Abstract
PURPOSE
The purpose of this study was to evaluate the effects of the surface treatment and shape of the dental alloy on the composition of the prosthetic work and its metallic ion release in a corrosive medium after casting.
MATERIALS AND METHODS
Orion Argos (Pd-Ag) and Orion Vesta (Pd-Cu) were used to cast two crowns and two disks. One of each was polished while the other was not. Two as-received alloys were also studied making a total of 5 specimens per alloy type. The specimens were submersed for 7 days in a lactic acid/sodium chloride solution (ISO standard 10271) and evaluated for surface structure characterization using SEM/EDAX. The solutions were quantitatively analysed for the presence of metal ions using ICP-MS and the results were statistically analysed with one-way ANOVA and a Tukey post-hoc test.
RESULTS
Palladium is released from all specimens studied (range 0.06-7.08 µg·cm-2·week-1), with the Pd-Cu alloy releasing the highest amounts. For both types of alloys, ion release of both disk and crown pairs were statistically different from the as-received alloy except for the Pd-Ag polished crown (P>.05). For both alloy type, disk-shaped pairs and unpolished specimens released the highest amounts of Pd ions (range 0.34-7.08 µg·cm-2·week-1). Interestingly, in solutions submerged with cast alloys trace amounts of unexpected elements were measured.
CONCLUSION
Shape and surface treatment influence ion release from dental alloys; polishing is a determinant factor. The release rate of cast and polished Pd alloys is between 0.06-0.69 µg·cm-2·week-1, which is close to or exceeding the EU Nickel Directive 94/27/EC compensated for the molecular mass of Pd (0.4 µg·cm-2·week-1). The composition of the alloy does not represent the element release, therefore we recommend manufacturers to report element release after ISO standard corrosion tests beside the original composition.
The general population is exposed to palladium (Pd) due to inhalation and contact with jewelry and especially dental restorations.1,2 Although the use of palladium in dentistry had been described already in 1933,3 its wide-scale use started in the early 70's due to the increasing gold price demanding the use of other metals as lower-cost alternatives.4 Since then, the palladium content in dental alloys has exponentially increased. For dental applications, palladium is alloyed with other metals such as gold (Au), silver (Ag), copper (Cu), gallium (Ga) and zinc (Zn).5 Among other alloys, palladium-silver based (Pd-Ag) and palladium-copper based (Pd-Cu) alloys became attractive for restorative dentistry.
Dental restorations are in situ for many years and are exposed to many factors such as mechanical load and the conditions of the oral environment that lead to substantial metal ion release with possible local and systemic adverse reactions as a result.2,5,6 Health concerns about palladium are mainly due to its effect on the immune system. Palladium is ranked, after nickel (Ni), as the second most frequent reacting skin sensitizer within metals in epidemiological studies with a prevalence of 7.4% in dental patients.1 Furthermore, Ni allergic individuals are more susceptible to Pd, and the majority of the people with Pd allergy are also sensitive to Ni due to cross reactivity.2,7 Clinically, signs of allergic contact dermatitis and allergic contact granuloma, contact stomatitis with gingival hyperplasia and oral lichen planus are described in case reports on Pd sensitization after exposure to Pd from dental restorations. Also, general symptoms such as swelling of the lips and cheeks, burning mouth, dizziness, asthma, chronic urticaria have been described. The removal of these restorations resulted in remission of the symptoms. Besides, occupational exposure to Pd may occur in dental technicians, miners and workers of the electronics and chemical industries.1,2,7
It has been shown that alloys containing palladium may release up to 33.7 µg·cm-2·week-1 metallic ions in a solution of sodium chloride and lactic acid at a pH of 2.3.8 Of note, these in vitro experiments used alloys that were industrially cast, standardized shaped, and highly polished and therefore do not resemble the in vivo situation. Many factors influence corrosion of the dental alloys such as the composition9,10 and the microstructure of the alloy,5,11,12 but also the surrounding environment6,13,14 and the manufacturing process.15
During the casting process impurities might be included in the alloys, resulting in a product with different composition from what manufacturer claimed for an "as-received" alloy. This may result either from the material transformation during the casting procedure or from the inclusion of residual metals from previous work. Furthermore, some laboratories recast the sprues by adding new alloy pellets. This requires caution since it was shown that recasting can reduce the corrosion resistance of Pd-based alloys.15 All together, these factors might influence the corrosion of the final product, leading to different corrosion properties from what manufacturer claimed. Nevertheless, the studies on the electrochemical properties of these alloys after casting reported satisfactory corrosion behavior.11,15 Also, when testing high-palladium alloys, a spontaneous passive behavior in electrochemical conditions similar to those in the oral environment was shown16 and a Ag-based Pd alloy could be recast up to 4 times with little effect on its corrosion susceptibility in artificial saliva.17
The aim of the present study was to evaluate whether the surface treatment and shape of the dental alloy would influence the elemental release of cast standardized shapes and crowns, compared to the "as received" Pd-Ag and Pd-Cu alloys.
The corrosion behavior of two crowns, two disks and the "as-received" alloys of a Pd-Cu (Orion Vesta, Elephant Dental B.V., Hoorn, Netherlands) and a Pd-Ag (Orion Argos, Elephant Dental B.V., Hoorn, Netherlands) alloy was evaluated. The disks (d = 10.0 mm; h = 1.4 ± 0.1 mm thick) and the crowns (polycarbonate incisor shaped temporary crowns (P-101), 3M™ ESPE™, St. Paul, MN, USA), were cast according to manufacturer's instructions using the lost-wax technique, phosphate-bonded graphitefree casting investment and individual ceramic crucibles per alloy; melting was done by means of a gas-oxygen torch. The two crowns and two disks were cast at the same time on one sprue/base to ensure that the variation due to the casting procedure is minimized. The final surface areas of the specimens were: 2.06 cm2 for the disks, 2.57 cm2 for the crowns and 1.17 cm2 for the "as-received" alloys. One crown and one disk from each alloy type were wet-ground with 600-grit and 1200-grit grinding paper. The other pair and the "as-received" alloy from each alloy type remained unpolished. To submerse the specimens in the test medium, a suspension point of composite (Filtek™ Supreme XT, 3M ESPE) with a nylon wire was created (see Fig. 1). The specimens were ultrasonically cleaned with alcohol 99.9% (Emsure™, Merck, Germany) for 5 minutes. The plastic vials were rinsed with distilled water and filled with 1% HNO3 (Merck Suprapur) for 24 hours after which, they were rinsed again with distilled water. The specimens were submersed for 7 days in 5 mL at 37℃. The solution used consisted of a lactic acid/sodium chloride solution from the ISO 10271 standard (Dental metallic materials - Corrosion test methods)18: pH = 2.3 ± 0.1, [NaCl] = 8.845 g (Merck Suprapur), [Lactic acid 90%] = 9.2 g/L (Merck Extrapur). The solutions with the submersed specimen were gently shaken daily for 30 seconds.
The samples were diluted 10x with 1% HNO3 (Merck Suprapur) and analysed by Inductively Coupled Plasma Mass Spectroscopy (ICP-MS)(ELAN 6100, SCIEX, Toronto, Canada). The palladium concentration was measured with a quantitative analysis method (no. of replicates = 8) using a blank and standard solutions of palladium of 10.0 ppb and 100.0 ppb (VWR International Ltd., Lutterworth, Leicestershire, UK). The detection limit, defined as 3x the standard deviation of the samples, was 0.7 ppb or 0.035 µg·cm-2·week-1 for palladium for the current test procedure. The concentrations of Au, Ag, Cu, Sn, In and Ga were measured using semi-quantitative analysis method (TotalQuant; no. of replicates = 1). TotalQuant, being a semi-quantitative program, gives quantitative results typically within +/-25% of the real value in simple matrices. The following settings were used for all analysis: RF power 1100W; nebulizer gas flow rate 0.92 L/min; fluid peri pump rate 24 rpm. Control samples were also analysed and the values for each ion were subtracted from each analysed sample. These controls were the experimental media (i.e. lactic acid/sodium chloride solution) without contact with the specimens.
Before and after submersion in the lactic acid/sodium chloride solution the specimens were also examined with Scanning Electron Microscopy and Energy Dispersive X-ray spectrometry (SEM/EDAX; XL20, Philips/FEI, Eindhoven, The Netherlands) for surface structure characterization using the secondary electrons detector and the mapping mode of the EDAX. Furthermore, the composition of the alloys (Table 1) was analysed by EDAX with a detection limit of 0.2 wt%.
The results of the quantitative analysis method of the Pd ion release (n=8) were statistically analysed using oneway ANOVA and Tukey post-hoc tests at a P-level of .05. The software used was SigmaStat 3.1 (SYSTAT Software, Inc., Point Richmond, CA, USA). It should be noted that all data, experimental and literature, are converted to µg·cm-2·week-1, using the given experiment conditions (volume of the medium, specimen surface area, observation period).
The metal ion release from the 5 specimens of the two alloys studied is summarized in Table 2. One-way ANOVA (P<.001) showed that all measured concentrations were significantly different from each other with two exceptions: (i) the "as received" Pd-Cu alloy (Orion Vesta) and the "as received" Pd-Ag alloy (Orion Argos) (P>.05), and (ii) the "as received" Pd-Ag alloy (Orion Argos) and the polished crown of the Pd-Ag alloy (Orion Argos) (P>.05).
Casting, surface treatment, and shape of the specimen had a significant effect on the palladium release from both alloys. Casting the Pd-Cu alloy (Orion Vesta) followed by polishing never resulted in the Pd release of the "as received" alloy (0.69/0.25 vs. 0.11 µg·cm-2·week-1). Casting the Pd-Ag alloy (Orion Argos) followed by polishing resulted in the same Pd release of the "as received" alloy but only for the crown specimen (0.34/0.06 vs. 0.07 µg·cm-2·week-1). The unpolished disks released higher amounts of palladium for both types of alloy, where Pd-Cu alloy (Orion Vesta) released the highest amounts. Interestingly, the shape also had an effect. For both alloys, the disks showed much higher palladium release compared to the crowns. This was observed for both the polished and unpolished specimens.
Fig. 2 shows the SEM and EDAX mapping images (Pd and Ag) of the surface of the polished specimens of Pd-Ag alloys (Orion Argos), before and after immersion in the lactic acid/sodium chloride solution and Fig. 3 the SEM and EDAX mapping images (Pd and Ag) of the surface of the unpolished specimens. The EDAX maps of Pd and Ag showed that the microstructure of the Pd-Ag alloy was homogenous. Unpolished specimens presented a coarser surface than their polished pairs. After immersion in the lactic acid/sodium chloride solution medium no or little differences in the surface appearance could be seen. The SEM/EDAX images of Orion Vesta were similar (data not shown).
The typical components of palladium-based alloys are silver (Ag), palladium (Pd), gallium (Ga) and copper (Cu).5 Besides, our specimens also contained gold (Au), tin (Sn), indium (In), and trace amounts of zinc (Zn), ruthenium (Ru) and iridium (Ir). These are thought to improve the mechanical properties of the dental alloys.19 The release of trace amounts of Cu from the Pd-Ag alloy (Orion Argos) and of Sn from the Pd-Cu alloy (Orion Vesta) was detected by ICP-MS analysis of the immersion solutions. Since the casting process was done as contamination-free as possible (e.g. use of a phosphate-bonded graphite-free casting investment and individual ceramic crucibles per alloy), the presence of this elements on the solution is likely due to the presence of trace amounts of these elements in the original alloy, under the detection limit of the EDAX (<0.5 wt%). Despite the presence of gold (Au) in both types of alloys, its release was not detected from any of the specimens.
For all specimens, Pd-Cu alloy (Orion Vesta) released higher amounts of Pd than Pd-Ag alloy (Orion Argos) did. Even though the wt% of Pd was higher than that of other metals, the release of silver (Ag) from the Pd-Ag alloy (Orion Argos) and copper (Cu) from the Pd-Cu alloy (Orion Vesta) was higher than the release of Pd itself from each type of alloy. This is in line with the theory that the less noble metal within the alloy is released more easily according to the liabilities of each metal. It is also consistent with earlier findings that the Pd release is not proportional to the content of Pd in the composition of the dental alloy.10,20 An explanation for the corrosion behavior of Pd-Cu and Pd-Ag alloys was proposed by Sarkar et al.21 in which intraoral corrosion of high-Pd alloys was associated with dealloying of base metals. Further, their proposition on the protective nature of Ag in limiting Pd release might also have been observed in our findings. The Pd-Ag alloy released less Pd compared to the Pd-Cu alloy, even after accounting for the higher Pd content in the composition of the Pd-Cu alloy (i.e. the release of Pd from the Pd-Cu alloy was always at least double than that of Pd-Ag even though its Pd content was not). Besides the main components of the alloys studied (Pd, Ag, and Cu), Sn, In and Ga also showed a variable amount of corrosion products. The influence of those products in the cytotoxicity and biocompatibility of these alloys deserves further studies.
Surprisingly, the shape of the specimens considerably influenced the metal ion release. Comparison of the unpolished disk and the unpolished crown showed that the corrosion of the disk was 3-4 times higher. The same observation was made for the polished specimens and also in both alloys. To our knowledge, there are no previous reports on the influence of shape on the corrosion of Pd-based dental alloys and an explanation is not straightforward. Besides shape, the difference between the crown and the disk is the layer thickness: the crown is generally slightly thinner, with approximately 1 mm, which may account for the different release. Furthermore, the corrosion resistance of these alloys can also be influenced by manipulation of the alloys, i.e. the casting procedure and finishing and porcelain-firing heat treatment.15,22,23 During the casting procedure, thin margins are affected differently by heat/cooling procedures, which can result in different microstructure and subsequently influence properties such as corrosion resistance.24,25,26
Analysis of the surface structure under the SEM showed a contrast between the homogeneous surface of the polished specimens and the rough, coarse appearance of the unpolished ones. Independently of the shape, polished specimens released much lower amounts of Pd than their unpolished pairs for both types of alloys, which is in line with previously reported results.22 Although the rough surface of the unpolished specimens has a bigger surface area, this will not account for the 4 to 64 fold increase in the corrosion rate. The most important factor in the increase in corrosion might therefore be the crevice corrosion in the coarse surface instead of the enhancement surface area due to the roughness. The polishing process can also create a homogeneous surface which may enable the formation of the protective layer, minimizing corrosion to some extent.
It was reported that high noble cast alloys containing gold (Au) have the highest corrosion resistance, followed by Au-based and Pd-based noble alloys.27 Au-Pd alloys were considered to have the highest corrosion resistance compared to other Au, Ag, Pd and Ni-based alloys.13 In our study, the lower Pd release from Pd-Ag alloy (Orion Argos) is in accordance with this. Furthermore, Au-Pd, Pd-Ag and Au-Pt-Pd alloys were reported to release minimal amounts of Pd (<10 µg·cm-2·week).8 In our study, only "as-received" alloys (and the polished crown) released low amounts of Pd, while the disk and crown specimens released much higher amounts of Pd. This suggests that the casting process and manipulation to shape the specimen introduce modifications in the alloy that play an important role in the corrosion resistance of the resultant product.
For the clinical implication it is interesting to compare the Pd release to the release of nickel (Ni), especially because it was shown that these molecules have a cross reactivity for hypersensitivity.28,29,30 The release rate for Ni assemblies that are inserted into pierced ears and other pierced parts of the human body should be less than 0.2 µg·cm-2·week-1 (EU Nickel Directive 94/27/EC). For Pd we may estimate that its release rate should be less than 0.4 µg·cm-2·week-1 considering its molecular mass. While the "as received" alloys are well below this limit, cast Pd-Ag is slightly below the limit and cast Pd-Cu alloys exceeds it. It should be noted that these values are obtained in a lactic acid solution as determined by the ISO standard for corrosion testing. However, these circumstances are also possible in the oral environment. Therefore, since the element release is fundamental for adverse reactions, we recommend manufacturers to include the element release from the dental alloys after ISO standard corrosive tests in their material's safety data sheet.
Shape and surface treatment influence the metallic ion release from Pd-based dental alloys with polishing being a determinant factor. The release rate of Pd from cast and polished Pd alloys is between 0.06 - 0.69 µg·cm-2·week-1; Pd-Cu alloy released more Pd than Pd-Ag alloys. These values are close to or exceed the EU Nickel Directive 94/27/EC compensated for the molecular mass of Pd (0.4 µg·cm-2 ·week-1) Finally, since the element release is fundamental for adverse reactions and the composition of the alloy does not determine the element release, we recommend manufacturers to include the element release from the dental alloys after ISO standard corrosive tests beside the original composition of the as-received alloy in their material's safety data sheet.
Figures and Tables
Fig. 2
SEM (SE) and EDAX mapping images (Pd and Ag) of the polished Pd-Ag alloy (Orion Argos) disk before (A) and after immersion for 7 days in 5 mL at 37℃ in a lactic acid solution specified by the ISO 10271 standard (B).
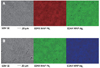
Fig. 3
SEM (SE) and EDAX mapping images (Pd and Ag) of the unpolished Pd-Ag alloy (Orion Argos) disk before (A) and after immersion for 7 days in 5 mL at 37℃ in a lactic acid solution specified by the ISO 10271 standard (B).
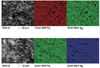
Table 1
Composition (in wt%) of Pd-Ag (Orion Argos) and Pd-Cu (Orion Vesta) alloys according to the manufacturer, EDAX analysis, and their wt% of corrosion products after one week exposure to the lactic acid solution (ISO 10271 standard)
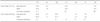
*Contain also traces of Zn, Ru, Ir (<0.2wt%). †wt% corrosion products derived from Table 2.
Table 2
Ion release from Pd-Cu (Orion Vesta) and Pd-Ag (Orion Argos) alloys (µg·cm-2·week-1) in lactic acid solution from the ISO 10271 standard. Standard deviations are given in between brackets
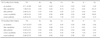
ACKNOWLEDGEMENTS
The authors would like to acknowledge Narek Grigorjan and André Rijswijk who partly performed the experiment and Arie Werner for the SEM-EDAX analysis.
References
1. Faurschou A, Menné T, Johansen JD, Thyssen JP. Metal allergen of the 21st century-a review on exposure, epidemiology and clinical manifestations of palladium allergy. Contact Dermatitis. 2011; 64:185–195.
2. Kielhorn J, Melber C, Keller D, Mangelsdorf I. Palladium-a review of exposure and effects to human health. Int J Hyg Environ Health. 2002; 205:417–432.
3. Wise EM, Eash JT. The Role of the Platinum Metals in Dental Alloys. III. The Influence of Platinum and Palladium and Heat Treatment upon the Microstructure and Constitution of Basic Alloys. Trans AIME Inst Metals Div. 1933; 102:270–307.
4. Goodacre CJ. Palladium-silver alloys: a review of the literature. J Prosthet Dent. 1989; 62:34–37.
5. Wataha JC. Biocompatibility of dental casting alloys: a review. J Prosthet Dent. 2000; 83:223–234.
6. Schmalz G, Garhammer P. Biological interactions of dental cast alloys with oral tissues. Dent Mater. 2002; 18:396–406.
7. WHO. International Programme on Chemical Safety (IPCS) Environmental Health Criteria (EHC) 226. Organization WH. Palladium. Geneva: 2002. p. 202.
8. Manaranche C, Hornberger H. A proposal for the classification of dental alloys according to their resistance to corrosion. Dent Mater. 2007; 23:1428–1437.
9. Endo K, Ohno H, Matsuda K, Asakura S. Electrochemical and surface studies on the passivity of a dental Pd-based casting alloy in alkaline sulphide solution. Corrosion Science. 2003; 45:1491–1504.
10. Wataha JC, Lockwood PE. Release of elements from dental casting alloys into cell-culture medium over 10 months. Dent Mater. 1998; 14:158–163.
11. Mülders C, Darwish M, Holze R. The influence of alloy composition and casting procedure upon the corrosion behaviour of dental alloys: an in vitro study. J Oral Rehabil. 1996; 23:825–831.
12. Wataha JC, Craig RG, Hanks CT. The release of elements of dental casting alloys into cell-culture medium. J Dent Res. 1991; 70:1014–1018.
13. Wataha JC, Lockwood PE, Khajotia SS, Turner R. Effect of pH on element release from dental casting alloys. J Prosthet Dent. 1998; 80:691–698.
14. Wataha JC, Nelson SK, Lockwood PE. Elemental release from dental casting alloys into biological media with and without protein. Dent Mater. 2001; 17:409–414.
15. Viennot S, Lissac M, Malquarti G, Dalard F, Grosgogeat B. Influence of casting procedures on the corrosion resistance of clinical dental alloys containing palladium. Acta Biomater. 2006; 2:321–330.
16. Cai Z, Vermilyea SG, Brantley WA. In vitro corrosion resistance of high-palladium dental casting alloys. Dent Mater. 1999; 15:202–210.
17. Horasawa N, Marek M. The effect of recasting on corrosion of a silver-palladium alloy. Dent Mater. 2004; 20:352–357.
18. ISO 10271:2011. Dentistry-Corrosion test methods for metallic materials. Geneva; Switzerland: International Standards Organization (ISO);2011.
19. Nitta SV, Clark WA, Brantley WA, Grylls RJ, Cai Z. TEM analysis of tweed structure in high-palladium dental alloys. J Mater Sci Mater Med. 1999; 10:513–517.
20. Wataha JC, Malcolm CT. Effect of alloy surface composition on release of elements from dental casting alloys. J Oral Rehabil. 1996; 23:583–589.
21. Sarkar NK, Berzins DW, Prasad A. Dealloying and electroformation in high-Pd dental alloys. Dent Mater. 2000; 16:374–379.
22. Kaneko T, Hattori M, Hasegawa K, Yoshinari M, Kawada E, Oda Y. Influence of finishing on the electrochemical properties of dental alloys. Bull Tokyo Dent Coll. 2000; 41:49–57.
23. Berzins DW, Kawashima I, Graves R, Sarkar NK. Heat treatment effects on electrochemical corrosion parameters of high-Pd alloys. J Mater Sci Mater Med. 2008; 19:335–341.
24. Carr AB, Brantley WA. New high-palladium casting alloys: 1. Overview and initial studies. Int J Prosthodont. 1991; 4:265–275.
25. Carr AB, Cai Z, Brantley WA, Mitchell JC. New high-palladium casting alloys: Part 2. Effects of heat treatment and burnout temperature. Int J Prosthodont. 1993; 6:233–241.
26. Brantley WA, Cai Z, Carr AB, Mitchell JC. Metallurgical Structures of as-Cast and Heat-Treated High-Palladium Dental Alloys. Cells Mater. 1993; 3:103–114.
27. Tuna SH, Pekmez NO, Keyf F, Canli F. The influence of the pure metal components of four different casting alloys on the electrochemical properties of the alloys. Dent Mater. 2009; 25:1096–1103.
28. Pistoor FH, Kapsenberg ML, Bos JD, Meinardi MM, von Blomberg ME, Scheper RJ. Cross-reactivity of human nickelreactive T-lymphocyte clones with copper and palladium. J Invest Dermatol. 1995; 105:92–95.
29. Moulon C, Weltzien HU. Human Nickel-Specific T-Cell Clones Cross-React with Other Metal Sensitizers - Implications for Associated Contact Sensitivities. J Invest Dermatol. 1995; 105:451.
30. Hindsén M, Spirén A, Bruze M. Cross-reactivity between nickel and palladium demonstrated by systemic administration of nickel. Contact Dermatitis. 2005; 53:2–8.