Abstract
PURPOSE
The aim of this study was to evaluate the effects of atmospheric plasma (APL) versus conventional surface treatments on the adhesion of self-adhesive resin cement to Ti-6Al-4V alloy.
MATERIALS AND METHODS
Sixty plates of machined titanium (Ti) discs were divided into five groups (n=12): 1) Untreated (CNT); 2) Sandblasted (SAB); 3) Tribochemically treated (ROC); 4) Tungsten CarbideBur (TCB); 5) APL treated (APL). SEM analysis and surface roughness (Ra) measurements were performed. Self-adhesive resin cement was bonded to the Ti surfaces and shear bond strength (SBS) tests, Ra and failure mode examinations were carried out. Data were analyzed by one-way analysis of variance and chi-squared test.
Titanium (Ti) and its alloys are widely used as dental materials in prosthodontics and implantology because of their low density, high corrosion resistance, superior biocompatibility and good mechanical properties.1 Using titanium as a prosthetic superstructure requires a strong and durable bonding of cement to the titanium to resist various challenges in the oral environment.234
Adhering a luting cement to a surface depends on both micromechanical interlocking and physicochemical bonding.5 Many different surface modification techniques have been proposed for increasing the binding and are classified as: micromechanical retention, chemical bonding, and micromechanical retention and chemical bonding.6
To provide micromechanical retention, airborne-particle abrasion with Al2O3 particles is typically used.34789 Additionally, surface roughening with a bur has been reported to be quite practical and safe method for modifying the material surface.10 These methods change the wettability characteristics of dental materials for improved adhesion and bonding. These procedures also clean the surface and increase the surface area.3478910
Chemical coupling can be obtained with metal primers and silanes. Metal primers contain active monomers such as 10-methacryloyloxydecyl-dihydrogen phosphate (MDP), 4-methacryloxyethyl trimellitate anhydride (4-META) and others that react chemically with the oxides present on the metal surface.11 Silanes, due to their bifunctional characteristics, establish a chemical bond between the resin matrix and the metal surface.12
One method known to achieve both micromechanical retention and chemical bonding is tribochemical silica coating, which uses airborne-particle abrasion with silica-modified Al2O3 particles. This method consists of a coating of abrasive sand, a silane coupling agent, a light-cured bonding resin and an opaque resin. This surface treatment provides a combination of micromechanical and chemical adhesion.34610
Plasma treatment has been frequently used to modify the surface of products in the industrial sector.1314 Plasma is an electrically neutral ionized gas, electrons,ultraviolet irradiation andfree radicals under normal pressure conditions.14 Previously, this technique has been successfully used to deactivate bacteria even in bio-films on titanium surfaces.1516 Plasma irradiation does not cause environmental pollution, as it does not require chemical additives.13 Plasma temperature is depending on the input power and varying according to the distance of the plasma flame. Its temperature can range between 37℃ and 62℃, which is why it was defined biocompatible.17 It was reported that, with the proper application of plasma treatment, an oxide layer is formed and surface becomes hydrophilic nature, which may influence the surface adhesion properties.18 As distinct from a high pressure plasma requires a complex reactor, atmospheric plasma may operate at atmospheric pressure. A plasma device that works at an atmospheric pressure can be easily used in the oral environment. Since the first report on low temperature atmospheric pressure plasma (APP) in 1968, the homogeneous dielectric barrier discharge in atmospheric air has been explored in many applications ranging from industry to biology and medicine.131415161718192021
Although numerous studies have evaluated the bond strength of resin cements to Ti alloys, very few have compared the effects of different surface treatments including roughening with a bur, sandblasting (conventional surface treatment), tribochemical silica coating, and plasma treatment (atmospheric pressure), on bond strength. The adhesion mechanism of Ti and the adhesive luting cements remains unclear.22 Various publications are mentioned that there have been many different surface treatment techniques which increase the bond strength.345678111222
The purpose of this study was to evaluate the effects of different surface treatments on the bond strength of a resin cement to Ti Grade 5 alloy. The null hypothesis was that the use of different surface treatments would not influence the shear bond strength at the titanium/cement interface. This test was intended to demonstrate the effect of atmospheric plasma treatment versus conventional surface treatment methods.
Sixty plates of machined Ti Grade 5 (Ti-6Al-4V) alloy (Biotecsrl, Povolaro di Dueville, Italy) discs (10 mm in diameter and 2.0 mm thick) were machined and then cleaned in an ultrasonic bath (Sonorex, Bandelin, Berlin, Germany) filled with distilled water for 5 minutes.
The specimens were randomly divided into five experimental groups (n=12) that were subjected to one of the following surface treatment techniques: 1) Untreated control (Group CNT); 2) Sandblasted (Group SAB); 3) Tribochemically surface treated (Group ROC); 4) Roughened with a tungsten carbide bur (Group TCB); and 5) Plasma treated (Group APL).
For Group CNT, the test surface was polished with silicone carbide abrasive paper from 600 through 1200 grit (English Abrasives: London, England) under water irrigation.
For Group SAB, the sample surface was cleaned to remove residues and dried before the surface was blasted with 110 µm high-purity aluminum oxide (Al2O3) sand (Rocatec™ pre, 3M ESPE, Seefeld, Germany, Lot No.: 372623) for 15 seconds at a pressure of 2.8 bar from a vertical angle, and the distance was maintained at 10 mm from the specimen surface via the coating unit (Rocatec™ Junior, 3M ESPE, Seefeld, Germany).
For Group ROC, the sample surface was blasted with Rocatec™ pre as described above. This was followed by tribochemical coating of the microblasted surface with 110 µm silica-modified aluminum oxide (Al2O3+SiO2) sand (Rocatec™ plus, 3M ESPE, Seefeld/Germany, Lot: 371315) according to the manufacturer's instructions. The blast pressure was adjusted to 2.8 bar to ensure an adequately high level of energy for creating the triboplasma. Any residual blast-coating agent was removed with a stream of oil-free air. After the Rocatec™ plus surface treatment, the silane solution (ESPE™ Sil, 3M ESPE, Seefeld, Germany, Lot No.: 391601) was applied to the bonding surface and the solution was allowed to dry for 5 minutes. During this time, care was taken to prevent any impurities from contacting the silanized surface.
For Group TCB, to improve mechanical retention, the bonding surface of the specimens was drilled with a cylindrical tungsten carbide bur (Jota AG Rotary Instruments, Rüthi, Switzerland, Lot No.: 779929), rotating at 40,000 rpm with consistent force under water spray. In order to provide a standard application, drilling was performed in parallel to the sample surface and cylindrical cavities of approximately equal volume (4 mm wide, 2 mm deep) were obtained with the use of a milling device (Paraskop M, Model No. 26060, Bego, Bremen, Germany) and drill depth was controlled.
For Group APL, plasma surface treatment was performed with an atmospheric plasma torch (Plasmatreat GmbH, Bisamweg 10, Steinhagen, Germany) to modify the titanium surfaces for bonding. This device does not require any special gas and generates plasma at room temperature. It was operated at a frequency of 21 kHz with an applied voltage of 5 kV. The treatment speed was 5 m/min. with pressure of 2 bar, and the approximate jet power was 1 kVA. It was used for 5 seconds, with a distance of 5 mm between the nozzle and the test surface. It was attempted to always keep the discs within the plasma. Manufacturer information of the materials usedwas shown in detail at Table 1.
Following the surface modification procedures, all specimens were rinsed under distilled water and exposed to dry air spray; then, the samples were placed in a slot prepared in polymethyl methacrylate resin (Meliodent, Bayer Dental, Newbury, UK) that covered with polyvinyl chloride tube (20.0 mm in diameter and 27.0 mm high).
SEM Analysis: One fractured specimen from each group was examined under a scanning electron microscope (SEM, JSM-5600 Scanning Microscope; JEOL Ltd., Tokyo, Japan). Representative specimens were captured at ×500 magnification.
Surface Roughness Measurements: After each surface treatment, surface roughness measurements were carried out using a profilometer (Perthometer M2, Mahr GmbH, Göttingen, Germany) to measure the Ra (average roughness height) in micrometers (µm). The roughness measurement surfaces of samples were not used for the shear bond test because the profilometer destroyed the surface. The mean roughness value was calculated from 3 single measurements.
Resin Cement Bonding: RelyX™ U 200 self-adhesive resin cement (3M Deutschland GmbH, Neuss-Germany, Lot: 517597) was condensed into an Ultradent Teflon mold (Ultradent Product, Inc., Utah, USA) and cured for 40 s.as proposed by the manufacturer. The specimens were clenched in the Ultradent Bonding Jig (Ultradent Products Inc., South Jordan, UT, USA), and Teflon molds (2.3 mm in internal diameter, 3 mm in height) were used to buildand conjunct the resin cement on the Ti plate surface (Fig. 1). After the cement had set via light curing (VOCO Celalux S/N, VOCO GmbH, Cuxhaven, Germany), the Teflon mold was disassembled.
Shear Bond Strength tests: SBS tests were conducted with a mechanical testing machine (Lloyd-LRX, Lloyd Instruments, Fareham, UK) with a 1 N load cell. A Sharp blade shearing sleeve, operating at a crosshead speed of 0.5 mm/min was used to apply a parallel shearing load as closely as possible to contact the cement/titanium interface. The failure strength was recorded in megapascal (MPa) unit.
Stereomicroscopy: Each specimen was examined under a stereomicroscope (MZ 12; Leica Microsystems; Glattbrugg, Switzerland) at ×20 magnification, and images were observed and analyzed with a software. The failure mode was scored by the same evaluator as either adhesive (between titanium and resin cement), cohesive (within the resin cement), or combined (areas of adhesive and cohesive failure). For the last classification, the adhesive area was divided into quadrants, and the pre-dominant type of failure was observed in each specimen according to the method of Santos et al.23
Statistical Analysis: SBS and Ra values were analyzed by one-way analysis of variance (ANOVA). According to assumption of homogeneity of variance, post-hoc Tukey's and Games-Howell multiple comparison tests were used for SBS and Ra values, respectively. Statistical significance was set at the 0.05 probability level. In addition, the failure mode data were analyzed by the chi-squared test.
The results of one-way ANOVA (Table 2) indicated that the surface treatment methods affected the bonding and roughness values significantly. Table 3 and Fig. 2 show the mean SBS values (MPa), mean Ra values (µm) and standard deviations for each group, as well as the statistical analysis results.
SBS: The lowest shear bond strength values were obtained for the CNT group and significantly differ from all other groups except from the APL group. The ROC group showed the highest test results and is significantly different from the other groups (P<.05) except from the TCB group (P>.05). Comparisons of the test values that were obtained for the CNT and APL groups, the SAB and TCB groups, and the ROC group and the TCB groups did not show significant differences (P>.05). When the groups abraded with 110 µm Al2O3 particles were compared, it was observed that silica-modified 110 µm Al2O3 (Rocatec™ plus) promoted significantly higher SBS than 110 µm Al2O3 alone (P<0.05).
Ra: The ROC group showed the highest surface roughness value of all the groups (Table 3). There were significant differences between all groups (P<.05) in terms of surface roughness. In support of this finding, SEM examination showed that groups with different Ra values had different surface topography from that of the control group.
SEM: The original CNT surface was in a roughened form because of the abrasive paper, which was no longer visible after treatment with SAB, ROC and TCB. SEM images revealed that more grooves were present in the SAB and ROC groups than in the TCB and APL groups (Fig. 3). For the SAB and ROC groups, there is no difference in terms of surface rheology, and they have deep macro cavities and pits coated with micro gaps, which possibly resulted from the high impact of the abrasive particles. The Ti surface appeared rough, with irregular, embedded alumina and silica particles. In the APL group, a superficially fissured coarse surface with carbon spots was observed, but the morphological characteristics of the Ti surface did not differ in any important way from that of the CNT group.
The results of this study demonstrate that the APL group showed the lowest values in terms of both surface roughness and bond strength. In contrast, the ROC group presented significantly higher surface roughness and bond strength values than the other groups (P<.05). Although the ROC-TCB and SAB-TCB groups had similar bond strengths internally, there were no significant differences between these pairs. Therefore, the null hypothesis was rejected.
It has been reported that a strong and durable bond at the metal/cement interface depends on a combination of micromechanical retention and chemical bonding. In cementation with non-adhesive resin cements, both bonding mechanisms (chemical and micromechanical) must be ensured.34 However as shown in the present study, in the case of self-adhesive resin cements, an appropriate adhesion can be obtained by abrasion with airborne Al2O3 particles8 and roughening with a bur to increase surface area.10
In this study, two different chemical compositions were used for the airborne-particle abrasion: Al2O3 particles- (Rocatec™ pre) and silica-modified Al2O3 particles (Rocatec™ plus). Sandblasting of a material surface is often used to obtain a micro-retentive topography and increased surface area; thus, the wettability of the material can be increased.7
Significantly higher bond strength was observed for samples having both micromechanical retention and chemical bonding with the silica coating system (Rocatec™ plus) than for samples treated by airborne-particle abrasion with 110 µm Al2O3 particles alone (P<.05). This result was in agreement with the findings of previous investigations.26272829 Significant differences were observed between the SAB and ROC groups for both Ra and SBS values (P<.05). A possible explanation for these statistical results is the superiority of combined micromechanical retention and chemical bonding that was provided with the SiO2 coating of the same-sized Al2O3 particles.
There are many studies on the effects of atmospheric plasma surface treatment on the adhesion of certain types of dental material, primarily for polymers18192030323334 and less so for zirconia2431 and titanium.142231 Some authors have reported that plasma surface modification procedures allow the formation of stronger bonds, improve wettability, and produce chemically active sites for the adhesion of other molecules.213031 To the best of our knowledge, there is limited information in the dentistry literature about the effects of plasma treatment of titanium surfaces on surface roughness and adhesion.
Valverde et al.24 detected a significant increase in the micro-tensile bond strength to Y-TZP surfaces when non-thermal plasma was applied alone or in combination with ceramic primer and/or Al2O3 sandblasting. In addition, Silva et al.31 used surface characterization to investigate the effects of non-thermal plasma exposure on Ti and Y-TZP and reported that the plasma treatment of both material surfaces resulted in increases in surface energy in parallel with increases of the execution time.
However, in the present study, the APL group had the lowest value for bond strength after the control group. This may be attributed to effects caused by the time of treatment, the range of applied power, the waveforms of the applied voltage or the distance between the sample surface and the plasma applicator nozzle, as noted by many publications.20213133
Han et al.21 and Cho et al.34 demonstrated that the application of non-thermal APP provided much lower adhesive strength than that obtained with conventional hydrofluoric acid etching and silane application to enhance the adhesion of composite resin to dental ceramic, which is in line with our findings. However, these authors2134 also emphasized that this approach could contribute to the enhancement of bond strength with dental adhesives.
Roughening the Ti surface increases surface area and wettability; it also provides mechanical interlocking with resin cements and is considered to be a significant factor influencing bond strength.63536 In this study, airborne-particle abrasion of the ROC and SAB groups resulted in greater degrees of roughness on the Ti surface than did the other treatments (Table 3). An increase in the surface roughness leads to an increase in surface area, which, in turn, may improve the Ti-resin cement bonding. However, in the comparison between groups for bond strength and roughness values, this was not the case. For surface roughness measurements, the TCB group value was less than the SAB group value. However, the shear bond strength value of the TCB group was higher than that of the SAB group (Table 3), which reveals that roughening the surface of the titanium does not increase the bonding strength. Therewithal, it was mentioned that surface roughness causes intense stress locations at the metal-cement interface and forms sharp angled edgesthat could avoid completely wetting.37 This result of the present study is in agreement with those of some previous studies.35363738
This experimental study revealed that an inadequate Ti/resin-cement bond was produced in the untreated control group. The analysis of failure modes showed variations in the test groups. Mixed failures occurred more frequently in groups with higher bond strength values (Table 4). This finding is in accordance with those of previous studies.3839
According to the requirements of ISO 10477,40 the minimum acceptable SBS value at the interface of resin-based materials and substrate is 5 MPa. Matsumura et al.41 reported that the resin-to-metal SBS value should be at least 10 MPa for clinically acceptable results. In the present study, SBS values above 10 MPa were observed in all groups except for the APL and CNT groups.
Plasma treatment did not bring about any structural change in the titanium surface, in contrast to treatments such as sandblasting and treatment with Rocatec™ plus, which can change the surface of the titanium morphologically.
Limitation of this study is the use of certain parameters for plasma application. Future studies are needed to evaluate in different execution time and applied power of plasma discharge which may bring different results. Also, only one category of dental cement and one type of titanium were tested; these findings may not apply to other cements or titanium alloy materials.
Figures and Tables
Fig. 2
Mean ± standard deviation of the shear bond strength (MPa) and surface roughness (µm) values.
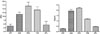
Fig. 3
Representative SEM photomicrographs of treated Ti surface (×500). (A) Control (CNT)-grinding marks, (B) Sandblasted (SAB) and (C) Rocatec-Plus (ROC)-deep macro cavities and pits coated with micro gaps with irregular, embedded alumina and silica particles, (D) Roughened with tungsten carbide bur (TCB)-irregular corrugated titanium surface, (E) Atmospheric plasma treatment (APL)-a superficially fissured coarse surface with carbon spots.
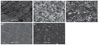
Fig. 4
Stereomicroscope images of failure mode between resin cement and Ti surface: (A) Adhesive failure mode, (B) Cohesive failure mode, (C) Mix failure mode.
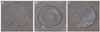
Table 1
Materials evaluated
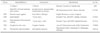
Table 2
One-way analysis of variance (ANOVA) results for mean shear bond strength (SBS) and mean surface roughness (Ra) values

Source of variation | SBS (MPa) | Ra (µm) | ||||||||
---|---|---|---|---|---|---|---|---|---|---|
SS | df | MS | F | Sig.* | SS | df | MS | F | Sig.* | |
Between groups | 1652.7 | 4 | 413.1 | 32.7 | .000 | 35.3 | 4 | 8.8 | 448.6 | .000 |
Within groups | 693.3 | 55 | 12.6 | 1.0 | 55 | 0.02 | ||||
Total | 2346.0 | 59 | 36.3 | 59 |
Table 3
Mean ± standard deviation (SD) of the shear bond strength (SBS) and surface roughness (Ra) values
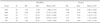
References
1. McCracken M. Dental implant materials: commercially pure titanium and titanium alloys. J Prosthodont. 1999; 8:40–43.
2. Berg E, Davik G, Hegdahl T, Gjerdet NR. Hardness, strength, and ductility of prefabricated titanium rods used in the manufacture of spark erosion crowns. J Prosthet Dent. 1996; 75:419–425.
3. Ozcan M, Pfeiffer P, Nergiz I. A brief history and current status of metal-and ceramic surface-conditioning concepts for resin bonding in dentistry. Quintessence Int. 1998; 29:713–724.
4. Tsuchimoto Y, Yoshida Y, Takeuchi M, Mine A, Yatani H, Tagawa Y, Van Meerbeek B, Suzuki K, Kuboki T. Effect of surface pre-treatment on durability of resin-based cements bonded to titanium. Dent Mater. 2006; 22:545–552.
5. Asmussen E, Peutzfeldt A. Surface energy characteristics of adhesive monomers. Dent Mater. 1998; 14:21–28.
6. Fonseca RG, Haneda IG, Almeida-Júnior AA, de Oliveira Abi-Rached F, Adabo GL. Efficacy of air-abrasion technique and additional surface treatment at titanium/resin cement interface. J Adhes Dent. 2012; 14:453–459.
7. Inan O, Acar A, Halkaci S. Effects of sandblasting and electrical discharge machining on porcelain adherence to cast and machined commercially pure titanium. J Biomed Mater Res B Appl Biomater. 2006; 78:393–400.
8. Fonseca RG, de Almeida JG, Haneda IG, Adabo GL. Effect of metal primers on bond strength of resin cements to base metals. J Prosthet Dent. 2009; 101:262–268.
9. Akın H, Ozkurt Z, Kırmalı O, Kazazoglu E, Ozdemir AK. Shear bond strength of resin cement to zirconia ceramic after aluminum oxide sandblasting and various laser treatments. Photomed Laser Surg. 2011; 29:797–802.
10. Bertolotti RL. Adhesion to porcelain and metal. Dent Clin North Am. 2007; 51:433–451.
11. Taira Y, Matsumura H, Yoshida K, Tanaka T, Atsuta M. Adhesive bonding of titanium with a methacrylate-phosphate primer and self-curing adhesive resins. J Oral Rehabil. 1995; 22:409–412.
12. Abi-Rached Fde O, Fonseca RG, Haneda IG, de Almeida-Júnior AA, Adabo GL. The effect of different surface treatments on the shear bond strength of luting cements to titanium. J Prosthet Dent. 2012; 108:370–376.
13. Nishigawa G, Maruo Y, Oka M, Okamoto M, Minagi S, Irie M, Suzuki K. Effect of plasma treatment on adhesion of self-curing repair resin to acrylic denture base. Dent Mater J. 2004; 23:545–549.
14. Duske K, Koban I, Kindel E, Schröder K, Nebe B, Holtfreter B, Jablonowski L, Weltmann KD, Kocher T. Atmospheric plasma enhances wettability and cell spreading on dental implant metals. J Clin Periodontol. 2012; 39:400–407.
15. Koban I, Holtfreter B, Hübner NO, Matthes R, Sietmann R, Kindel E, Weltmann KD, Welk A, Kramer A, Kocher T. Antimicrobial efficacy of non-thermal plasma in comparison to chlorhexidine against dental biofilms on titanium discs in vitro - proof of principle experiment. J Clin Periodontol. 2011; 38:956–965.
16. Idlibi AN, Al-Marrawi F, Hannig M, Lehmann A, Rueppell A, Schindler A, Jentsch H, Rupf S. Destruction of oral biofilms formed in situ on machined titanium (Ti) surfaces by cold atmospheric plasma. Biofouling. 2013; 29:369–379.
17. Weltmann KD, Kindel E, Brandenburg R, Meyer C, Bussiahn R, Wilke C, von Woedtke T. Atmospheric pressure plasma jet for medical therapy: Plasma parameters and risk estimation. Contrib Plasma Phys. 2009; 49:631–640.
18. Vogelsang A, Ohl A, Steffen H, Foest R, Schröder K, Weltmann KD. Locally resolved analysis of polymer surface functionalization by an atmospheric pressure argon microplasma jet with air entrainment. Plasma Process Polym. 2010; 7:16–24.
19. Choi YH, Kim JH, Paek KH, Ju WT, Hwang YS. Characteristics of atmospheric pressure N2 cold plasma torch using 60-Hz AC power and its application to polymer surface modification. Surf Coat Technol. 2005; 193:319–324.
20. Lee SB, Kim YK. Adhesion improvement of polyimide/metal interface by He/O2/NF3 atmospheric pressure plasma. Plasma Process Polym. 2009; 6:525–529.
21. Han GJ, Chung SN, Chun BH, Kim CK, Oh KH, Cho BH. Effect of the applied power of atmospheric pressure plasma on the adhesion of composite resin to dental ceramic. J Adhes Dent. 2012; 14:461–469.
22. Schneider R, de Goes MF, Henriques GE, Chan DC. Tensile bond strength of dual curing resin-based cements to commercially pure titanium. Dent Mater. 2007; 23:81–87.
23. dos Santos JG, Fonseca RG, Adabo GL, dos Santos Cruz CA. Shear bond strength of metal-ceramic repair systems. J Prosthet Dent. 2006; 96:165–173.
24. Valverde GB, Coelho PG, Janal MN, Lorenzoni FC, Carvalho RM, Thompson VP, Weltemann KD, Silva NR. Surface characterisation and bonding of Y-TZP following non-thermal plasma treatment. J Dent. 2013; 41:51–59.
25. Marchesi G, Mazzoni A, Turco G, Cadenaro M, Ferrari M, Di Lenarda R, Breschi L. Aging affects the adhesive interface of posts luted with self-adhesive cements: a 1-year study. J Adhes Dent. 2013; 15:173–180.
26. Papadopoulos T, Tsetsekou A, Eliades G. Effect of aluminium oxide sandblasting on cast commercially pure titanium surfaces. Eur J Prosthodont Restor Dent. 1999; 7:15–21.
27. Watanabe I, Kurtz KS, Kabcenell JL, Okabe T. Effect of sandblasting and silicoating on bond strength of polymerglass composite to cast titanium. J Prosthet Dent. 1999; 82:462–467.
28. Petridis H, Garefis P, Hirayama H, Kafantaris NM, Koidis PT. Bonding indirect resin composites to metal: part 2. Effect of alloy surface treatment on elemental composition of alloy and bond strength. Int J Prosthodont. 2004; 17:77–82.
29. Sarafianou A, Seimenis I, Papadopoulos T. Effectiveness of different adhesive primers on the bond strength between an indirect composite resin and a base metal alloy. J Prosthet Dent. 2008; 99:377–387.
30. Ozden N, Akaltan F, Suzer S, Akovali G. Time-related wettability characteristic of acrylic resin surfaces treated by glow discharge. J Prosthet Dent. 1999; 82:680–684.
31. Silva NR, Coelho PG, Valverde GB, Becker K, Ihrke R, Quade A, Thompson VP. Surface characterization of Ti and Y-TZP following non-thermal plasma exposure. J Biomed Mater Res B Appl Biomater. 2011; 99:199–206.
32. Nishigawa G, Maruo Y, Oka M, Oki K, Minagi S, Okamoto M. Plasma treatment increased shear bond strength between heat cured acrylic resin and self-curing acrylic resin. J Oral Rehabil. 2003; 30:1081–1084.
33. Fang Z, Yang H, Qiu Y. Surface treatment of polyethylene terephthalate films using a microsecond pulse homogeneous dielectric barrier discharges in atmospheric air. IEEE Trans Plasma Sci. 2010; 38:1615–1623.
34. Cho BH, Han GJ, Oh KH, Chung SN, Chun BH. The effect of plasma polymer coating using atmospheric-pressure glow discharge on the shear bond strength of composite resin to ceramic. J Mater Sci. 2011; 46:2755–2763.
35. Elsaka SE, Swain MV. Effect of surface treatments on adhesion of low-fusing porcelain to titanium as determined by strain energy release rate. Dent Mater. 2011; 27:1213–1220.
36. Miyakawa O, Watanabe K, Okawa S, Kanatani M, Nakano S, Kobayashi M. Surface contamination of titanium by abrading treatment. Dent Mater J. 1996; 15:11–21.
37. Lim BS, Heo SM, Lee YK, Kim CW. Shear bond strength between titanium alloys and composite resin: sandblasting versus fluoride-gel treatment. J Biomed Mater Res B Appl Biomater. 2003; 64:38–43.
38. Elsaka SE. Effect of surface pretreatments on the bonding strength and durability of self-adhesive resin cements to machined titanium. J Prosthet Dent. 2013; 109:113–120.
39. Ozcan M, Valandro LF. Bond strength of two resin cements to titanium after different surface conditioning methods. Gen Dent. 2012; 60:e6–e12.
40. ISO 10477. Dentistrypolymer-based crown and bridge materials. International Standards Organization (ISO). Geneva: Switzerland;2004.
41. Matsumura H, Yanagida H, Tanoue N, Atsuta M, Shimoe S. Shear bond strength of resin composite veneering material to gold alloy with varying metal surface preparations. J Prosthet Dent. 2001; 86:315–319.