Abstract
PURPOSE
The aim of this study was to evaluate the fracture resistance and fracture behavior of monolithic zirconia crowns in accordance with the preparation design and aging simulation method.
MATERIALS AND METHODS
An upper first molar was prepared sequentially with three different preparation designs: shoulderless preparation, 0.4 mm chamfer and 0.8 mm chamfer preparation. For each preparation design, 30 monolithic zirconia crowns were fabricated. After cementation on Cr-Co alloy dies, the following artificial aging procedures were performed: (1) thermal cycling and mechanical loading (TCML): 5000 cycles of thermal cycling 5℃–55℃ and chewing simulation (1,200,000 cycles, 50 N); (2) Low Temperature Degradation simulation (LTD): autoclave treatment at 137℃, 2 bar for 3 hours and chewing simulation; and (3) no pre-treatment (control group). After artificial aging, the crowns were loaded until fracture.
RESULTS
The mean values of fracture resistance varied between 3414 N (LTD; 0.8 mm chamfer preparation) and 5712 N (control group; shoulderless preparation). Two-way ANOVA analysis showed a significantly higher fracture loads for the shoulderless preparation, whereas no difference was found between the chamfer preparations. In contrast to TCML, after LTD simulation the fracture strength of monolithic zirconia crowns decreased significantly.
Yttrium-stabilized tetragonal zirconia ceramic (Y-TZP) has proven to be a high-performance material and has found use as a core material for virtually any type of fixed restoration, due to its superior mechanical properties as the result of an inherent transformation toughening mechanism.1 In comparison to other metal-free core materials for fixed dental prostheses (FDPs), Y-TZP exposes the highest initial and most favorable long-term stability.2 In addition, Y-TZP presents good biocompatibility and tooth-like optical properties.3
Analogous to metal-ceramic crowns, zirconia frameworks are veneered with translucent feldspathic or glass ceramic materials for esthetic reasons. In laboratory studies of veneered zirconia single crowns, the reliability was mainly limited to veneer chip-off fractures.45 In clinical applications, the veneering ceramic has also been revealed to be the weakest link in zirconia-supported reconstructions. A systematic review showed that the frequency of chipping was statistically significantly higher for zirconia-supported FDPs when compared to metal-supported FDPs6; however, there was a strong study effect. Different modifications have been sought to address the problem, both in the firing process and in the technical fabrication of the FDPs.78
A very simple method to avoid chipping is to omit the veneering porcelain and mill zirconia to full-contour. Several manufacturers offer this approach; they have improved the aesthetics of the zirconia materials mainly by reducing the opacity of the material and by addition of colouring pigments. In addition to improved fracture-resistance, further advantages of this approach compared to conventional zirconia-based restorations can be expected, such as reduction of the technique sensitivity and production costs by automatic designing and milling to full contour using computer aided desing (CAD)/computer aided manufacturing (CAM) technology. It might also be assumed that, by omitting the veneering, a more solid framework can be made and a conservative preparation similar to full-cast metal-alloy restorations can be performed.
For zirconia restorations, different preparation designs have been suggested 3910; in most cases, a chamfer or a rounded shoulder finishing line with an occlusal reduction of 1.5 mm and axial reduction of 1.0–1.5 mm is recommended. Many contradictory results concerning the influence of preparation design and material thickness on the strength of all-ceramic crowns have been reported. In different studies, no significant differences for the fracture resistance of leucite-reinforced ceramic11 and glass-infiltrated alumina12 molar crowns with different preparation designs were found. In contrast, in a study on zirconia frameworks with a wall thickness of 0.4 mm, Beuer et al.13 indicated significant differences in fracture strength among the different preparation designs. However, the authors decided not to veneer the copings, as they considered it practically impossible to achieve equal veneering layers with standardized dimensions. Vult von Steyern14 found, in an in vitro study, that the fracture load for aluminum oxide and zirconia-based three-unit FDPs with a shoulder preparation was significantly higher than that of the deep chamfer preparation. However, on full-contour zirconia restorations, there is still a lack of scientific data.
Several concerns regarding the use of monolithic zirconia restorations have been raised in the literature. In addition to the possible high abrasiveness of the non-veneered material toward enamel,1516 a limiting factor could be the aging of Y-TZP, due to its potential sensitivity to Low Temperature Degradation (LTD).17 For Y-TZP, LTD is a process caused by the progressive spontaneous transformation of the tetragonal phase into the monoclinic phase in the presence of water,18 which may lead to surface degradation and a decrease in mechanical properties. At present, limited data are available concerning the effects of aging on zirconium dioxide used for oral rehabilitation.19202122 In full-contour restorations, the Y-TZP ceramic is directly exposed to mechanical and thermal stress, as well as to the wetness of the oral environment, so that the excellent initial mechanical properties could be affected in the long-term.
The working hypothesis of this study is that the fracture resistance of monolithic Y-TZP single molar crowns will be influenced by (1) the type of preparation design and (2) the artificial aging procedures.
All preparation procedures were performed sequentially on an acrylic maxillary right molar (Frasaco, Tettnang, Germany). The initial preparation form was a shoulderless preparation with an occlusal reduction of 0.8 mm. Impressions of the prepared tooth were made with an A-silicone (Adisil Blau, Siladent Dr. Böhme und Schöps, Goslar, Germany). After that, the tooth was prepared with a 0.4 mm chamfer margin, followed by a chamfer preparation with 0.8 mm circumferential reduction and replicas were made. (Fig. 1) The preparation finishing line was marked with permanent marker (Edding 3000, Ahrensburg, Germany) and special care was taken to ensure that the markings remained intact. For the slight chamfer and the chamfer preparation designs, no additional modifications of the occlusal surface were made, except for rounding sharp edges. The preparation angle of the axial walls was set to 8 degrees by means of a parallel milling machine (Degussa F1, DeguDent, Hanau, Germany). The preparation depth and material reduction were controlled by a temporary crown (Protemp 3 Garant, 3M ESPE, Seefeld, Germany) and a crown gauge (Iwarson Gauge, Carl Martin, Solingen, Germany).
For each preparation design, the replicas were cast in super-hard plaster (Everest Rock, KaVo, Biberach, Germany) and scanned with a 3D laser scanner (3shape D700, Wieland, Pforzheim, Germany). Crowns with identical contours and uniform cement layer thickness of 50 µm were designed for each preparation form using CAD software (DentalDesigner, Wieland, Germany). To ensure constant thickness of the occlusal walls, a simplified, semi-anatomical occlusal design was chosen (Fig. 2). The generated data were sent via the Internet to the manufacturer (Wieland), who produced and delivered the monolithic zirconia crowns [ZenoTec Zr Blank, Wieland: (ZrO2 + HfO2) 94%, (Y2O3) 5%, (Al2O3) <1%, other oxides <1%)].
Duplicate molds of the plaster dies were made with an addition curing silicone (Adisil Blau) and used to produce wax patterns for each crown design. After the fit of the wax dies to the crowns was controlled, 30 metal dies were cast in Cr-Co alloy (Remanium 800, Dentaurum, Ispringen, Germany) for each type of preparation. The metal dies were embedded in an auto-polymerizing resin (Paladur, Heraeus Kulzer, Hanau, Germany). After being cast, the metal dies were divested and cleaned. The dies were fitted to the crowns by an experienced dental technician using water-cooling with fine and superfine finishing diamond burs (Brasseler, Lemgo, Germany). The precision of fit and marginal adaptation of the crowns were inspected visually and by light-body silicone (Fit Test C&B, Voco, Cuxhaven, Germany). After adaptation, polishing rubber wheels were used (Eveflex Polisher, EVE Ernst Vetter GmbH, Pforzheim, Germany) to smooth out sharp edges and junctions of the metal dies. All crowns were subjected to airborne-particle abrasion with 50 µm aluminum oxide using 0.4 MPa air pressure (Rocatector delta, 3M ESPE®, Seefeld), cleaned with air-steam and 70% alcohol and conventionally cemented to the metal dies with glass ionomer cement (KetacCem, 3M ESPE) under a static load of 50 N for 10 minutes in a standardized loading device Z1445 (Zwick, Ulm, Germany).
The cemented crowns of each preparation design were randomly divided into 3 groups according to the different pre-loading procedures from the following test protocol:
Group 1: Control group
The specimens of the control group (n = 30) were stored dry prior to fracture loading.
Group 2: TThermal Cycling and Mechanical Loading (TCML)
The cemented crowns (n = 30) were subjected to 5000 cycles of thermocycling at temperatures between 5℃ and 55℃. Then, they were mounted in a commercially available dual-axis mastication simulator (Willytech, Munich, Germany) and were subjected to 1,200,000 masticatory cycles of unidirectional antagonist movements with a frequency of 1.2 Hz and an applied force of 50 N. Force was applied with a loading stylus placed on the occlusal surfaces of the crowns alongside the tooth axis.
Group 3: LTD simulation and chewing simulation
To simulate the effect of LTD, the crowns from group 3 were subjected to 3 hours of autoclave treatment at 134℃ and 2 bar (Siemens Validator, Siemens AG, Bensheim, Germany), corresponding roughly to 10 years under in vivo conditions at 37℃.23
In total, 90 specimens were loaded until fracture occurred in a universal testing machine (Type 1445, Zwick, Ulm, Germany). The load was vertically applied with a 4-mm-diameter stainless steel ball placed at the center of the occlusal surfaces of the crowns and a crosshead speed of 0.5 mm/min as recommended in previous studies.3424 A thin (0.5 mm) piece of polyethylene foil (Erkodent, Erich Koop GmbH, Pfalzgrafenweiler, Germany) was placed between the loading piston and the occlusal surface of the specimen to avoid loading stress peaks on the ceramic surface. 13 Fracture was defined as the occurrence of visible cracks in combination with load drops in the stress-strain diagram and acoustic events, and the fracture load was recorded with the appropriate software (TestXpert, Zwick).
Statistical evaluation was performed with SPSS for Windows, Release 17.1 (SPSS Inc., Chicago, IL, USA). Fracture strength values were positively tested for normal distribution (Kolmogorov-Smirnov test) and equality of variances (Levene test). Differences in means of each group were analyzed by two-way ANOVA. Post-hoc comparisons were performed with the Bonferroni correction. Level of significance was set at 0.05.
A qualitative fractography analysis was performed on selected specimens by standard SEM techniques (Strata DB 235, FEI Company, Hillsboro, OR, USA).
The results of the fracture-loading test are presented as box plots in Fig. 3. The highest mean fracture loads were observed for the shoulderless preparation: 5712 N (SD, 758) in the control group, 5487 N (SD, 310) for the thermocycled and 4799 N (SD, 499) for the autoclaved specimens. The control group showed the highest standard deviations; the LTD simulation group, the lowest. The lowest mean fracture load of 3414 N (SD, 457) was observed for chamfer preparation after LTD (Table 1).
Two-way ANOVA indicated significant influences of the factors "preparation form" and "pre-loading treatment" on the fracture loads, as well as the correlation between them (P < .001). Post-hoc analysis showed a significantly higher fracture load for the shoulderless preparation, whereas no difference between chamfer preparations was found. In contrast to thermocycling, LTD simulation showed a significant influence on the fracture loads.
Fig. 4 shows a typical SEM image of a fracture surface. All observed fractures initiated at the tensile surface under the loading point and propagated to the periphery of the specimens. No porosity or flaws were detected in the fracture areas.
The results of this in vitro study confirmed the hypothesis, that the fracture strength of monolithic Y-TZP single molar crowns was influenced by the preparation design and the artificial aging procedure. The reduction of tooth substance for the preparation forms used in this study was considerably less than the manufacturer's recommendations for conventional zirconia-based restorations (1.2 mm circular and 1.5 mm occlusal). A less-invasive preparation design is an important requirement for a modern restoration material. The differences in substance loss are also reflected in the calculated volumes for the 3 different restorations. While the crown, generated for the shoulderless preparation had a volume of 244 mm3, for the 0.4 mm chamfer preparation the crown volume was 280 mm3 and for the 0.8mm chamfer 298 mm3. These values can be transferred to the tooth structure loss, as the occlusal relief, as well as the preparation finishing line were constant and preparations were performed sequentially on the same acrylic molar.
The highest mean fracture loads were observed for the shoulderless preparation. However, the shoulderless preparation cannot be recommended due to periodontal considerations. 25 From this point of view, as increased material thickness did not show a significant impact on loading capacity among the chamfer preparation groups, a minimal invasive preparation design should be determined the optimal choice.
A clear interpretation of the literature results on the influence of preparation design on the mechanical properties of all-ceramic restorations is difficult mainly due to the big variety of experimental settings for fracture strength measurement as well as the used materials. In different studies, the tested all-ceramic crown consisted of a high-strength ceramic framework, veneered with porcelain. In these cases, the effect of the veneering material on the fracture strength of the restorations should be taken into consideration. Furthermore, the manual production of veneering porcelain for identical crowns with standardized dimensions is practically impossible. Using CAD/CAM techniques and the preparation method described in the present work, a more systematic approach is applicable.
In this study, the Cr-Co dies were manufactured after the crowns were fabricated. From a clinical point of view, duplicating the prepared abutment in metal and making impressions from the metal dies would be the more realistic approach. However, the present manufacturing sequence and the CAD/CAM production ensured identical geometries for the crowns within the groups.
The final surface treatment of monolithic zirconia restorations is an important issue. Currently, there is a lack evidence-based recommendation regarding the techniques for achieving optimal surface quality. Polishing and glazing are the common methods of creating a smooth surface on ceramic restorations. Polishing zirconia is elaborate and time-consuming. Only a few methods for polishing zirconia have been suggested in the literature.262728 A polishing kit for dental zirconia ceramics has recently been introduced (Glidewell Laboratories, Newport Beach, CA, USA). Manufacturers recommend a final glazing of the restoration; however, glaze is lost by contact wear with clinical service time, and the effect of surface roughness becomes a self-limiting factor. 2930 Different finishing methods have also been reported to have a significant influence on the mechanical properties of zirconia.31 To have identical specimens the crowns used in this study were tested "as sintered", with no further finishing procedures performed. If necessary, adaptation of the crowns was performed by modifying the metal dies, to avoid machine-induced defects in the ceramic surface.
For all-ceramic crowns, the experimental settings have a significant impact on the fracture strength measured. Rosentritt et al.32 showed that the fracture resistance of all-ceramic crowns is dependent on the modulus of elasticity of the supporting material and the periodontal ligament simulation. A significantly higher fracture load was measured for crowns fixed on Co-Cr-Mo alloy materials than for crowns on human teeth. The artificial periodontium also reduced the fracture resistance of the tested all-ceramic restorations. Therefore, natural teeth with artificial periodontium as abutments would have probably resulted in lower values for the fracture strength of monolithic zirconia crowns. In contrast, the use of natural teeth leads to a wider distribution of fracture results and restricts their significance. 32 For that reason, the authors decided to use standardized metal dies to allow a better reproducibility and comparability with existing studies.13 However, all laboratory studies present limitations, and the results should thus be interpreted carefully.
In a study on zirconia copings with a wall thickness of 0.4 mm with different preparation designs without artificial aging and using the same test conditions, Beuer et al.13 attained significantly higher fracture strength with the shoulderless preparation. The authors explained the favorable results achieved with the shoulderless preparation by the stress distribution pattern during loading. They assumed that, under increasing load, the crown could slide down the axial wall of the shoulderless die without being limited by the margin. However, the cross-sections of Finite Element Analysis, conducted for the different crown designs could not show any influence of the preparation design on the stress distribution. The maximum tensile stress concentration was on the occlusal surface of the crowns; no differences in stress distribution could be detected for the marginal regions of the different crown designs.
The fracture loads determined by Beuer et al. are far lower than the present results, but still well above the clinically required strength of 1000 N for zirconia.2 The superior results from the present study could be explained by the different geometry of the monolithic crowns and especially by the 2-fold thickness of the occlusal surface. Therefore, it would be interesting to determine clinically relevant limits for occlusal dimensions, to allow minimally invasive preparation forms.
Different laboratory simulations have been used for pre-clinical investigations of all-ceramic restorations33343536 in attempts to predict catastrophic failures. The TCML method used in this study has been widely applied in mechanical testing for simulating approximately 5 years of oral service. In a study estimating the relevance of laboratory simulation on zirconia FPDs, Rosentritt et al.32 indicated that the explanatory power of this kind of simulation might provide sufficient estimations of the survival rate. In this case, all observed failures were chippings of the veneering ceramics; no framework fractures were determined. In the present study, TCML did not significantly decrease the fracture strength of the zirconia crowns. These results are in accordance with the findings of Senyilmaz et al.37 on the influence of thermal and mechanical fatigue loading on the fracture resistance of all-ceramic posterior crowns.
The low-temperature aging process can influence different aspects of the long-term clinical performance of monolithic zirconia. The t-m transformation causes a surface uplift,23 so that the rough surface could lead to increased contact wear during function. Furthermore, microcracking can occur2338; the microcracks can grow slowly under mechanical stress, leading to premature critical fracture. For the highly translucent zirconia used for monolithic restorations, microcracking could also lead to aesthetic deterioration. 39 In this study, no signs of surface degradation were detected after LTD and chewing simulation.
One of the main factors affecting the aging phenomenon in Y-TZP is the Yttrium content. High Yttrium dopant content has been reported to increase degradation resistance.40 Since the material used in this study had high Yttrium content (5 mol%), a low susceptibility to LTD might be expected. However, LTD simulation significantly decreased the fracture strength of the test specimens. This fact underlines the need for long-term clinical studies to assess the clinical performance of nonveneered Y-TZP materials for dental purposes.
Within the limitations of this in vitro study, the following conclusions could be drawn:
The monolithic crowns tested in this study showed generally high fracture load values regardless of the type of tooth preparation and aging simulation design.
While LTD simulation caused a significant decrease of the fracture load for all groups of different preparation depths, thermocycling did not influence the fracture loads.
Regardless of artificial aging method, the monolithic zirconia crowns with circumferential shoulderless preparation had a significantly higher fracture load than other groups.
Figures and Tables
Fig. 1
Preparation designs used in the study: overlay of scans of the three different preparation designs.
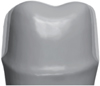
Fig. 2
Monolithic zirconia crown, cemented on the metal die with resin socket. (A) Vestibular view. (B) Occlusal view: semi-anatomical occlusal design.
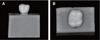
Table 1
Results of the fracture-loading test in N (standard deviation)

References
1. Raigrodski AJ. Contemporary materials and technologies for all-ceramic fixed partial dentures: a review of the literature. J Prosthet Dent. 2004; 92:557–562.
2. Tinschert J, Natt G, Mohrbotter N, Spiekermann H, Schulze KA. Lifetime of alumina- and zirconia ceramics used for crown and bridge restorations. J Biomed Mater Res B Appl Biomater. 2007; 80:317–321.
3. Manicone PF, Rossi Iommetti P, Raffaelli L. An overview of zirconia ceramics: basic properties and clinical applications. J Dent. 2007; 35:819–826.
4. Sundh A, Molin M, Sjogren G. Fracture resistance of yttrium oxide partially-stabilized zirconia allceramic bridges after veneering and mechanical fatigue testing. Dent Mater. 2005; 21:476–482.
5. Coelho PG, Silva NR, Bonfante EA, Guess PC, Rekow ED, Thompson VP. Fatigue testing of two porcelain-zirconia all-ceramic crown systems. Dent Mater. 2009; 25:1122–1127.
6. Heintze SD, Rousson V. Survival of zirconia- and metal-supported fixed dental prostheses: a systematic review. Int J Prosthodont. 2010; 23:493–502.
7. Swain MV. Unstable cracking (chipping) of veneering porcelain on all-ceramic dental crowns and fixed partial dentures. Acta Biomater. 2009; 5:1668–1677.
8. Taskonak B, Borges GA, Mecholsky JJ, Anusavice KJ, Moore BK, Yan J. The effects of viscoelastic parameters on residual stress development in a zirconia/glass bilayer dental ceramic. Dent Mater. 2008; 24:1149–1155.
9. McLaren EA, White SN. Glass-infiltrated zirconia/alumina based ceramic for crowns and fixed partial dentures. Pract Periodontics Aesthet Dent. 1999; 11:985–994.
10. Goodacre CJ, Campagni WV, Aquilino SA. Tooth preparations for complete crowns: an art form based on scientific principles. J Prosthet Dent. 2001; 85:363–376.
11. Fenske C, Jarren MP, Sadat-Khhonsari MR. Fracture strength of full ceramic crowns in dependence of the preparation width. Dtsch Zahnarztl Z. 1999; 54:732–734.
12. Meier M, Fischer H, Richter EJ, Maier HR. Influence of different preparation forms on the fracture resistance of full-ceramic molar crowns. Dtsch Zahnarztl Z. 1995; 50:295–299.
13. Beuer F, Edelhoff D, Gernet W, Naumann M. Effect of preparation angles on the precision of zirconia crown copings fabricated by CAD/CAM system. Dent Mater J. 2008; 27:814–820.
14. Vult von Steyern P. All-ceramic fixed partial dentures. Studies on aluminum oxide- and zirconium dioxide-based ceramic systems. Swed Dent J Suppl. 2005; (173):61–69.
15. Kern M. Clinical long-term survival of two-retainer and single-retainer all-ceramic resin-bonded fixed partial dentures. Quintessence Int. 2005; 36:141–147.
16. Ghazal M, Kern M. The influence of antagonistic surface roughness on the wear of human enamel and nanofilled composite resin artificial teeth. J Prosthet Dent. 2009; 101:342–349.
17. Kobayashi K, Kuwajima H, Masaki T. Phase change and mechanical properties of ZrO2-Y2O3 solid electrolyte after aging. Solid State Ionics. 1981; 3:489–493.
18. Chevalier J, Cales B, Drouin JM. Low temperature aging of Y-TZP ceramics. J Am Ceram Soc. 1999; 82:2150–2154.
19. Lughi V, Sergo V. Low temperature degradation -aging- of zirconia: a critical review of the relevant aspects in dentistry. Dent Mater. 2010; 26:807–820.
20. Ardlin I. Transformation-toughened zirconia for dental inlays, crowns and bridges: chemical stability and effect of low-temperature aging on flexural strength and surface structure. Dent Mater. 2002; 18:590–595.
21. Papanagiotou HP, Morgano SM, Giordano RA, Pober R. In vitro evaluation of low-temperature aging effects and finishing procedures on the flexural strength and structural stability of Y-TZP dental ceramics. J Prosthet Dent. 2006; 96:154–164.
22. Kim JW, Covel NS, Guess PC, Rekow ED, Zhang Y. Concerns of hydrothermal degradation in CAD/CAM zirconia. J Dent Res. 2010; 89:91–95.
23. Deville S, Chevalier J, Dauvergne C, Fantozzi G, Bartolome JF, Moya JS, Torrecillas R. Microstructural investigation of the aging behavior of (3Y-TZP)-Al2O3 composites. J Am Ceram Soc. 2005; 88:1273–1280.
24. Snyder MD, Hogg KD. Load-to-fracture value of different all-ceramic crown systems. J Contemp Dent Pract. 2005; 6:54–63.
25. Lang NP. Periodontal considerations in prosthetic dentistry. Periodontol. 2000; 9:118–131.
26. Preis V, Schmalzbauer M, Bougeard D, Schneider-Feyrer S, Rosentritt M. Surface properties of monolithic zirconia after dental adjustment treatments and in vitro wear simulation. J Dent. 2015; 43:133–139.
27. Akar GC, Pekkan G, Cal E, Eskitascioglu G, Ozcan M. Effects of surface-finishing protocols on the roughness, color change, and translucency of different ceramic systems. J Prosthet Dent. 2014; 112:314–321.
28. Kou W, Molin M, Sjogren G. Surface roughness of five different dental ceramic core materials after grinding and polishing. J Oral Rehabil. 2006; 33:117–124.
29. Monasky GE, Taylor DF. Studies on the wear of porcelain, enamel, and gold. J Prosthet Dent. 1971; 25:299–306.
30. Oh WS, Delong R, Anusavice KJ. Factors affecting enamel and ceramic wear: a literature review. J Prosthet Dent. 2002; 87:451–459.
31. Kosmac T, Oblak C, Jevnikar P, Funduk N, Marion L. The effect of surface grinding and sandblasting on flexural strength and reliability of Y-TZP zirconia ceramic. Dent Mater. 1999; 15:426–433.
32. Rosentritt M, Behr M, van der Zel JM, Feilzer AJ. Approach for valuating the influence of laboratory simulation. Dent Mater. 2009; 25:348–352.
33. DeLong R, Douglas WH. An artificial oral environment for testing dental materials. IEEE Trans Biomed Eng. 1991; 38:339–345.
34. Krejci I, Reich T, Lutz F, Albertson M. In-vitro test method for evaluation of dental restorations. Schweiz Monatsschr Zahnmed. 1990; 100:8–12.
35. Teoh SH, Ong LF, Yap AU, Hastings GW. Bruxing-type dental wear simulator for ranking of dental restorative materials. J Biomed Mater Res. 1998; 43:175–183.
36. Gale MS, Darvell BW. Thermal cycling procedures for laboratory testing of dental restorations. J Dent. 1999; 27:89–99.
37. Senyilmaz DP, Canay S, Heydecke G, Strub JR. Influence of thermomechanical fatigue loading on the fracture resistance of all-ceramic posterior crowns. Eur J Prosthodont Restor Dent. 2010; 18:50–54.
38. Lee JK, Kim H. Surface crack initiation in 2Y-TZP ceramics by low temperature aging. Ceram Int. 1994; 20:413–418.
39. Lance MJ, Vogel EM, Reith LA, Cannon RW. Low-temperature aging of zirconia ferrules for optical connectors. J Am Ceram Soc. 2001; 84:2731–2733.
40. Zhenglan L, Danyu J, Lei H, Guoqiang Z, Qiang L, Cheng Z. Low temperature degradation of yttria stabilized zirconia. Key Eng Mater. 2007; 336-338:1188–1189.