility.222325 The dissociation of polyamide involves cleavage of the C-N bond between amide (-NH) and carbonyl (=C=O) groups.24 As the reaction rate is considerably low at room temperature, it was expected that short-term exposure to acid would not give rise to detectable IR changes; but the ATR-FTIR spectroscopy revealed an increased intensity of amide and carbonyl bands. The increase in the N-H deformation and C=O stretching bands at ~1540 cm-1 and ~1650 cm-1, respectively, confirmed the amide bond dissociation. Additionally, the emergence of a broad band between 3000 and 3500 cm-1 corresponded to hydroxyl absorption.
Acid dissociates in the matrix of water-swollen polyamide and moves around under the influence of chemical potential and the electric field of ionic movement.192228 Reduction of the pH in the polymer inhibits re-polymerization of cleaved amide bond and results in softening of the polymer. It seems that the plasticization of the polymer is accompanied by phase inflation, which facilitates the diffusion of monomer into denture base material, cross-linking with auto-polymerized resin, chain entanglements, and the formation of interpenetrating polymer network (IPN).1920212225262833 IPN refers to a specific class of polymer mixtures consisting of two or more polymers in a network form when one of the polymers is synthesized or crosslinked in the presence of the others.34 IPNs are prepared by different methods. The semi-IPN is an intermediate system in which a thermosetting polymer network is formed and crosslinked within a thermoplastic matrix.3435 Although none of these definitions are exactly consistent with the condition of the present study, entanglements of polymer chains serve as a type of crosslink. Thus, the functional polymer is physically crosslinked with the thermoplastic polymer.
The swelling and plasticization of polymer increase the structural mobility of polymer chains, and therefore a larger number of hydrophilic functional groups are exposed.36 In this regard, the diffusion theory of adhesion may be extended to the interface bonding of polymers. Based on the theory, adhesion is achieved by mutual penetration of both the substrate and the adhesive.15 The mobile polymer chains diffuse across the solvated layer of polyamide, leading to extensive intertwining of chains between the surfaces and resulting in strong bonds.32333536 In the present study, the acid-swollen polymer is believed to be responsible for the enhanced diffusion of monomer molecules in polymers with inter diffusion of polymer chains between the surfaces.
The present study showed a significant increase in bond strength of auto-polymerized reline resin for group AT compared with that for AG, while the results were not significantly different between SG and ST. The auto-polymerized reline resins are categorized into two groups: monomer-containing methyl methacrylate and high-molecular-weight methyl methacrylate.6 The bonding of reline resins to denture base materials is achieved by penetration and diffusion of monomers into denture base resin and depends on various variables such as temperature, concentration, morphology, molecular weight, and degree of crystallinity of the polymer.1101236 Higher diffusion rate of resin monomers in a swollen polymer may cause higher bond strength between the reline and denture base resins.36 The diffusion of high-molecular-weight methyl methacrylate molecules can, however, be impeded to a greater degree than that of small MMA monomer molecules, leading to an apparent lower infusion of monomer into acid swollen polymer matrix.
The AFM analysis represented an increased surface roughness of grit blasted specimens compared with that of acid treated specimens. Therefore, nanoscale roughness cannot solely explain the improved bond strength of auto-polymerized resin to polyamide. The increased bond strength appears to be related mostly to chemical reactions occurring at the interface rather than the surface morphology. However, more detailed investigations should be conducted to obtain more accurate information regarding the precise effects of acid treatment and its durations on various thermoplastic polymers.
Generally, it is concluded that treatment of polyamide surface by acetic acid evidently enhances the bond between reline resins and polyamide polymer, which is comparable to those obtained with tribochemical silica coating. Therefore, procedures for denture base repairing or relining can be performed at the same appointment without any need of sophisticated equipment or sending the prosthesis to the responsible laboratory.
Within the limitations of this study, it was concluded that both of the surface treatment methods influenced the shear bond strength (P < .001). Although the bond strength of auto-polymerized resin to acid treated polyamide polymer was higher than that to polymer with tribochemical silica coating and further application of silane, the difference was not statistically significant (P = .234). Also, it appeared that acetic acid surface treatment had the greatest effect on the bond strength of MMA based reline resin.
Figures and Tables
Fig. 2
AFM images of polyamide after treatment with acetic acid (A), silica coating (B), and without any treatment (C).
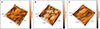
Fig. 3
ATR-FTIR spectra of the polyamide after treatment with acetic acid (a) and without any treatment (b).
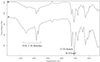
Table 1
Materials used in this study
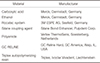
Table 2
Results of two-way ANOVA test

Table 3
Mean and standard deviation of shear bond strength values and differences between treatment groups (MPa)

Group | Mean (SD) | Difference | |
---|---|---|---|
G | T | ||
A | 18.02b | 33.20a | A |
A | 20.92a | 25.26a | S |
B | 5.99a | 5.07a | N |
A: Surface treatment using acetic acid, S: Silica coating followed by silane application, N: No treatment, T: Triplex reline resin, G: GC reline resin.
Values with distinct upper case subscript letters (A-B) are significantly different (P < .05).
Values with the same lower case subscript letters (a-b) are not statistically different (P > .05).
References
1. Meng GK, Chung KH, Fletcher-Stark ML, Zhang H. Effect of surface treatments and cyclic loading on the bond strength of acrylic resin denture teeth with autopolymerized repair acrylic resin. J Prosthet Dent. 2010; 103:245–252.
2. Ucar Y, Akova T, Aysan I. Mechanical properties of polyamide versus different PMMA denture base materials. J Prosthodont. 2012; 21:173–176.
3. Fueki K, Ohkubo C, Yatabe M, Arakawa I, Arita M, Ino S, Kanamori T, Kawai Y, Kawara M, Komiyama O, Suzuki T, Nagata K, Hosoki M, Masumi S, Yamauchi M, Aita H, Ono T, Kondo H, Tamaki K, Matsuka Y, Tsukasaki H, Fujisawa M, Baba K, Koyano K, Yatani H. Clinical application of removable partial dentures using thermoplastic resin-part I: definition and indication of non-metal clasp dentures. J Prosthodont Res. 2014; 58:3–10.
4. Kohli S, Bhatia S. Flexural properties of polyamide versus injection-molded polymethylmethacrylate denture base materials. Eur J Prosthodont. 2013; 1:63–67.
5. Soygun K, Bolayir G, Boztug A. Mechanical and thermal properties of polyamide versus reinforced PMMA denture base materials. J Adv Prosthodont. 2013; 5:153–160.
6. Alkurt M, Yeşil Duymuş Z, Gundogdu M. Effect of repair resin type and surface treatment on the repair strength of heat-polymerized denture base resin. J Prosthet Dent. 2014; 111:71–78.
7. Fueki K, Ohkubo C, Yatabe M, Arakawa I, Arita M, Ino S, Kanamori T, Kawai Y, Kawara M, Komiyama O, Suzuki T, Nagata K, Hosoki M, Masumi S, Yamauchi M, Aita H, Ono T, Kondo H, Tamaki K, Matsuka Y, Tsukasaki H, Fujisawa M, Baba K, Koyano K, Yatani H. Clinical application of removable partial dentures using thermoplastic resin. Part II: Material properties and clinical features of non-metal clasp dentures. J Prosthodont Res. 2014; 58:71–84.
8. Kim JH, Choe HC, Son MK. Evaluation of adhesion of reline resins to the thermoplastic denture base resin for non-metal clasp denture. Dent Mater J. 2014; 33:32–38.
9. Katsumata Y, Hojo S, Hamano N, Watanabe T, Yamaguchi H, Okada S, Teranaka T, Ino S. Bonding strength of autopolymerizing resin to nylon denture base polymer. Dent Mater J. 2009; 28:409–418.
10. Sarac YS, Sarac D, Kulunk T, Kulunk S. The effect of chemical surface treatments of different denture base resins on the shear bond strength of denture repair. J Prosthet Dent. 2005; 94:259–266.
11. Takahashi Y, Chai J. Assessment of shear bond strength between three denture reline materials and a denture base acrylic resin. Int J Prosthodont. 2001; 14:531–535.
12. Mutluay MM, Ruyter IE. Evaluation of adhesion of chairside hard relining materials to denture base polymers. J Prosthet Dent. 2005; 94:445–452.
13. Azevedo A, Machado AL, Giampaolo ET, Pavarina AC, Vergani CE. The effect of water immersion on the shear bond strength between chairside reline and denture base acrylic resins. J Prosthodont. 2007; 16:255–262.
14. Takabayashi Y. Characteristics of denture thermoplastic resins for non-metal clasp dentures. Dent Mater J. 2010; 29:353–361.
15. Brown HR. Adhesion of polymers. MRS Bull. 1996; 21:24–27.
16. Meloto CB, Silva-Concílio LR, Rodrigues-Garciai RC, Canales GT, Rizzatti-Barbosa CM. Effect of surface treatments on the bond strength of different resin teeth to complete denture base material. Acta Odontol Latinoam. 2013; 26:37–42.
17. Heikkinen TT, Lassila LV, Matinlinna JP, Vallittu PK. Effect of operating air pressure on tribochemical silica-coating. Acta Odontol Scand. 2007; 65:241–248.
18. Kern M, Thompson VP. Sandblasting and silica coating of a glass-infiltrated alumina ceramic: volume loss, morphology, and changes in the surface composition. J Prosthet Dent. 1994; 71:453–461.
19. Nirmala R, Panth HR, Yi C, Nam KT, Park SJ, Kim HY, Navamathavan R. Effect of solvents on high aspect ratio polyamide-6 nanofibers via electrospinning. Macromol Res. 2010; 18:759–765.
20. Jacques B, Werth M, Merdas I, Thominette F, Verdu J. Hydrolytic ageing of polyamide 11. 1. Hydrolysis kinetics in water. Polymer. 2002; 43:6439–6447.
21. Richaud E, Derue I, Gilormini P, Verdu J, Vaulot C, Coquillat M, Desgardin N, Vandenbrouke A. Plasticizer effect on network structure and hydrolytic degradation. Eur Polym J. 2015; 69:232–246.
22. Serpe G, Chaupart N, Verdu J. Ageing of polyamide 11 in acid solutions. Polymer. 1997; 38:1911–1917.
23. Murthy NS. Hydrogen bonding, mobility, and structural transitions in aliphatic polyamides. J Polym Sci Part B: Polym Phys. 2006; 44:1763–1782.
24. Hocker S, Rhudy AK, Ginsburg G, Kranbuehl DE. Polyamide hydrolysis accelerated by small weak organic acids. Polym. 2014; 55:5057–5064.
25. El-Mazry C, Correc O, Colin X. A new kinetic model for predicting polyamide 6-6 hydrolysis and its mechanical embrittlement. Polym Degrad Stab. 2012; 97:1049–1059.
26. Bernstein R, Derzon DK, Gillen KT. Nylon 6.6 accelerated aging studies: thermal-oxidative degradation and its interaction with hydrolysis. Polym Degrad Stab. 2005; 88:480–488.
27. Meyer A, Jones N, Lin Y, Kranbuehl D. Characterizing and modeling the hydrolysis of polyamide-11 in a pH 7 water environment. Macromolecules. 2002; 35:2784–2798.
28. Chaupart N, Serpe G, Verdu J. Molecular weight distribution and mass changes during polyamide hydrolysis. Polymer. 1998; 39:1375–1380.
29. Sirisha K, Rambabu T, Ravishankar Y, Ravikumar P. Validity of bond strength tests: A critical review-Part II. J Conserv Dent. 2014; 17:420–426.
30. Lung CY, Matinlinna JP. Aspects of silane coupling agents and surface conditioning in dentistry: an overview. Dent Mater. 2012; 28:467–477.
31. Sarmento HR, Campos F, Sousa RS, Machado JP, Souza RO, Bottino MA, Ozcan M. Influence of air-particle deposition protocols on the surface topography and adhesion of resin cement to zirconia. Acta Odontol Scand. 2014; 72:346–353.
32. Sakai T, Sembokuya H, Kubouchi M, Tsuda K. The reciprocal influence between ion transport and degradation of PA66 in acid solution. Polym Degrad Stab. 2006; 91:2595–2604.
33. Ward JH, Furman K, Peppas NA. Effect of monomer type and dangling end size on polymer network synthesis. J Appl Polym Sci. 2003; 89:3506–3519.
34. Vallittu PK. Interpenetrating polymer networks (IPNs) in dental polymers and composites. J Adhes Sci Technol. 2009; 23:961–972.
35. Liu S, Zhao N, Rudenja S. Surface interpenetrating networks of poly(ethylene terephthalate) and polyamides for effective biocidal properties. Macromol Chem Phys. 2010; 211:286–296.
36. Wolff D, Geiger S, Ding P, Staehle HJ, Frese C. Analysis of the interdiffusion of resin monomers into pre-polymerized fiber-reinforced composites. Dent Mater. 2012; 28:541–547.