Abstract
PURPOSE
This study aimed to discover a way to increase the bond strength between bis-acryl resins, using a comparison of the shear bond strengths attained from bis-acryl resins treated with light curing, pressure, oxygen inhibition, and heat.
MATERIALS AND METHODS
Self-cured bis-acryl resin was used as both a base material and as a repair material. Seventy specimens were distributed into seven groups according to treatment methods: pressure - stored in a pressure cooker at 0.2 Mpa; oxygen inhibition- applied an oxygen inhibitor around the repaired material,; heat treatment - performed heat treatment in a dry oven at 60℃, 100℃, or 140℃. The shear bond strength was measured with a universal testing machine, and the shear bond strength (MPa) was calculated from the peak load of failure. A comparison of the bond strength between the repaired specimens was conducted using one-way ANOVA and Tukey multiple comparison tests (α=.05).
RESULTS
There were no statistically significant differences in the shear bond strength between the control group and the light curing, pressure, and oxygen inhibition groups. However, the heat treatment groups showed statistically higher bond strengths than the groups treated without heat, and the groups treated at a higher temperature resulted in higher bond strengths. Statistically significant differences were seen between groups after different degrees of heat treatment, except in groups heated at 100℃ and 140℃.
Materials commonly used to fabricate provisional restorations include polyethyl methacrylate (PEMA), polymethyl methacrylate (PMMA), polyvinyl methacrylate, and bisacryl. Among these materials, bis-acryl resin has become popular as a material for provisional restorations because it is convenient to use, delivers a low exothermic reaction, and causes less polymerization shrinkage.1,2
In general, the chemical similarity between materials being bonded has a great effect on the bond strength between the polymers involved.3 Therefore, employing a repair resin that is chemically similar to the provisional base appears to deliver a higher bond strength than that of other materials that have a dissimilar composition.3 However, in contrast to this rationale the bond strength between bisacryl and bis-acryl is considered weak,4 and the difficulty of using it in repair is therefore seen to be a critical shortcoming of bis-acryl resin. To overcome this limitation, some authors have suggested the use of light-cured flowable composites as a repair material for bis-acryl provisional restoration. Hagge et al.4 showed that air abrasion, followed by the application of a flowable composite, can be a successful method of repair for use with bis-acryl composite. Through case reports, Bohnekamp and Garcia,5 and Hammond et al.6 demonstrated efficacy of the use of flowable composite as a method of bis-acryl provisional restoration, although the resistance of the repaired composite to fracture using this method has still not yet been proven.3
Repairing a provisional bis-acryl restoration using bisacryl would simplify the process of repair, and the bond strength of the repaired material would be expected to surpass that of bis-acryl and flowable composites. However, no reports have yet determined a method to achieve strong bond strength between bis-acryl resins. The purpose of this in vitro study is therefore to determine an appropriate way of increasing the bond strength between bis-acryl resins, by comparing the shear bond strength between bis-acryl resins obtained using different treatments involving light curing, pressure, oxygen inhibition, and heat.
Self-curing bis-acryl resin (Luxatemp AM Plus, DMG, Hamburg, Germany; shade A2, Lot 698382) was used as the base material and repair material. The shear bond strength test required the use of a sample holder to fix the specimen in the universal testing machine, perpendicular to the orientation of the shear force, and transparent acrylic glass rods (Polymicar, Tae-guang, Korea) were fabricated for this purpose. The mixed self-curing bis-acryl resin was dispensed into a 12-mm-wide hole in the acrylic glass rod and was allowed to set for 10 minutes.
Prior to repair, the surfaces of the specimens were ground with silica carbide (SiC) paper (grit 180), rinsed with water for 10 seconds, and dried for 30 seconds using an air syringe. Hard transparent gelatin capsules (4.0 mm in diameter) were used as matrices for the production of columns of relining materials bonded to the bis-acrylic provisional resin surface. The capsule was partially filled with repair materials to limit the thickness of the bonded material to 2 mm (Fig. 1).
Immediately after specimen preparation, the specimens were treated in the following different ways for 20 minutes: CONTROL - room temperature without any treatment, LIGHT CURING - exposed the top surface of the specimen to a light curing unit (Drs light, Good Doctors Co., Jungcheon, Incheon, Korea), PRESSURE - stored in a pressure cooker (PSPC-24C, PN Poongnyun, Danwon, Ansan, Korea) without waater at 0.2 Mpa, OXYGEN INHIBITION - applied an oxygen inhibitor (Oxyguard II, Kuraray Noritake Dental Inc., Kurashiki, Okayama, Japan) around the repaired material, and HEAT - applied heat using a dry oven (WiseVen, Daihan Scientific Co., Yeong-deungpo, Seoul, Korea) at 60℃, 100℃, or 140℃. Each group involved 10 specimens and Table 1 describes the groups tested.
After the treatments were applied, the shear bond strength was measured with a universal testing machine (AG-10KNX, Shimadzu Co., Kyoto, Japan). A knife-edge shearing rod was used at a crosshead speed of 1 mm/min to place a load on the specimens until fracture occurred. The shear bond strength (MPa) was calculated from the peak load of failure. The aspect of each bonding failure was determined and recorded using a video measuring system (Optical video measuring system, Seven Ocean, Seoul, Korea) at 10× magnification.
The mean and standard deviation of the shear bond strengths were calculated for each treatment group. The data was evaluated for homogeneity of the variance based on the Levene test (α=.05). A comparison of the bond strength between repaired specimens was conducted by one-way ANOVA and Tukey multiple comparison tests (α <.05). All statistical analyses were carried out with SPSS for Windows (release 12.01; SPSS Inc., Chicago, IL, USA).
The results of the shear bond strength for each experimental group are summarized in Fig. 2. The detachment results for the groups treated at room temperature indicates that group 3 (pressure) had the highest shear bond strength followed by group 4 (oxygen inhibition), group 2 (light curing), and group 1 (control). However, there were no statistically significant differences between the groups treated at room temperature.
The heat treatment groups (Groups 5-7) showed statistically higher bond strengths than the groups treated without heat (Groups 1-4), and the groups treated at a higher temperature resulted in higher bond strengths. There were also statistically significant differences between group 5 (heat/60℃) and group 6 (heat/100℃) (P<.05), but there were no statistically significant differences between group 6 (heat/100℃) and group 7 (heat/140℃). There was a burn odor recorded during heat treatment at 140℃, but there was no trace of burn on the specimen. All of the groups treated at room temperature (Groups 1-4) showed adhesive failure and all of groups subjected to heat treatment (Groups 5-7) showed cohesive failure. Most cohesive failures took place in the base material of the specimens, but four specimens of group 7 (heat/140℃) showed cohesive failure of the repaired material.
There is little available information concerning methods to increase the bond strength of dental composites especially for bis-acryl resin, but light curing,7 pressure,8 oxygen inhibition,9 and heat10 have been proposed to fortify the mechanical properties of resin composites. Therefore, to achieve the purpose of this in vitro study, finding the way to increase the bond strength between bis-acryl resins, light curing, pressure, oxygen inhibition and heat was selected as variables of the experiment.
The effects of light-curing, pressure, oxygen inhibition, and heat treatments on the shear bond strength between bis-acryl composites were evaluated. Among the above identified treatment methods, only the application of heat had an effect on the shear bond strength between bis-acryl resins and strong bond strength could be achieved using heat treatment after 20 minutes of bonding.
Although there are few available studies involving the heat treatment of bis-acryl, investigations of the heat treatment of other dental resins showed that increasing wear resistance,11 tensile strength,12,13 fracture toughness14 and hardness15 were obtained by applying heat treatment. These reports concluded that the increased mechanical properties resulting from heat treatment was related to an increase of the degree of cure. Therefore, the effect of heat treatment on dental resins prompts the degree of cure. The degree of cure of bis-acryl is lower than other provisional restoration materials16 and it can be improved more than other provisional restoration materials by heat treatment. In conclusion, the dramatic increase in bond strength between bisacryl obtained by heat treatment in this study seems to be related to the low degree of cure of bis-acryl and the accelerated cure of bis-acryl by heat treatment.
The optimal heat treatment for composite resin was 125℃ for a total of 7.5 minutes,17 but there is no available information about the proper heat temperature for the treatment of bis-acryl. In our study, there were no significant differences between the groups heat-treated at a temperature of 100℃ and groups treated at a temperature of 140℃. During heat treatment at 140℃, a burning odor was smelt and only specimens heat-treated at 140℃ showed cohesive failure of the repaired material. Therefore, it can be assumed that the strongest bonding between bis-aryl resins was achieved from heat treatment around a temperature of 100℃ for a time period of 20 minutes and the chemical deformation of bis-acryl occurred at a heat treatment temperature between 100℃ and 140℃.
Ferracane and Condon18 showed that shorter heat treatment was sufficient to enhance the mechanical properties of a composite resin compared to heat treatment for different durations (3 hours and 10 minutes) at the same temperature (120℃). The duration of heat treatment is also an important factor, although it was not considered in this study. Indeed, although a strong bond between bis-acryl resins was achieved through heat treatment of 20 minutes, there are concerns about shrinkage and deformation of bisacryl resin due to the heat treatment. Therefore, in order to apply the results of this study to the clinical setting, more data regarding time factors of heat treatment and the other effects of heat on the mechanical properties of bis-acryl resin are required.
Figures and Tables
Fig. 1
Schematic figure of specimen; a- acrylic glass rod, b- bis-acryl base material, c- gelatin capsule, d- bis-acryl repair material.
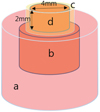
References
1. Young HM, Smith CT, Morton D. Comparative in vitro evaluation of two provisional restorative materials. J Prosthet Dent. 2001; 85:129–132.
2. Tjan AH, Castelnuovo J, Shiotsu G. Marginal fidelity of crowns fabricated from six proprietary provisional materials. J Prosthet Dent. 1997; 77:482–485.
3. Chen HL, Lai YL, Chou IC, Hu CJ, Lee SY. Shear bond strength of provisional restoration materials repaired with light-cured resins. Oper Dent. 2008; 33:508–515.
4. Hagge MS, Lindemuth JS, Jones AG. Shear bond strength of bis-acryl composite provisional material repaired with flowable composite. J Esthet Restor Dent. 2002; 14:47–52.
5. Bohnenkamp DM, Garcia LT. Repair of bis-acryl provisional restorations using flowable composite resin. J Prosthet Dent. 2004; 92:500–502.
6. Hammond BD, Cooper JR 3rd, Lazarchik DA. Predictable repair of provisional restorations. J Esthet Restor Dent. 2009; 21:19–24.
7. Tanoue N, Matsumura H, Atsuta M. Comparative evaluation of secondary heat treatment and a high intensity light source for the improvement of properties of prosthetic composites. J Oral Rehabil. 2000; 27:288–293.
8. Murakami N, Wakabayashi N, Matsushima R, Kishida A, Igarashi Y. Effect of high-pressure polymerization on mechanical properties of PMMA denture base resin. J Mech Behav Biomed Mater. 2013; 20:98–104.
9. Velazquez E, Vaidyanathan J, Vaidyanathan TK, Houpt M, Shey Z, Von Hagen S. Effect of primer solvent and curing mode on dentin shear bond strength and interface morphology. Quintessence Int. 2003; 34:548–555.
10. Bausch JR, de Lange C, Davidson CL. The influence of temperature on some physical properties of dental composites. J Oral Rehabil. 1981; 8:309–317.
11. Lutz F, Phillips RW, Roulet JF, Setcos JC. In vivo and in vitro wear of potential posterior composites. J Dent Res. 1984; 63:914–920.
12. Wendt SL Jr. The effect of heat used as secondary cure upon the physical properties of three composite resins. II. Wear, hardness, and color stability. Quintessence Int. 1987; 18:351–356.
13. Covey DA, Tahaney SR, Davenport JM. Mechanical properties of heat-treated composite resin restorative materials. J Prosthet Dent. 1992; 68:458–461.
14. Cook WD, Johannson M. The influence of postcuring on the fracture properties of photo-cured dimethacrylate based dental composite resin. J Biomed Mater Res. 1987; 21:979–989.
15. Wendt SL Jr. The effect of heat used as a secondary cure upon the physical properties of three composite resins. I. Diametral tensile strength, compressive strength, and marginal dimensional stability. Quintessence Int. 1987; 18:265–271.
16. Ha JY, Kim SH, Kim KH, Kwon TY. Influence of the volumes of bis-acryl and poly(methyl methacrylate) resins on their exothermic behavior during polymerization. Dent Mater J. 2011; 30:336–342.
17. Wendt SL Jr. Time as a factor in the heat curing of composite resins. Quintessence Int. 1989; 20:259–263.
18. Ferracane JL, Condon JR. Post-cure heat treatments for composites: properties and fractography. Dent Mater. 1992; 8:290–295.