Abstract
PURPOSE
This in-vitro study aimed to evaluate the fracture resistances and failure modes of endodontically treated mandibular premolars restored with endocrowns and conventional post-core retained crowns.
MATERIALS AND METHODS
Thirty mandibular premolars were assigned into three groups (n=10): GI, intact teeth; GE, teeth with endocrowns; GC, teeth with conventional post-core supported crowns. Except for the teeth in group GI, all specimens were cut to 1.5 mm above the cementoenamel junction and endodontically treated. Both endocrowns and conventional crowns were fabricated from lithium-disilicate blocks using a CEREC 3D CAD/CAM unit. All specimens were subjected to thermocycling and then to 45° oblique compressive load until fracture occurred. The fracture resistance and failure mode of each specimen were recorded. Data were analyzed with one-way ANOVA and LSD Post Hoc Test (α=.05).
RESULTS
The fracture resistances of GE and GC were significantly lower than that of GI (P<.01), while no significant difference was found between GE and GC (P=.702). As of the failure mode, most of the specimens in GE and GC were unfavorable while a higher occurrence of favorable failure mode was presented in GI.
Endodontically treated teeth with extensive coronal hard tissue defects usually present a higher risk of biomechanical failure than vital teeth, due to the loss of structural integrity. 12345 The conventional method for restoring these teeth is to fabricate post-core supported crowns.6 This was believed to provide better reinforcement of the residual tooth structure.789 However, many studies have reported that placing a post only promotes retention of the crown and preparation for a post may weaken the residual tooth tissues, thus increasing the chance for accidental tooth fracture.101112 Moreover, to obtain a sufficient ferrule height of 1.5 - 2 mm, additional treatments such as crown lengthening methods are recommended under some circumstances, which may lead to even lower fracture resistance of the tooth-crown complex.1314
Minimally invasive preparation to preserve a maximum amount of tooth structure is considered the gold standard for restoring teeth. Endocrowns, with a decay-orientated design concept,15 have become increasingly popular because of their advantages in preserving the maximum tooth tissue, reducing the need for auxiliary retentive geometry and saving treatment time and expense as fewer operation steps are involved. Moreover, the development of dental CAD/CAM systems provides a novel means of chair-side design and automatic fabrication of all ceramic restorations, especially the ceramic endocrown that constructs both the crown and the core as a single unit.
Several in vitro studies have reported that molars and maxillary premolars with ceramic endocrowns showed better fracture resistance than those with conventional postcore supported ceramic crowns.151617 However, there were few studies about the fracture strength of endocrowns of the mandibular premolars, whose coronal and radicular geometries are quite different from their maxillary counterparts. Yet there have rarely been studies regarding the differences between the fracture resistance of intact mandibular premolars and teeth restored with endocrowns and conventional crowns. Therefore, the aim of this study was to assess the fracture resistance and failure patterns of endodontically treated mandibular premolars restored with ceramic endocrowns and conventional post-core supported ceramic crowns, using intact teeth as a control.
This study was approved by the Chinese PLA General Hospital Ethics Committee (Process Number: S2015-008-01).
Thirty human mandibular premolars, extracted for orthodontic reasons, were selected. All the premolars were of similar size and shape, selected by similar mesiodistal and buccolingual dimensions at the cementoenamel junction (CEJ) and root lengths measured with a caliper with an accuracy of 0.05 mm (Chengliang Tools Group Co. LTD, Chengdu, China), permitting a maximum deviation of 10% from the mean. After the dental plaque, calculus, and periodontal soft tissues cleaned, the teeth were stored in 0.5% chloramine solution at 4℃.
The specimens were randomly assigned into three groups (n = 10) : the intact teeth group (GI), the endocrown group (GE), and the conventional post-core supported crown group (GC).
Except for the intact teeth in GI, the crown portions of all the specimens were sectioned 1.5 mm above the CEJ and endodontic treatments were performed. The working length was visually determined by placing a #10 K-file into the canals. Root canals were instrumented with K-files # 10-20 and enlarged by nickel-titanium rotary instruments (ProTaper, Dentsply Maillefer, Tulsa, OK, USA) according to the manufacturer's instructions. After each file, the canals were rinsed with 1% NaClO. Then the root canals were obturated with laterally condensed gutta-percha (Dentsply Maillefer, Tulsa, OK, USA) and sealer (AH-Plus, Dentsply Maillefer, Tulsa, OK, USA). The specimens were kept in saline at 37℃ for one week.
In GE, the gutta-percha was removed by a small carbide bur to 7 mm below the top of the chamber walls. A flowable resin composite (Filtek Z350XT flowable, 3M ESPE, St. Paul, MN, USA) was then put to fill the canals, and 5 mm depth of the pulp chamber retention form was reserved. The cavity preparation process was subjected to elimination of the retentive areas and alignment of the axial walls. A total occlusal convergence of 2 - 5° was performed by a tapered diamond bur. The circular butt margin and a central retention cavity were prepared and smoothed according to Figure 1 and measured with the caliper.
In GC, the gutta-perchas were removed from the root canals, leaving a 3 - 5 mm apical gutta-percha seal. The root canals were enlarged with burs included in the post system. Each glass fiber post (RTD Post #1.2, St. Egreve, France) was tried in and cut to adequate length so as to keep 3 mm buried in the composite resin core. The canal walls and the exposed portion of the coronal dentin were etched and the bonding agent (Adper Single Bond Plus, 3M ESPE) was applied. The post was cemented using a dual resin cement (RelyX ARC; 3M ESPE) according to manufacturer's instructions. Then a 3 mm-high core was built up with a resin core (MutiCore flow, Ivoclar Vivadent). Each tooth was prepared with a 1.5 mm-high ferrule and a 1.0 mmwidth margin at the CEJ (Fig. 1B).
Using the CEREC 3D system (Sirona Dental Systems GmbH, Bensheim, Germany), endocrowns and conventional crowns were designed and fabricated from lithium disilicate (e.max CAD, Ivoclar Vivadent, Schaan, Liechtenstein). After the ceramic restorations being fitted and polished, the tissue surfaces of the restorations were etched with 5% hydrofluoric acid (IPS Ceramic Etching Gel, Ivoclar Vivadent, Schaan, Liechtenstein) and pretreated by a silanecoupling agent (Monobond S; Ivoclar Vivadent).
The adhesive surfaces of the teeth were etched and pretreated by dentin primer (Syntac, Ivoclar Vivadent) and dentin adhesive (Syntac, Ivoclar Vivadent). All crowns were luted with a dual cure resin cement (Variolink II, Ivoclar Vivadent) according to manufacturer's instructions.
A 0.2 mm layer of polyether impression material (Impregum Penta Soft, 3M ESPE) was applied on the root surface. After that, all specimens were embedded in self-cured acrylic resin (Shanghai Dental Material Company, shanghai, China), leaving a height of 2 mm apically to the CEJ, then stored in saline at 37℃ for a week.
All specimens were subjected to 5000 cycles of thermocycling at 5℃ to 55℃ for 30 seconds dwell time in a thermal cycling machine (C-501F, Will laboratory supplies LTD, Suzhou, China). Subsequently, the specimens were stored in a humid environment for 24 hours before testing.
Each specimen was fixed in a universal testing machine (WDW-100, Changchun, China), with a stainless-steel sphere (5 mm in diameter) contacting the lingual plane of the buccal cusps. Then a load was applied at a cross-head speed of 1.0 mm/min and at an angle of 45° to the long axis of the tooth until fracture occurred (Fig. 2). The fracture resistance of each specimen was recorded in Newton, and its fracture mode was classified according to the following patterns:1819
Favorable failures: repairable fractures of the teeth/restorations above the level of bone simulation;
Unfavorable failures: non-repairable fractures below the level of bone simulation.
Data were statistically analyzed with SPSS 17.0 (SPSS Inc, Chicago, IL, USA). One-way analysis of variance (ANOVA) and LSD Post Hoc Test (α = .05) were used to detect the statistically significant variations among the groups.
The means of fracture resistance of the three groups are presented in Table 1. GI revealed the highest mean fracture resistance compared with those of GE and GC, and the differences were of statistical significance (P < .01). No significant difference was found between GE and GC (P = .702) (Table 2).
The failure modes are demonstrated in Table 3. Most failure modes in GI were favorable, while most failure modes in GE and GC were unfavorable. In group GI, most fracture areas were above the level of bone simulation on the buccal side. On the other hand, in group GE and GC, majority fracture lines invaded into the bone simulation areas on the buccal side.
Advances in CAD/CAM all-ceramic materials and adhesive dentistry, especially regarding the high bonding capacity of the lithium-disilicate ceramics, provide highly conservative approaches for endodontically treated teeth with better mechanical character, biocompatibility, and efficiency. The endocrown is built on a decay-orientated preparation to preserve a maximum amount of tooth tissue for bonding instead of extensive preparation for retention. In previous studies, there has been considerable disagreement about the endocrown as an available method for premolars. Some studies found that endocrowns for endodontically treated maxillary premolars had higher fracture resistance than the conventional restorations,1617 while others reported that endocrowns appeared inadequate for premolars compared with conventional crowns.2021 Moreover, there has not been any recommendation about the validity of the endocrown for endodontically treated mandibular premolars, which have quite different anatomic forms from maxillary premolars.
This study showed that the fracture resistances were 479.1 N for GE and 510.1 N for GC, and ANOVA analysis demonstrated no significant difference between them. Compared with maxillary premolar, the mandibular premolar had a smaller crown, which caused insufficient resistance forms of residual tooth tissue. Sufficient bonding area is crucial for the retention and stability of endocrown. A smaller pulp chamber area of mandibular premolars leads to a decreased bonding area and therefore to the decline of retention force. Moreover, the narrow neck of mandibular premolar is more obvious and the diameter of the root is smaller than that of the maxillary premolar, which might lead to poor stress transmission patterns from crown to root. In addition, for fiber post-core retained conventional crowns, the post inserted into the root canal connects the crown and root as a whole, and the post enhances the retention of the restoration. The elastic modulus of the fiber post close to that of dentin is conducive to the stress concentration. 22 All these might be the reasons that the endocrown doesn't show a higher mean fracture resistance value than conventional crown in mandibular premolars.
Most of the failure modes of both the endocrown and the conventional groups of mandibular premolars in our study were unfavorable. This was consistent with a report about maxillary premolars.16 It can be inferred that in most situations, the mechanical failure of restored premolars, including both maxillary and mandibular premolars, whether by endocrowns or by conventional crowns, may mainly due to the weakening of the residual tooth structures instead of ceramic materials and resin cement materials.
The fracture resistance and failure modes are associated with many factors. The loading methods might have been one of the reasons for the differences between our study and previous researches. In our study, the load was applied at an angle of 45° to the long axis of the tooth to simulate occlusal forces in both lateral and long-axis directions, while an axial loading was adopted in most previous studies. Instead of the middle fossa, our loading position was on the lingual slope of the buccal cusp, which was the key occlusal contact area of mandibular premolars. The morphological characters of the mandibular premolars, such as a sharply narrowed neck at CEJ, a narrower bucco-lingual width compared with the maxillary premolars, may also contribute to the divergent results shown in our study.
Our study also showed that the fracture resistance of the tooth-restoration complexes was lower than that of intact mandibular premolars. Therefore, it is important to reduce the chance of fracture failure when making either endocrown-morphological design or conventional design on mandibular premolars. Reducing the height and inclines of the cusps and minimizing the mesio-distal and bucco-lingual width are recommended. Adopting polyceramics or other materials, with relatively low modulus of elasticity and increasing strength, may also be options to reduce the risk of unfavorable failure under certain circumstances.
Under the condition of this in-vitro study, we discovered that the endocrown and the conventional glass fiber postcore retained ceramic crown showed similar fracture resistance, which was significantly lower than that of the intact mandibular premolar. However, clinical long-term follow-up studies are required to support these findings.
Figures and Tables
Fig. 1
Schematic representation of the tooth preparation. Schematic representation of the tooth preparation. (A) Group GE: endocrown preparation: the depth of central retention cavity was 5 mm from the cervical margin, margin width was 2 mm; (B) Group GC: conventional preparation with core height of 3 mm, height ferrule of 1.5 mm, shoulder width of 1 mm, and apical sealing of 5 mm.
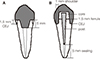
Table 1
Mean fracture resistance values (in N) for the GI, GE, GC groups of mandibular premolars

Group | N | Mean | SD | 95% CI | Max | Min | |
---|---|---|---|---|---|---|---|
Lower | Upper | ||||||
GI | 10 | 997.1 | 166.3 | 878.2 | 1116.0 | 1269.5 | 740.0 |
GE | 10 | 479.1 | 180.6 | 349.9 | 608.2 | 764.0 | 267.5 |
GC | 10 | 510.1 | 191.0 | 373.5 | 646.7 | 882.0 | 219.0 |
Table 2
Results of LSD Post Hoc Test from multiple comparison of fracture resistance of different groups for mandibular premolars
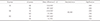
(I) group | (J) group | Mean difference (I - J) | Standard error | Significance |
---|---|---|---|---|
GI | GE | 518.050* | 80.294 | .000 |
GC | 487.000* | .000 | ||
GE | GI | −518.050* | .000 | |
GC | -31.050 | .702 | ||
GC | GI | −487.000* | .000 | |
GE | 31.050 | .702 |
References
1. Reeh ES, Messer HH, Douglas WH. Reduction in tooth stiffness as a result of endodontic and restorative procedures. J Endod. 1989; 15:512–516.
2. Huang TJ, Schilder H, Nathanson D. Effects of moisture content and endodontic treatment on some mechanical properties of human dentin. J Endod. 1992; 18:209–215.
3. Linn J, Messer HH. Effect of restorative procedures on the strength of endodontically treated molars. J Endod. 1994; 20:479–485.
4. Assif D, Gorfil C. Biomechanical considerations in restoring endodontically treated teeth. J Prosthet Dent. 1994; 71:565–567.
5. Soares PV, Santos-Filho PC, Martins LR, Soares CJ. Influence of restorative technique on the biomechanical behavior of endodontically treated maxillary premolars. Part I: fracture resistance and fracture mode. J Prosthet Dent. 2008; 99:30–37.
6. Bitter K, Kielbassa AM. Post-endodontic restorations with adhesively luted fiber-reinforced composite post systems: a review. Am J Dent. 2007; 20:353–360.
7. Stern N, Hirschfeld Z. Principles of preparing endodontically treated teeth for dowel and core restorations. J Prosthet Dent. 1973; 30:162–165.
8. Hirschfeld Z, Stern N. Post and core-the biomechanical aspect. Aust Dent J. 1972; 17:467–468.
9. Guzy GE, Nicholls JI. In vitro comparison of intact endodontically treated teeth with and without endo-post reinforcement. J Prosthet Dent. 1979; 42:39–44.
10. Sorensen JA, Engelman MJ. Effect of post adaptation on fracture resistance of endodontically treated teeth. J Prosthet Dent. 1990; 64:419–424.
11. Schwartz RS, Robbins JW. Post placement and restoration of endodontically treated teeth: a literature review. J Endod. 2004; 30:289–301.
12. Cheung W. A review of the management of endodontically treated teeth. Post, core and the final restoration. J Am Dent Assoc. 2005; 136:611–619.
13. Sivers JE, Johnson WT. Restoration of endodontically treated teeth. Dent Clin North Am. 1992; 36:631–650.
14. Gegauff AG. Effect of crown lengthening and ferrule placement on static load failure of cemented cast post-cores and crowns. J Prosthet Dent. 2000; 84:169–179.
15. Biacchi GR, Basting RT. Comparison of fracture strength of endocrowns and glass fiber post-retained conventional crowns. Oper Dent. 2012; 37:130–136.
16. Chang CY, Kuo JS, Lin YS, Chang YH. Fracture resistance and failure modes of CEREC endo-crowns and conventional post and core-supported CEREC crowns. J Dent Sci. 2009; 4:110–117.
17. Lin CL, Chang YH, Chang CY, Pai CA, Huang SF. Finite element and Weibull analyses to estimate failure risks in the ceramic endocrown and classical crown for endodontically treated maxillary premolar. Eur J Oral Sci. 2010; 118:87–93.
18. Carvalho AO, Bruzi G, Anderson RE, Maia HP, Giannini M, Magne P. Influence of Adhesive Core Buildup Designs on the Resistance of Endodontically Treated Molars Restored With Lithium Disilicate CAD/CAM Crowns. Oper Dent. 2016; 41:76–82.
19. Salameh Z, Sorrentino R, Papacchini F, Ounsi HF, Tashkandi E, Goracci C, Ferrari M. Fracture resistance and failure patterns of endodontically treated mandibular molars restored using resin composite with or without translucent glass fiber posts. J Endod. 2006; 32:752–755.
20. Bindl A, Mörmann WH. Clinical evaluation of adhesively placed Cerec endo-crowns after 2 years-preliminary results. J Adhes Dent. 1999; 1:255–265.
21. Bernhart J, Bräuning A, Altenburger MJ, Wrbas KT. Cerec3D endocrowns--two-year clinical examination of CAD/CAM crowns for restoring endodontically treated molars. Int J Comput Dent. 2010; 13:141–154.
22. Ma J, Miura H, Okada D, Yusa K. Photoelastic stress analysis of endodontically treated teeth restored with different post systems: normal and alveolar bone resorption cases. Dent Mater J. 2011; 30:806–813.