Abstract
PURPOSE
Bone density at implant placement site is a key factor to obtain the primary stability of the fixture, which, in turn, is a prognostic factor for osseointegration and long-term success of an implant supported rehabilitation. Recently, an implant motor with a bone density measurement probe has been introduced. The aim of the present study was to test the objectiveness of the bone densities registered by the implant motor regardless of the operator performing them.
MATERIALS AND METHODS
A total of 3704 bone density measurements, performed by means of the implant motor, were registered by 39 operators at different implant sites during routine activity. Bone density measurements were grouped according to their distribution across the jaws. Specifically, four different areas were distinguished: a pre-antral (between teeth from first right maxillary premolar to first left maxillary premolar) and a sub-antral (more distally) zone in the maxilla, and an interforaminal (between and including teeth from first left mandibular premolar to first right mandibular premolar) and a retroforaminal (more distally) zone in the lower one. A statistical comparison was performed to check the inter-operators variability of the collected data.
Implant primary stability at implant placement plays a pivotal role in determining the osseointegration of the fixture and therefore its long-term success.1 Primary stability is affected by different factors: bone quantity, implant geometry and surgical technique.2 A correct assessment of the anatomical conditions at the placement sites guides the choice of the best implant site preparation approach and of the most appropriate loading protocol among immediate, early or delayed.3 Computed tomography (CT) or cone beam computed tomography (CBTC) scans provide a detailed evaluation of the implant placement site anatomy, and therefore a precise assessment of the bone quantity and quality available at the site itself. Nevertheless, both techniques seem to be still not sufficiently reliable in measuring bone density. CT scans seem to provide a reliable quantitative pre-surgical measurement of the bone density around the implant sites,4 but concerns have been expressed about the reproducibility of the technique.5,6 Attempts of getting reliable numerical bone density measurements from cone-beam CT scans have been made,7 but it has been found that results, are affected by the CT unit and even by the Field of View (FOV) of the unit itself.8,9 The only reliable numerical measurement of bone density at implant sites is the histomorphometric assessment, although it is unfeasible as it requires a bone biopsy and therefore it cannot be used intra-operatively.
For the reasons listed above, measurements of bone density at implant sites still relies on subjective assessments, as described by the most common classifications, namely the ones by Lekholm and Zarb,10 or by Misch11 with further changes introduced by Trisi and Rao.12 However, such classifications suffer from being empirical and subjective, and moreover, they have a limited utility in the diagnosis as they are performed at the time of implant placement. Recently, an intra-operatory bone density measuring system has been introduced; it is a bone measuring density probe provided with an implant motor unit.13 The unit is endowed with an instantaneous torque measuring system. Such a torque is a function of the friction exerted by the tunnel bone walls; indeed, the average torque along the whole tunnel is significantly correlated with the bone density of the surrounding bone wall, as it has been shown by a recent study on bovine ribs.13 When used in a clinical context, 1254 bone density measurements in 464 patients showed the device allowed the distinction of anatomic areas with different bone densities, namely IDI1-4 (Intra-operatory Density Index) from the more to the less dense.14 If the repeatability of measurements with such device were confirmed, it would represent an invaluable tool to perform a proper intra-operatory diagnosis of bone density and therefore, to adapt the implant site preparation accordingly. However, the measurements could be operator-dependent, thus providing useful but still subjective assessment. The aim of the present study was to test the inter-operator repeatability in order to validate the objectiveness of the bone density values recorded by this system.
Thirty-nine operators were provided with a bone density measurement unit, namely a TMM2 implant motor (IDI Evolution, Concorezzo, Italy) and they, independently, collected 3704 bone density measurements of implant placement sites. When a bone density measurement was performed a measuring probe was mounted on the handpiece. The probe was a 2 mm wide cylinder featuring equally spaced threads, whose width was 3 mm and shape was a 1-degree reverse cone (patented, Fig. 1). At tunnel preparation, the surgeons first created a 2.2 mm round hole for the whole depth of the cortical bone layer, and subsequently used a 2.3 mm bur to drill a first, narrow tunnel, till the desired implant placement depth. Before enlarging the tunnel to its final width, in order to standardize the bone density measurement procedure and permit further statistical comparison among homogeneous data, operators were instructed to use a bone reamer to drill a 3 mm deep, 3 mm wide, circular access hole, thus eliminating the first overdense cortical bone ridge layer. After mounting the probe on the handpiece, and switching it in its measurement mode, the first probe thread was inserted in the access hole. The surgeons proceeded to switch on rotation and let the probe screw itself into the previously prepared tunnel, without exerting any additional pressure (Fig. 2). The upside-down cone shape of the threads allowed the device to measure the friction encountered by the first thread only. When the device was in its measurement mode the probe rotated at a given speed (30 rpm) and could reach 35 Ncm maximum torque. While the probe deepened into the tunnel, digital software performed a high frequency sample measurement of the instantaneous torque needed to keep the speed constant. The device recorded also the depth the probe had reached, given the fact probe threads were evenly spaced, and their pitch was known. The device, calibrated with high-precision dynamometers, automatically performed a self-calibration routine at each switching on. The device displayed a torque/depth graph as an output showing how the instantaneous torque had varied according to the probe depth (Fig. 3). During measurement average torque and curve integral were calculated and displayed together with the peak torque on the device display.
Data were recorded in the device solid state memory to be downloaded to a common PC for statistical analysis.
Mean torque measurements collected by each operator were compared applying the Kruskall-Wallis test at a confidence level of P=.05. Statistical analysis was performed with standard analysis software (Origin 9.0, Microcal, Northampton, MA,USA).
Further analysis was performed as follows: four anatomic zones were defined, namely a pre-antral (the upper anterior teeth from first right maxillary premolar to first left maxillary premolar) and a sub-antral (more distal) zone in the upper maxilla, and an interforaminal (the lower anterior teeth from first left mandibular premolar to first right mandibular premolar) and a retroforaminal (more distal) zone in the lower one.
Data from each operator were pooled according to the position in the four zones identified above to get an average density value for each given zone. Then the two average density values corresponding to the distal and mesial zones of a given arch (upper or lower) were compared through a two-tail t-test at P=.05 (sub-antral versus pre-antral, retroforaminal versus interforaminal). With the same statistical approach, bone density values of corresponding upper and lower regions of the jaws were compared (pre-antral, versus interforaminal, sub-antral versus retroforaminal). Additionally, the bone density values corresponding to the overall means of the measurements assessed in the mandible and in the maxilla were calculated and compared with a two samples, two-tails, t-test (P=.05). All values were given as mean ± standard error of mean (s.e.m).
Single bone density measurements were not shown for the sake of brevity. No statistical differences among the measurements recorded by the 39 operators were detected (Table 1), indicating that the measurements provided by the device were operator-independent.
"Implant success strictly relies on the correct evaluation of bone density at implant placement site, which is a strong predictor of primary stability and allows to make proper decisions about site preparation and the immediate loading protocol viability.15,16
The device used in the present study has already been shown to provide reliable bone density measurements, with a significant correlation with histomorphometric data,13 and to distinguish pre-defined zones with different bone densities.14 In addition, the present investigation showed that the system provides objective, operator-independent measurements, not based on subjective perception as current classifications do, thus allowing to adjust the site preparation according to the results of the measure. Clinical consequences could be of high interest. The availability of an objective measurement of bone density at the placement site enables a reliable intra-surgical diagnosis of bone density, and could affect clinical practice positively in two ways. Firstly, a reliable rationale for tunnel preparation could be formulated, providing well-defined criteria for under-preparation at low-density sites. Secondly, the availability of a torque-depth graph, showing how bone density varies along the implant placement site, could lead to further refinements of the implant placement strategy also in relation to the loading protocol (delayed vs. early or immediate), or even to come to a definitive decision about the loading protocol itself after implant placement, according to actual, objective bone density values recorded with the device. Further studies should be aimed to formulate such criteria. It is worth noticing that the device allows measurement of the same quantities (i.e., average and peak torque values, and torque-depth curve integral) also at implant insertion, providing additional data about implant primary stability and about the implant-bone relation at site of placement. Further studies should also be aimed to investigate if loading protocols may be adjusted according to the measurements recorded at implant insertion.
Figures and Tables
![]() | Fig. 3The torque/depth plot generated by the device. I = integral (corresponding to the area below the curve); CM = average torque; N = progressive measurement number; P = depth; CP = maximum torque. GO/STOP = pre-set depth the probe stops at. |
![]() | Fig. 4Statistical comparison of overall means of bone density measurement collected in the four anatomical zones, a pre-antral (anterior) and a sub-antral (posterior) zone in the upper maxilla, and an interforaminal (anterior) and a retroforaminal (posterior) zone in the lower jaw.
*: statistically significant difference
Cm: average torque
|
Table 1
Statistical comparison of the data recorded by the different operators in the implant placement sites
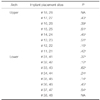
References
1. Davies JE. Mechanisms of endosseous integration. Int J Prosthodont. 1998; 11:391–401.
2. Romanos GE. Bone quality and the immediate loading of implants-critical aspects based on literature, research, and clinical experience. Implant Dent. 2009; 18:203–209.
3. Bahat O, Sullivan RM. Parameters for successful implant integration revisited part I: immediate loading considered in light of the original prerequisites for osseointegration. Clin Implant Dent Relat Res. 2010; 12:e2–e12.
4. Turkyilmaz I, Ozan O, Yilmaz B, Ersoy AE. Determination of bone quality of 372 implant recipient sites using Hounsfield unit from computerized tomography: a clinical study. Clin Implant Dent Relat Res. 2008; 10:238–244.
5. Shapurian T, Damoulis PD, Reiser GM, Griffin TJ, Rand WM. Quantitative evaluation of bone density using the Hounsfield index. Int J Oral Maxillofac Implants. 2006; 21:290–297.
6. Turkyilmaz I, Tözüm TF, Tumer C. Bone density assessments of oral implant sites using computerized tomography. J Oral Rehabil. 2007; 34:267–272.
7. Valiyaparambil JV, Yamany I, Ortiz D, Shafer DM, Pendrys D, Freilich M, Mallya SM. Bone quality evaluation: comparison of cone beam computed tomography and subjective surgical assessment. Int J Oral Maxillofac Implants. 2012; 27:1271–1277.
8. Mah P, Reeves TE, McDavid WD. Deriving Hounsfield units using grey levels in cone beam computed tomography. Dentomaxillofac Radiol. 2010; 39:323–335.
9. Nomura Y, Watanabe H, Honda E, Kurabayashi T. Reliability of voxel values from cone-beam computed tomography for dental use in evaluating bone mineral density. Clin Oral Implants Res. 2010; 21:558–562.
10. Lekholm U, Zarb GA. Patient selection and preparation. In : Branemark PI, Zarb GA, Albrektsson T, editors. Tissue-integrated prostheses: osseointegration in clinical dentistry. Chicago: Quintessence;1985. p. 199–209.
11. Misch CE. Density of Bone: effects on surgical approach and healing. In : Misch CE, editor. Contemporary Implant Dentistry. St. Louis: CV Mosby;2008. p. 645–667.
12. Trisi P, Rao W. Bone classification: clinical-histomorphometric comparison. Clin Oral Implants Res. 1999; 10:1–7.
13. Iezzi G, Scarano A, Di Stefano D, Arosio P, Doi K, Ricci L, Piattelli A, Perrotti V. Correlation between the bone density recorded by a computerized implant motor and by a histomorphometric analysis: a preliminary in vitro study on bovine ribs. Clin Implant Dent Relat Res. 2015; 17:e35–e44.
14. Di Stefano DA, Arosio P, Pagnutti S. A possible novel objective intraoperative measurement of maxillary bone density. Minerva Stomatol. 2013; 62:259–265.
15. Ekfeldt A, Christiansson U, Eriksson T, Lindén U, Lundqvist S, Rundcrantz T, Johansson LA, Nilner K, Billström C. A retrospective analysis of factors associated with multiple implant failures in maxillae. Clin Oral Implants Res. 2001; 12:462–467.
16. Javed F, Romanos GE. The role of primary stability for successful immediate loading of dental implants. A literature review. J Dent. 2010; 38:612–620.