Abstract
PURPOSE
To compare the shear bond strength of various veneering materials to grade II commercially pure titanium (CP-Ti).
MATERIALS AND METHODS
Thirty specimens of CP-Ti disc with 9 mm diameter and 10 mm height were divided into three experimental groups. Each group was bonded to heat-polymerized acrylic resin (Lucitone 199), porcelain (Triceram), and indirect composite (Sinfony) with 7 mm diameter and 2 mm height. For the control group (n=10), Lucitone 199 were applied on type IV gold alloy castings. All samples were thermocycled for 5000 cycles in 5-55℃ water. The maximum shear bond strength (MPa) was measured with a Universal Testing Machine. After the shear bond strength test, the failure mode was assessed with an optic microscope and a scanning electron microscope. Statistical analysis was carried out with a Kruskal-Wallis Test and Mann-Whitney Test.
RESULTS
The mean shear bond strength and standard deviations for experimental groups were as follows: Ti-Lucitone 199 (12.11 ± 4.44 MPa); Ti-Triceram (11.09 ± 1.66 MPa); Ti-Sinfony (4.32 ± 0.64 MPa). All of these experimental groups showed lower shear bond strength than the control group (16.14 ± 1.89 MPa). However, there was no statistically significant difference between the Ti-Lucitone 199 group and the control group, and the Ti-Lucitone 199 group and the Ti-Triceram group. Most of the failure patterns in all experimental groups were adhesive failures.
When metal-resin implant fixed complete denture were first introduced, type III gold alloys were recommended for superstructure and have been generally used until today for their biocompatibility, corrosion resistance and high strength. However, the price of gold alloy has increased significantly, and the passive fit of the casting method remains questionable. Therefore, grade II commercially pure titanium (CP-Ti) was introduced as an alternative in recent years due to having the advantages of gold alloy, and moreover having low density (4.5 g/cm3), lower cost and accurate fit.1,2,3
The titanium frameworks manufactured by CAD/CAM (computer-aided design/computer-aided manufacturing) have an accurate fit.4,5,6 But retention forms, such as beads, hooks or nail heads to enhance the bond strength cannot be mill-performed due to the limitations of CAD/CAM procedure. Therefore, optimum adherence between titanium and veneering material is the prerequisite for clinical success. There are many articles that evaluated the bond strength between veneering materials and CP-Ti,7,8,9,10 but few comparative studies among various veneering materials bonding to titanium are reported. In this study, a comparison of the shear bond strength of various veneering materials, which are generally used in implant supported fixed dentures, on titanium were performed.
The purpose of this study was to compare the shear bond strength of various veneering materials: heat-polymerized acrylic resin, porcelain and indirect composite to grade II CP-Ti. The conventional method for making a metal-resin implant fixed complete denture with type VI gold alloy and heat-polymerized acrylic resin was also examined as a control.
A total of forty specimens were prepared. Ten milled CP-Ti specimens were prepared for each of three different veneering groups and ten cast gold specimens were used as controls. Thirty grade II CP-Ti (Dynamet, Santa Fe Springs, CA, USA) disc specimens of 9 mm diameter and 10 mm height were divided into three groups. In addition, each group was bonded to heat-polymerized acrylic resin (Lucitone 199, Dentsply Trubyte, York, PA, USA) (Group A, n=10), titanium porcelain (Triceram, Dentaurum, Ispringen, Germany) (Group B, n=10) and indirect composite (Sinfony, 3M ESPE, Seefeld, Germany) (Group C, n=10) respectively. The veneering materials were molded into the discs of 7 mm diameter and 2 mm height. Gold alloys (GOLDNIAN C-55, Shinhung, Seoul, Korea) were cast into the discs with retentive beads & loop of 9 mm diameter and 3 mm height and were bonded to heat-polymerized acrylic resin (Lucitone 199) (Control group, n=10). Framework materials used in this study are presented in Table 1, and diagram of specimen are shown in Fig. 1.
Group A specimens were prepared using grade II CP-Ti discs of 9 mm diameter and 10 mm height. They were wetground with silicon carbide (SiC) paper 500-600-1000-1200 grits gradually. After polishing the surface, retention grooves were made using a veradisk and sand blasting was done with 110 µm alumina particles. The air-abrasion was performed under the pressure of 60 psi for 10 seconds at a distance of 2 mm. They were then ultrasonically cleaned for 10 minutes and rinsed with ethyl acetate for 3 minutes. Group B specimens were prepared with grade II CP-Ti discs of 9 mm diameter and 10 mm height. They were polished with SiC paper. Then the 110 µm alumina air abrasive was applied to the test surface. They were ultrasonically cleaned and rinsed with ethyl acetate. In group C, grade II CP-Ti discs were wet-ground with SiC paper. After making retention grooves using a veradisk, ultrasonic cleansing and rinsing with ethyl acetate was performed. Rocatec (3M ESPE, Seefeld, Germany) 110 µm silica coating was applied from a 10 mm distance under the pressure of 280 kPa for 10 seconds following the manufacturer's instruction.
For the control group, auto-polymerizing acrylic resin patterns of 9 mm diameter and 3 mm height were fabricated for gold casting. After casting the gold specimens, they were embedded in an acrylic resin remaining uncovered test surface for bonding procedures. The specimens were airborne particle abraded with the same way mentioned above. The specimens were then ultrasonically cleaned and rinsed with ethyl acetate.
For group A, the Metal Primer II (Kuraray medical Inc., Okayama, Japan) was applied and Lucitone 199 was molded to the titanium surface. Then, the material was heat processed according to the manufacturer's instruction. For group B, a thin, uniform layer of bonder (Triceram bonder, Dentaurum, Ispringen, Germany) and opaque porcelain was applied with brush on the titanium surface. The body porcelain was applied using Teflon mold. The firing shrinkage was compensated with a second porcelain application. For group C, ESPE sil (3M ESPE, Seefeld, Germany), Opaquer (3M ESPE, Seefeld, Germany) were applied to the titanium surface, and light curing was done. Then Sinfony was bonded to the specimen.
For the control group, the Metal Primer II (Kuraray medical Inc. Okayama, Japan) was used for the chemical bonding material. The Lucitone 199 was applied to the gold specimens and heat processed. The surface treatment order for each group was presented in Table 2.
For shear bond strength measurement, all samples were thermocycled for 5000 cycles in 5-55℃ water. The maximum fracture force was measured using a Universal Testing Machine (Instron 4465, Canton, MA) with cross head speed of 1 mm/min, and then shear bond strength was calculated using the equation as follows:
After the shear bond test, the failure mode of veneering materials to titanium and gold alloys was examined using a stereomicroscope (Nikon SMZ-U Nikon Instruments Inc., Melville, NY). The interfaces of representative debonded specimens were analyzed using a scanning electron microscope (FE-SEM; HITACHI S-4700, Tokyo, Japan) at ×30 to ×10000 magnification.
Statistical analysis of the results was carried out with a Kruskal-Wallis Test (α=.05) and Mann-Whitney Test (α=.05) using SPSS (SPSS 15.0, SPSS Inc., Chicago, IL, USA).
The mean shear bond strength and standard deviations for the veneering materials to titanium groups were as follows: Ti-Lucitone 199 (12.11 ± 4.44 MPa); Ti-Triceram (11.09 ± 1.66 MPa); Ti-Sinfony (4.32 ± 0.64 MPa). One specimen was excluded in the Ti-Lucitone 199 group because of a wide variation of values. All of these experimental groups showed lower shear bond strength than the control group of gold casting with Lucitone 199 (16.14 ± 1.89 MPa), but there was no statistically significant difference between the Ti-Lucitone 199 group and the control group, and the Ti-Lucitone 199 group and the Ti-Triceram group. Mean and standard deviation of shear bond strength are shown in Fig. 2. Median, IQR and comparative significance of shear bond strength are shown in Table 3. Most of the failure patterns in all experimental groups were adhesive failure. SEM images of the debonded surfaces are shown in Fig. 3.
There are two methods of making the titanium frameworks; CAD/CAM milling and casting method. Titanium for the conventional casting method has many challenges. Casting of titanium alloy requires special equipment and procedures. In addition, during casting, oxides in the investment diffuse into titanium at high temperatures, forming the zone of contamination, known as the α-case.11 This surface layer results in inferior titanium-ceramic bonding.8,12,13,14 On the other hand, CP-Ti frameworks made by CAD/CAM milling has shown the accurate fit and adequate porcelain-titanium bonding in several studies.4,5,6,7 In this study, milled titanium disc was used for the experimental group to verify the usage as a substitute for gold casting. Since onepiece milled titanium framework fits accurately, the only concern was the bonding between milled titanium and veneering materials.
As a concern of the mechanical surface treatment of gold alloy, a variety of methods, such as retention beads, nail heads, peripheral undercuts, dovetails, wire loops and grooves, can be used to enhance the bond strength.15,16 In the unpublished pilot study of the author, mechanical treatment with beads showed lower shear bond strength than with wire loops. Higher or lower shear bond strength might be obtained with different mechanical methods in the control group. In groups A and C, CP-Ti specimens were grooved using a veradisk as a mechanical retention form. This is because retention forms, such as beads, hooks or nail heads, cannot be cut due to the limitations of CAD/CAM procedure. On the other hand, retention grooves were not made for group B because of the flow characteristics of the porcelain.
Chemical bonding between metal and veneering material is achieved using the silicoating technique.17 The silane on the metal surface bonds to the veneering materials chemically. But contamination of the silanated metal surface will remarkably reduce bond strength, and it is impossible for the dentist to confirm whether it has been accomplished satisfactorily. This is a clinical problem of the silicoating technique. Besides this, a comparison between chemical and mechanical treatments shows that mechanical retention is greater than chemical.18,19,20 However, chemical bonding has many advantages. It reduces microleakage at the interface and is useful in limited space where the mechanical retention methods are restricted. Also, chemical bonding is beneficial to the milled titanium framework which has limited mechanical retentions. In order to treat noble and base metal alloys simultaneously, the Metal Primer II (Kuraray medical Inc. Okayama, Japan) was used. It contained refined methacrylate with a thiophosphoric acid moiety as a functional monomer. This bonding system is effective for both titanium and gold alloy.21 The combination of chemical and mechanical retentive systems has been proposed to achieve greater retention. In the previous study, the association of chemical and mechanical retention showed higher bond strength than mechanical or chemical retention alone.22,23 Therefore, all the possible treatments for better retention were applied to this study.
Titanium reacts with oxygen at high temperature and makes an excessively thick oxide layer of TiO2. CP-Ti oxidized at 750℃ shows a thin oxide layer (approximately 32 nm) with good adherence to the titanium surface, whereas the oxide layer formed at 1000℃ shows a thick oxide layer (approximately 1 µm) with significantly lower adherence.24 Attempts to overcome titanium oxidation problems have been considered in the literature. An argon atmosphere improves porcelain-titanium alloy bonding by limiting the formation of an excessive and nonadherent oxide layer on the titanium surface during porcelain firing.25 Silicon nitrate (Si3N4) is coated on the titanium surface to prevent the oxidation of titanium and also to improve the bond strength between titanium and porcelain.9 Triceram used in this study was the low-fusing dental ceramic of the firing temperature under 750℃. Since a thin oxide layer with good adherence to the titanium surface was expected in this situation, an argon atmosphere or coated titanium surface was not needed in this study.
Another problem of porcelain-titanium bond strength is the stress caused by the difference of the thermal expansion coefficient between titanium and conventional noble metal porcelain. Low-fusing dental ceramics that match the coefficient of thermal expansion of titanium (8.4 × 10-6/℃) are appropriate.26,27 Triceram satisfies this requirement.
Rocatec was used to enhance the bonding of Sinfony to the titanium surface in this study. The silica-coated alumina (Al2O3) particles strike the titanium surface, creating microretention and cause embedding of a SiO2 layer on the surface. Then ESPE-Sil with functional methacrylic groups was applied. Therefore Rocatec is a hybrid bonding system that combines mechanical (microretention) and chemical (reactive groups and methacrylic groups) retention. However, the shear bond strength of Ti-Sinfony group shows the lowest value in this study. The low bond strength between indirect composite and metal is the main disadvantage of indirect veneering materials.28 Sinfony has more esthetic and color stabilization than heat curing denture base resin, but needs to improve its bonding properties.
Thermocycling is a common laboratory method to simulate the oral environment and decreases the bond strength of titanium and veneering materials. It affects relaxation of stresses that the veneering materials produced by polymerization shrinkage.29,30 Thermocycling results in a rapid increase in the rate of water absorption into the veneering materials at higher temperature.30,31 It makes a large mismatch of coefficient of thermal expansion between the veneering materials and the metal.30 Most current studies in the literature have different thermocycling times, but the common consensus is that thermocycling decreases the bond strength.30,32 The number of thermocycles proposed in the recent study was based on the assumption that at most 10 extreme thermocycles would occur per day.32,33 Therefore, the 5000 cycles would be equivalent to approximately 1.5 years in a clinical situation.
In this study, Group A showed comparatively the large standard deviation of shear bond strength. It may result from the unstandardized mechanical retention form or microvoid inside the veneering material. Although the gold alloy and ceramic combination has been successfully used for many decades, Persson and Bergman suggested that the bond strength of titanium-ceramic was higher than the bond strength of the gold alloy and ceramic combination.34 In the previous unpublished pilot study, the gold specimen with bead retention bond to Lucitone 199 showed lower shear bond strength (7.70 ± 1.46 MPa) than CP-Ti + Lucitone 199 (12.11 ± 4.44 MPa) or CP-Ti + Triceram (11.09 ± 1.66 MPa). This suggests that the mechanical retention form influences the shear bond strength between the gold alloy and veneering materials. It also suggests that CP-Ti + Lucitone 199 or CP-Ti + Triceram might be successfully used in a restricted space for mechanical retention of gold alloy. The three point flexural test has an advantage in providing complex tensions, being indicated for bond strength measuring of ceramo-metal specimens compared to the shear bond test stated by Pröbster et al.35 Several authors have proposed the use of the shear bond strength test.14,34,36 In this study, the shear bond test was performed for comparing bond strength of resin and porcelain. Further evaluation using bending tests such as the three or four point flexural test are needed for verification.
There are three layers of the specimens: (1) the metal layer, (2) the opaque layer, (3) the veneering material layer. A combined failure pattern involves at least two layers.16 In most of the experimental groups, the failure patterns were adhesive failures. This means that the bonding interface between metal and veneering material is the weakest point. In the control group, the major failure pattern was the combined pattern. However, the remaining veneering material is not because of the adhesive failure, but because of the mechanical retentive form.
Within the limits on this study, it can be suggested that the prosthesis utilizing the heat-polymerized acrylic resin or porcelain as veneering material to the CAD/CAM milled titanium frameworks is clinically acceptable. With more complementary methods, a larger sample size and long-term clinical observation is needed for further study.
The combination of casted gold alloy with Lucitone 199 showed the highest shear bond strength followed by titanium with Lucitone 199, titanium with Triceram. There were no significant differences between the Ti-Lucitone 199 group and the control group, the Ti-Lucitone 199 group and the Ti-Triceram group. The combination of titanium with Sinfony showed the lowest shear bond strength. In conclusion, casted gold alloy with Lucitone 199, titanium with Lucitone 199, and titanium with Triceram have shown the clinically acceptable shear bond strength.
Figures and Tables
Fig. 3
SEM images of the debonded surfaces: (A)-(C) CP-Ti/Lucitone 199, (D)-(F) CP-Ti/Triceram, (G)-(I) CP-Ti/Sinfony, (J)-(L) Gold alloy/Lucitone 199. At higher magnification, the small amount of veneering materials retained in the titanium surfaces suggests mainly adhesive bond failures (arrows) ((A)-(I)). Veneering materials under retentive form is observed in control group (arrow) ((J)-(L)).
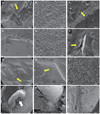
Table 1
Titanium and gold alloy

Table 2
Surface treatment of each group

References
1. Lautenschlager EP, Monaghan P. Titanium and titanium alloys as dental materials. Int Dent J. 1993; 43:245–253.
2. King AW, Lautenschlager E, Chai J, Gilbert J. A comparison of the hardness of different types of titanium and conventional metal ceramics. J Prosthet Dent. 1994; 72:314–319.
3. Wang RR, Fenton A. Titanium for prosthodontic applications: a review of the literature. Quintessence Int. 1996; 27:401–408.
4. Jemt T, Bäck T, Petersson A. Precision of CNC-milled titanium frameworks for implant treatment in the edentulous jaw. Int J Prosthodont. 1999; 12:209–215.
5. Riedy SJ, Lang BR, Lang BE. Fit of implant frameworks fabricated by different techniques. J Prosthet Dent. 1997; 78:596–604.
6. Ortorp A, Jemt T, Bäck T, Jälevik T. Comparisons of precision of fit between cast and CNC-milled titanium implant frameworks for the edentulous mandible. Int J Prosthodont. 2003; 16:194–200.
7. Gilbert JL, Covey DA, Lautenschlager EP. Bond characteristics of porcelain fused to milled titanium. Dent Mater. 1994; 10:134–140.
8. Kimura H, Horng CJ, Okazaki M, Takahashi J. Oxidation effects on porcelain-titanium interface reactions and bond strength. Dent Mater J. 1990; 9:91–99.
9. Wang RR, Welsch GE, Monteiro O. Silicon nitride coating on titanium to enable titanium-ceramic bonding. J Biomed Mater Res. 1999; 46:262–270.
10. Ohkubo C, Watanabe I, Hosoi T, Okabe T. Shear bond strengths of polymethyl methacrylate to cast titanium and cobalt-chromium frameworks using five metal primers. J Prosthet Dent. 2000; 83:50–57.
11. Miyakawa O, Watanabe K, Okawa S, Nakano S, Kobayashi M, Shiokawa N. Layered structure of cast titanium surface. Dent Mater J. 1989; 8:175–185.
12. Kaus T, Pröbster L, Weber H. Clinical follow-up study of ceramic veneered titanium restorations-three-year results. Int J Prosthodont. 1996; 9:9–15.
13. Cai Z, Bunce N, Nunn ME, Okabe T. Porcelain adherence to dental cast CP titanium: effects of surface modifications. Biomaterials. 2001; 22:979–986.
14. Taira Y, Matsumura H, Yoshida K, Tanaka T, Atsuta M. Influence of surface oxidation of titanium on adhesion. J Dent. 1998; 26:69–73.
15. Lee CF, Pierpont HP, Strickler ER. The effect of bead attachment systems on casting patterns and resultant tensile bond strength of composite resin veneer cast restorations. J Prosthet Dent. 1991; 66:623–630.
16. Shue SL, Nicholls JI, Townsend JD. The effect of metal retentive designs on resin veneer retention. J Prosthet Dent. 1987; 58:297–305.
17. McConnell RJ. Metal-resin bonding. J Calif Dent Assoc. 1993; 21:38–42.
18. Jones RM, Moore BK, Goodacre CJ, Munoz-Viveros CA. Microleakage and shear bond strength of resin and porcelain veneers bonded to cast alloys. J Prosthet Dent. 1991; 65:221–228.
19. Barzilay I, Myers ML, Cooper LB, Graser GN. Mechanical and chemical retention of laboratory cured composite to metal surfaces. J Prosthet Dent. 1988; 59:131–137.
20. Faria AC, de Matos RL, Rodrigues RC, Antunes RP, Ribeiro RF, de Mattos Mda G. Comparative study of chemical and mechanical retentive systems for bonding of indirect composite resin to commercially pure titanium. Braz Dent J. 2008; 19:134–138.
21. Taira Y, Yanagida H, Matsumura H, Yoshida K, Atsuta M, Suzuki S. Adhesive bonding of titanium with a thione-phosphate dual functional primer and self-curing luting agents. Eur J Oral Sci. 2000; 108:456–460.
22. Vojvodic D, Predanic-Gasparac H, Brkic H, Celebic A. The bond strength of polymers and metal surfaces using the 'silicoater' technique. J Oral Rehabil. 1995; 22:493–499.
23. Andrade Tarozzo LS, Chiarello De Mattos Mda G, Faria Ribeiro R, Semprini M. Comparison of retentive systems for composites used as alternatives to porcelain in fixed partial dentures. J Prosthet Dent. 2003; 89:572–578.
24. Adachi M, Mackert JR Jr, Parry EE, Fairhurst CW. Oxide adherence and porcelain bonding to titanium and Ti-6Al-4V alloy. J Dent Res. 1990; 69:1230–1235.
25. Atsü S, Berksun S. Bond strength of three porcelains to two forms of titanium using two firing atmospheres. J Prosthet Dent. 2000; 84:567–574.
26. Low D, Sumii T, Swain M. Thermal expansion coefficient of titanium casting. J Oral Rehabil. 2001; 28:239–242.
27. Togaya T, Suzuki M, Tsutsumi S, Ida K. An application of pure titanium to the metal porcelain system. Dent Mater J. 1983; 2:210–219.
28. Petridis H, Garefis P, Hirayama H, Kafantaris NM, Koidis PT. Bonding indirect resin composites to metal: Part 1. Comparison of shear bond strengths between different metal-resin bonding systems and a metal-ceramic system. Int J Prosthodont. 2003; 16:635–639.
29. Tanaka T, Kamada K, Matsumura H, Atsuta M. A comparison of water temperatures for thermocycling of metal-bonded resin specimens. J Prosthet Dent. 1995; 74:345–349.
30. Kim JY, Pfeiffer P, Niedermeier W. Effect of laboratory procedures and thermocycling on the shear bond strength of resin-metal bonding systems. J Prosthet Dent. 2003; 90:184–189.
31. Kourtis SG. Bond strengths of resin-to-metal bonding systems. J Prosthet Dent. 1997; 78:136–145.
32. Gale MS, Darvell BW. Thermal cycling procedures for laboratory testing of dental restorations. J Dent. 1999; 27:89–99.
33. Rossomando KJ, Wendt SL Jr. Thermocycling and dwell times in microleakage evaluation for bonded restorations. Dent Mater. 1995; 11:47–51.
34. Persson M, Bergman M. Metal-ceramic bond strength. Acta Odontol Scand. 1996; 54:160–165.
35. Pröbster L, Maiwald U, Weber H. Three-point bending strength of ceramics fused to cast titanium. Eur J Oral Sci. 1996; 104:313–319.
36. Stannard JG, Marks L, Kanchanatawewat K. Effect of multiple firing on the bond strength of selected matched porcelain-fused-to-metal combinations. J Prosthet Dent. 1990; 63:627–629.