Abstract
PURPOSE
The purpose of this study was to assess the surface characteristics and the biocompatibility of zirconium (Zr) coating on Ti-6Al-4V alloy surface by radio frequency (RF) magnetron sputtering method.
MATERIALS AND METHODS
The zirconium films were developed on Ti-6Al-4V discs using RF magnetron sputtering method. Surface profile, surface composition, surface roughness and surface energy were evaluated. Electrochemical test was performed to evaluate the corrosion behavior. Cell proliferation, alkaline phosphatase (ALP) activity and gene expression of mineralized matrix markers were measured.
RESULTS
SEM and EDS analysis showed that zirconium deposition was performed successfully on Ti-6Al-4V alloy substrate. Ti-6Al-4V group and Zr-coating group showed no significant difference in surface roughness (P>.05). Surface energy was significantly higher in Zr-coating group than in Ti-6Al-4V group (P<.05). No difference in cell morphology was observed between Ti-6Al-4V group and Zr-coating group. Cell proliferation was higher in Zr-coating group than Ti-6Al-4V group at 1, 3 and 5 days (P<.05). Zr-coating group showed higher ALP activity level than Ti-6Al-4V group (P<.05). The mRNA expressions of bone sialoprotein (BSP) and osteocalcin (OCN) on Zr-coating group increased approximately 1.2-fold and 2.1-fold respectively, compared to that of Ti-6Al-4V group.
Titanium (Ti) and Ti alloy are useful material for dental implants due to its biocompatibility and mechanical properties similar to bone.1,2 Among Ti alloys, Ti-6Al-4V alloy is considered to be physiologically inert, and to have high mechanical properties such as high strength and high wear resistance.3,4,5 However, metal ions released from Ti alloys into body fluids have been reported to be associated with implant failure.6,7,8
Coating method has been considered as one approach for preventing and reducing these problems. Liu and Mattews reported that physical vapour deposition (PVD) nitride coatings on Ti-6Al-4V alloy significantly reduced the corrosion rate and enhanced wear resistance compared to non-coated surface of Ti-6Al-4V alloy.9 Coatings of other non-toxic metals, such as tantalum (Ta), niobium (Nb) and zirconium (Zr) were also considered to prevent the metal ion release. These non-toxic metals react with oxygen at room temperature, forming a thin coating of metallic oxide surface.10 This coating plays a protective role as a barrier between the metal and host tissues.
The use of Zr as a biomaterial has increased due to its good chemical and dimensional stability.11,12,13,14 Zr has a good corrosion resistance to acid and other chemicals. Zr also has no adverse effect on living organism. It was reported that Ti-Zr alloy showed enhanced cell response.15 Recently, several researches have introduced the use of Zr or zirconium oxide (ZrO2) in surface modifications.14,16,17,18 Kurell and Dahotre showed that ZrO2 coating on Ti-6Al-4V alloy led to a phase transformation on the surface and changed the surface roughness.16 They also pointed out that such a physical and chemical transformation by ZrO2 coating could be effective for cells and tissues. ZrO2 film on titanium produced by cathodic arc deposition showed enhanced osteoblastic response.17 It was reported that ZrO2 coating enhanced dental implant osseointegration.18
Among various coating techniques including plasma spraying, electrophoretic deposition, chemical vapour deposition and sol-gel method, radio frequency (RF) magnetron sputtering produced a smooth, uniform film and did not change surface roughness.19 The thickness of titanium substrate was thinner than other methods.20,21 Also, the adhesion strength of coatings obtained by RF magnetron sputtering was 46 MPa, which is expected to be strong enough to endure a torque of 30 Ncm.
Taken together, Zr coatings on Ti-based metals is thought to increase the life expectancy of surgical implants and prosthesis due to its greater wear and corrosion resistance and its better cell response than pure titanium alloys. However, cell responses to Zr coated surface on Ti-based metals by RF magnetron sputtering have poorly been studied.
Thus, the aim of this study was to evaluate on the surface characteristics and the biocompatibility of Zr-coating on Ti-6Al-4V alloy by RF magnetron sputtering.
Ti-6Al-4V discs were fabricated (15 mm and 25 mm diameter, 1 mm thickness, provided by the school of Materials Science and Engineering, Chonnam University, Gwangju, Korea). All discs were ground with 240 grit silicon carbide papers to remove debris and deposits of surfaces, and then ultrasonically cleaned in acetone and ethanol for 10 minutes each. All discs were rinsed with deionized water between applications of each solvent.
The discs were divided into 2 groups. Group I was a non-coated machined Ti-6Al-4V surface (Ti-6Al-4V group). Group II was a Zr-coated surface by RF magnetron sputtering (Zr-coating group). Forty discs per group were used for this study.
The Zr deposition by RF magnetron sputtering was performed for 20 minutes at room temperature using L-210HS-F (Anelva Corp, Kanagawa, Japan). The power was 100 W, the base pressure was 3 × 10-4 Pa and the working gas was argon (Ar) and oxygen (O2). The inlet flow rate was 100 standard cubic centimeter per minute (sccm). During deposition, the Ar flow rate was fixed at 120 sccm and the O2 flow rate was varied from 0 to 30 sccm, which caused a change in the total pressure in the chamber in the range from 1.5 to 1.7 Pa.
The surface morphology and the surface composition was evaluated using scanning electron microscopy (SEM, S-4700, Hitachi, Tokyo, Japan) equipped with energy dispersive spectroscopy (EDS, Emax, Horiba, Kyoto, Japan). Atomic force microscopy (AFM, MNAFM-2, Digital Instruments, Santa Barbara, CA, USA) was used to evaluate surface microprofile and surface microroughness. The cross-sectional image of Zr-coated Ti-6Al-4V was obtained by SEM.
Roughness test was performed in five samples from each group, using an electronic portable surface roughness tester (Diavite DH-7, Asmeto AG, Richterswil, Switzerland). The mean surface roughness was calculated and represented as arithmetical mean roughness (Ra).
The water contact angle was analyzed using image analyzing software (Surftens QA 3.0, OEG GmbH, Frankfurt, Germany). Distilled water was dropped on the sample at room temperature. The image of the water droplet was captured at 30 seconds of the delivery using image analyzing microscope (Camscope, Sometech Inc., Seoul, Korea). The contact angle was measured for 3 samples per group.
The surface energy was calculated using the Good & Van Oss model.22
γ = γSLW + γSAB
- γ: surface energy
- γSLW: dispersive component (Lifshitz-Van der Waals interactions)
- γSAB = 2√(γS+ γS-): polar component (polar interactions, Lewis acid-base)
- γS+: Lewis acid, γS-: Lewis base
γSLW, γS+ and γS- were calculated using following equation: γL(1 + cosθ) = 2[(γSLW γLLW)1/2 + (γS+ γL-)1/2 + (γL+ gS-)]
The equation was calculated from measured contact angle (θ) and known components (Table 1) of 3 different liquids, which are known as polar (distilled water and formamide) and dispersive components (diiodomethane). The contact angle for each solvent was measured for 3 samples per group, thus the surface energy was calculated for 3 samples per group.
Potentiodynamic polarization test was performed to analyze the corrosion behavior using a potentiostat (PARSTAT 2273, Princeton applied research, Oak Ridge, TN, USA). The reference electrode was a saturated calomel electrode, a counter-electrode was a platinum wire, and a working electrode was the exposed surface of the Ti-6Al-4V and Zr-coating discs. The electrolyte was phosphate buffered saline. The samples were anodically polarized from -1 V to 2 V at a scan rate of 100 mV/min.
Discs (15 mm and 25 mm diameter) for evaluation of biologic response were placed in the bottom of culture dishes (12-well and 6-well, respectively), rinsed in 70% ethanol for 3 times, exposed to UV light for 1 hour and airdried in a laminar flow.
Cultures of mouse MC3E3-T1 cells (ATCC, Rockville, MD, USA) were grown in alpha minimum essential medium (α-MEM, Invitrogen Corp., Carlsbad, CA, USA) supplemented with 10% heat-inactivated fetal bovine serum (FBS, Invitrogen Corp., Carlsbad, CA, USA), 100 mg/mL penicillin, and 100 mg/mL streptomycin (Invitrogen Corp., Carlsbad, CA, USA) at 37℃ in humidified atmosphere of 5% CO2-95% air.
Cell attachment and cell spreading were evaluated by SEM using MC3E3-T1 cells. Cells were cultured on samples in 12-well plate at a density of 2 × 104 cells/mL with α-MEM containing 10% FBS. After incubation for 1 hour, the dishes were washed with phosphate buffered saline (PBS, Invitrogen Corp., Carlsbad, CA, USA) for three times and fixed with 2.5% glutaraldehyde (Sigma, St. Louis, MO, USA) in 100 mM cacodylate buffer (Sigma, St. Louis, MO, USA). Samples were dehydrated in ethanol (30%, 60%, 95%, and 100%, in order), immersed in hexamethyldisilazane (Sigma, St. Louis, MO, USA) for 15 minutes and airdried. Samples mounted on aluminum stubs were coated with platinum to be evaluated by SEM.
The extent of cell adhesion and spreading were classified into four stages according to Rajaraman et al.23 as follows: stage 1, rounded cells in initial contact with the surface via a few filopodia; stage 2, cells exhibiting centrifugal growth of filopodia; stage 3, cells exhibiting cytoplasmic webbing; and stage 4, fully spread cells in round or polygonal shape. The number of cells at stage 3 and 4 in 6 random fields on 3 samples per group were counted using SEM at a magnification of ×500. The mean number of cells at each stage was expressed as a percentage of the total number of cells present on the samples.
The cell proliferation was assessed by 3-(4,5-dimethylthiazol-2-yl)-2,5-diphenyltetrazolium bromide (MTT) assay (CellTiter 96 AQueous, Promega, Fitchburg, WI, USA) using MC3E3-T1 cells at day 1, 3 and 5. Cells were cultured on samples in 12-well plates at a density of 2 × 104 cells/mL with α-MEM medium. The control was cultured on tissue culture plate. The medium was changed after 24 hours. The number of viable cells can be estimated from the amount of reduced fomazan product. An enzyme-linked immunoabsorbant assay (ELISA) plate reader (VERSAmax, Molecular Devices, Sunnyvale, CA, USA) was used for the quantification of fomazan accumulation by absorbance at 490 nm. All experiments were performed for 3 times and 4 samples per group were used for each experiment.
Alkaline phosphatase (ALP) activity was determined to assess the differentiation of cells using MC3T3-E1 cells at day 7. Cells were grown in 12-well plates at a density of 2 × 104 /mL with α-MEM media containing 10% FBS, 40 µg/mL ascorbate and 20 µg/mL β-glycerol phosphate. Cultured cells were treated with 0.1% Triton (Triton X-100, Sigma, St. Louis, MO, USA) and followed by freeze-thawing. p-nitrophenol phosphate (Sigma, St. Louis, MO, USA) and 2-amino-2-methyl-1-propanol buffer (Sigma, St. Louis, MO, USA) were added to cell lysates. The mixture was incubated in the oven at 60℃ for 60 minutes. After 7 days of incubation, ALP activity was estimated by measurement of absorbance at 405 nm using a spectrophotometer (SmartSpec, BioRad, Hercules, CA, USA). All experiments were repeated 3 times and 2 samples per group were used for each experiment.
Gene expressions of bone sialoprotein (BSP), collagen type-1 (COL-1) and osteocalcin (OCN) were evaluated to study the molecular events using reverse transcription-polymerase chain reaction (RT-PCR). MC3T3-E1 cells were grown in 6-well plates at a density of 1 × 105 /mL in α-MEM media containing 10% FBS, 40 µg/mL ascorbate and 20 µg/mL β-glycerol phosphate.
First-strand cDNA was synthesized from RNA extracted at day 7 in an Amplitron II thermocycler using SuperScript II (Invitrigen, USA). PCR was carried out for 30 cycles. The primer sets (Sigma-Genosys, St. Louis, MO, USA) for the amplification of genes used in this study are listed in Table 2.
All RT-PCR products were detected by agarose gel electrophoresis. A Gel-Doc imaging system (BioRad, Hercules, CA, USA) was used for the visualization. Glyceraldehyde-3-phosphate dehydrogenase (GAPDH) was used as a reference. These experiments were repeated 2 times and 2 samples per group were used for each experiment.
Surface roughness, water contact angle, surface energy, cell proportion, cell proliferation and ALP activity were evaluated for significant differences between two groups using one-way analysis of the variance (SPSS v.22.0, IBM, Armonk, NY, USA). Statistical significance was defined as P<.05.
Fig. 1 shows AFM images and SEM images of Ti-6Al-4V and Zr-coating group. AFM (Fig. 1B) and SEM (Fig. 1B') images of Zr-coating group showed a surface with irregularities. EDS profiles showed peaks of Zr in Zr-coating group (inserted figure in Fig. 1B'). On the other hand, AFM (Fig. 1A) and SEM (Fig. 1A') images of Ti-6Al-4V group showed a machined smooth surface.
Profile roughness measurements revealed that Zr-coating group was significantly rougher than Ti-6Al-4V group (0.34 and 0.27 mm, respectively, Table 3).
In cross-sectional view of SEM (Fig. 2), the coating produced by RF magnetron sputtering was uniform in the thickness. The thickness of coated film in Zr-coated surfaces was 1.61 mm. In addition, the coating combined with the substrate without a gap.
There were significant differences in the water contact angle and the surface energy between Zr-coating group and Ti-6Al-4V group (Fig. 3). Zr-coating group showed significantly lower water contact angle and significantly higher surface energy (71.44 ± 4.40 degree and 40.13 ± 30.02 mN/m, respectively) than Ti-6Al-4V group (86.39 ± 2.40 degree and 24.06 ± 4.27 mN/m, respectively).
The polarization curves for Ti-6Al-4V group and Zr-coating group were shown in Fig. 4. Ti-6Al-4V group shows unstable state in comparison with Zr-coating group (arrow heads). After that, as the potential (V) increases, both groups maintained passive state. At high potential, breakdown of passive film occurred (arrow). The result shows that Zr-coating group has faster formation of passive film and better stability compared to control group.
Cell morphology was assessed by SEM (Fig. 5). MC3E3-T1 cells cultured on all samples spread well and present normal osteoblast-like morphology. Any difference in cell morphology was not observed between Ti-6Al-4V group and Zr-coating group, showing polygonal-shaped cells with connecting projections on the surfaces of both groups.
Fig. 6 shows the mean proportion of spreading cells of stage 3 and 4 in Ti-6Al-4V group and Zr-coating group. At 1 hour incubation, the mean proportion of spreading cells of stage 3 was significantly lower in Zr-coating group than Ti-6Al-4V group (23% and 56%, respectively, P<.05), while the mean proportion of spreading cells of stage 4 was significantly higher in Zr-coating group than Ti-6Al-4V group (77% and 23%, respectively, P<.05).
Cell proliferation was evaluated by the MTT assay (Fig. 7). Both group showed good cell viability, as shown increased number of viable cells during the culture period (P<.05). At day 1, 3 and 5, Zr-coating group showed significantly higher cell proliferation level with respect to Ti-6Al-4V group (136%, 132% and 117% compared to that of Ti-6Al-4V group, respectively, P<.05).
After 7 days of culture, the ALP activity was measured (Fig. 8). ALP activity of Zr-coating group significantly increased to 140 %, compared to that of Ti-6Al-4V group (P<.05).
Fig. 9 shows the mRNA expression of BSP, COL-I and OCN from cells cultured on Ti-6Al-4V and Zr-coating group at day 7. The genes of BSP and OCN were highly expressed in Zr-coating group than in Ti-6Al-4V group (approximately 1.2-fold and 2.1-fold, respectively), while the gene of COL-I showed similar expression in Ti-6Al-4V and Zr-coating group.
Because the biologic response of bone tissues to implant materials is mediated by implant surface characteristics, such as surface morphology and surface chemistry, surface modification has been reported to enhance the success rate of implant materials.24,25 The key physical properties of implant materials should be remained, while the surface is modified to improve the biologic response between implant and host tissues. The coating technique is well-established as one of surface modifications. Among various coating techniques, RF magnetron sputtering method was used in this study, because it has been reported to form a uniform and thin coating layer with excellent bonding strength.19 RF magnetron sputtering has been introduced for calcium phosphate coating on the titanium implant surface. However, there is no study to evaluate the effect of Zr coating by RF magnetron sputtering in implant dentistry. Thus, the present study was to assess the surface characteristics and the biocompatibility of Zr coating on Ti-6Al-4V alloy by RF magnetron sputtering methods.
Zr-coating group showed a surface with irregularities, indicating that Zr-coating changed the surface topography. The coating uniformly combined with the substrate without a gap, indicating that the deposition by RF magnetron sputtering was successfully performed.
The surface roughness of Zr-coating group was significantly higher than that of Ti-6Al-4V group (P<.05). This result would contribute to the enhanced cell response. Surface roughness can affect the adsorption of protein and the proliferation of osteoblast cells.26,27 This result can explain the enhanced cell response, such as cell proliferation and ALP activity, in this study.
Wettability and surface energy are other factors that can affect cell response. The low contact angle represents the good wettability. High surface energy promote the adhesion of proteins.28 In the present study, Zr-coating reduced the contact angle and increased the surface energy. Increased surface roughness and the alteration of surface chemical composition are related with these results.
Electrochemical test showed a uniform and stable passive layer in Zr-coating group. This result could help to resolve a concern about metal ions release of Ti alloys in body fluids.
The cell morphology on the surfaces of both groups showed no significant difference. However, there was a difference in proportions of cells at stage 3 and 4 between groups. The higher proportion of fully-spread cells on Zr coating group indicates that Zr-coated surface are more cytocompatibile, leading to higher cell proliferation level compared with that of Ti-6Al-4V group.
MTT assay showed cell proliferation increasing from day 1 to day 5 in both groups (P<.05). Zr-coating group had significantly higher proliferation than Ti-6Al-4V group at day 1, 3 and 5 (P<.05). The formation of stable passive layer might have improved biocompatibility, because the passive layer prevents the release of harmful metal ions from discs in culture media. In addition, improved initial cell attachment and cell spreading can result in increased cell proliferation.
Since ALP is associated with bone mineralization, ALP activity can be used as a biomarker for the differentiation of osteoblast.29 Zr-coating group showed 140% higher ALP levels compared to Ti-6Al-4V group. From the result of ALP activity, Zr-coating group could increase cell differentiation, thereby enhancing bone apposition on the implant surface. This result was confirmed on the molecular level by using RT-PCR.
The expressions of bone-associated markers were evaluated by semi-quantitative PCR at day 7. COL-I expression is necessary before mineralized matrix formation. In this study, mRNA level of COL-I on Ti-6Al-4V and Zr-coating group expressed well. This result indicated that Ti-6Al-4V and Zr-coating group did not inhibit mineralization of MC3T3-E1 cells. BSP and OCN are known to regulate calcification and maturation of osteoblasts.30,31,32 Zr-coating group increased the expressions of BSP and OCN. Therefore, it can be said that more differentiated phenotype of osteoblasts was cultured on Zr-coating group than on Ti-6Al-4V group.
Cell response can be affected by surface properties, such as surface topography, surface roughness, hydrophilicity, surface energy and surface chemistry.33 In the present study, Zr-coating group showed enhanced cell response, that is increased number of fully-spread cells, increased cell proliferation, increased ALP activity and increased expression of BSP mRNA and OCN RNA. These results can be explained by increased surface roughness, decreased water contact angle and increased surface energy of Zr-coating group. Modified surface topography and surface chemistry, shown in SEM and AFM images, can also contribute to enhanced cell response, because it has been reported that surface properties can affect cell attachment and proliferation.28
The limitation of this study is that the experimental time period was short for evaluating the effect of Zr-coating on the osseointegration. However, it was sufficient to assess the changes in osteoblast proliferation, differentiation, and maturation, which are important events in bone remodeling. Therefore, it can be assumed that Zr-coating might enhance bone remodeling. To clarify this, further in vivo and in vitro studies are necessary.
This study showed that Zr-coating on Ti-6Al-4V could enhance the early osteoblast responses, which are important for the subsequent cell interactions and bone healing in vivo. Enhanced cell response on Zr coating surface could make non-toxic metal coatings on Ti-6Al-4V alloy suitable for orthopedic and dental implants.
Figures and Tables
Fig. 1
AFM and SEM images of (A and A') Ti-6Al-4V and (B and B') Zr-coating group. The inserted shows Zr peaks of Zr-coating group.
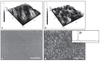
Fig. 3
(A) Water contact angle and (B) surface energy. The star (*) indicates significant difference compared with Ti-6Al-4V group.
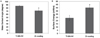
Fig. 5
Morphology of osteoblasts at 24 hour incubation on (A) Ti-6Al-4V group and (B) Zr-coating group.
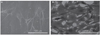
Fig. 6
Proportion of spreading cells of stage 3 and stage 4. The same letters (a, b) indicate significant differences.
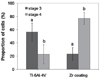
Fig. 7
Cell proliferation assay after 1, 3 and 5 days. The same letters (a, b, c) indicate significant differences.
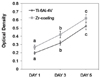
Fig. 8
Alkaline phosphatase activity after 7 days. The star (*) indicates significant difference compared with Ti-6Al-4V group.
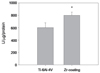
Fig. 9
Reverse transcription-polymerase chain reaction (RT-PCR) analysis of bone sialoprotein (BSP), collagen Type-I (COL-I) and osteocalcin (OCN). Glyceraldehyde-3-phosphate dehydrogenase (GAPDH) was used as a reference.
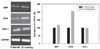
Table 1
Energy components of liquids (mN/m)
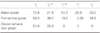
γL | γLLW | γLAB | γL+ | γL- | |
---|---|---|---|---|---|
Water (polar) | 72.8 | 21.8 | 51.0 | 25.5 | 25.5 |
Formamide (polar) | 58.0 | 39.0 | 19.0 | 2.28 | 39.6 |
Diiodomethane (non-polar) | 50.8 | 50.8 | 0 | 0 | 0 |
Table 2
Amplification primer sets used in polymerase chain reaction
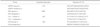
References
1. Park JB, Lakes RS. Biomaterials: an introduction. 2nd ed. New York: Plenum Press;1992. p. 89–92.
2. Oshida Y. Bioscience and bioenginerring of titanium materials. 1st ed. New York: Elsevier Press;2007. p. 11–24.
3. Khan MA, Williams RL, Williams DF. In-vitro corrosion and wear of titanium alloys in the biological environment. Biomaterials. 1996; 17:2117–2126.
4. Khan MA, Williams RL, Williams DF. Conjoint corrosion and wear in titanium alloys. Biomaterials. 1999; 20:765–772.
5. Matono Y, Nakagawa M, Matsuya S, Ishikawa K, Terada Y. Corrosion behavior of pure titanium and titanium alloys in various concentrations of Acidulated Phosphate Fluoride (APF) solutions. Dent Mater J. 2006; 25:104–112.
6. Okazaki Y, Gotoh E, Manabe T, Kobayashi K. Comparison of metal concentrations in rat tibia tissues with various metallic implants. Biomaterials. 2004; 25:5913–5920.
7. Okazaki Y, Gotoh E. Comparison of metal release from various metallic biomaterials in vitro. Biomaterials. 2005; 26:11–21.
8. Gomes CC, Moreira LM, Santos VJ, Ramos AS, Lyon JP, Soares CP, Santos FV. Assessment of the genetic risks of a metallic alloy used in medical implants. Genet Mol Biol. 2011; 34:116–121.
9. Liu C, Bi Q, Matthews A. Tribological and electrochemical performance of PVD TiN coatings on the femoral head of Ti-6Al-4V artificial hip joints. Surf Coat Technol. 2003; 163-164:597–604.
10. Arnould C, Volcke C, Lamarque C, Thiry PA, Delhalle J, Mekhalif Z. Titanium modified with layer-by-layer sol-gel tantalum oxide and an organodiphosphonic acid: a coating for hydroxyapatite growth. J Colloid Interface Sci. 2009; 336:497–503.
11. Qian MA, St John DH, Frost MT. Effect of soluble and insoluble zirconium on the grain refinement of magnesium alloys. Mater Sci Forum. 2003; 419-422:593–598.
12. Samuel S, Nag S, Nasrazadani S, Ukirde V, El Bouanani M, Mohandas A, Nguyen K, Banerjee R. Corrosion resistance and in vitro response of laser-deposited Ti-Nb-Zr-Ta alloys for orthopedic implant applications. J Biomed Mater Res A. 2010; 94:1251–1256.
13. Rosalbino F, Macciò D, Giannoni P, Quarto R, Saccone A. Study of the in vitro corrosion behavior and biocompatibility of Zr-2.5Nb and Zr-1.5Nb-1Ta (at%) crystalline alloys. J Mater Sci Mater Med. 2011; 22:1293–1302.
14. Duygulu O, Kaya AA, Oktay G, Sahin FC. Diffusion bonding of magnesium, zirconium and titanium as implant material. Mater Sci Forum. 2007; 546-549:417–420.
15. Gómez-Florit M, Xing R, Ramis JM, Taxt-Lamolle S, Haugen HJ, Lyngstadaas SP, Monjo M. Human gingival fibroblasts function is stimulated on machined hydrided titanium zirconium dental implants. J Dent. 2014; 42:30–38.
16. Kurella A, Dahotre NB. Laser induced multi-scale textured zirconia coating on Ti-6Al-4V. J Mater Sci Mater Med. 2006; 17:565–572.
17. Zhang S, Sun J, Xu Y, Qian S, Wang B, Liu F, Liu X. Adhesion, proliferation and differentiation of osteoblasts on zirconia films prepared by cathodic arc deposition. Biomed Mater Eng. 2013; 23:373–385.
18. Sollazzo V, Pezzetti F, Scarano A, Piattelli A, Bignozzi CA, Massari L, Brunelli G, Carinci F. Zirconium oxide coating improves implant osseointegration in vivo. Dent Mater. 2008; 24:357–361.
19. Yang Y, Kim KH, Ong JL. A review on calcium phosphate coatings produced using a sputtering process--an alternative to plasma spraying. Biomaterials. 2005; 26:327–337.
20. Nelea V, Morosanu C, Iliescu M, Mihilescu IN. Microstructure and mechanical properties of hydroxyapatite thin films grown by RF magnetron sputtering. Surf Coat Technol. 2003; 173:315–322.
21. Wan T, Aoki H, Hikawa J, Lee JH. RF-magnetron sputtering technique for producing hydroxyapatite coating film on various substrates. Biomed Mater Eng. 2007; 17:291–297.
22. van Oss CJ, Chaudhury MK, Good RJ. Monopolar surfaces. Adv Colloid Interface Sci. 1987; 28:35–64.
23. Rajaraman R, Rounds DE, Yen SP, Rembaum A. A scanning electron microscope study of cell adhesion and spreading in vitro. Exp Cell Res. 1974; 88:327–339.
24. Hanawa T, Sakamoto H, Tanaka Y. Biofunctional hybride of titanium with polymers. Mater Sci Forum. 2007; 539-543:563–566.
25. Kawahara H, Soeda Y, Niwa K, Takahashi M, Kawahara D, Araki N. In vitro study on bone formation and surface topography from the standpoint of biomechanics. J Mater Sci Mater Med. 2004; 15:1297–1307.
26. Lincks J, Boyan BD, Blanchard CR, Lohmann CH, Liu Y, Cochran DL, Dean DD, Schwartz Z. Response of MG63 osteoblast-like cells to titanium and titanium alloy is dependent on surface roughness and composition. Biomaterials. 1998; 19:2219–2232.
27. Kawahara H, Soeda Y, Niwa K, Takahashi M, Kawahara D, Araki N. In vitro study on bone formation and surface topography from the standpoint of biomechanics. J Mater Sci Mater Med. 2004; 15:1297–1307.
28. Pessková V, Kubies D, Hulejová H, Himmlová L. The influence of implant surface properties on cell adhesion and proliferation. J Mater Sci Mater Med. 2007; 18:465–473.
29. Gerstenfeld LC, Chipman SD, Glowacki J, Lian JB. Expression of differentiated function by mineralizing cultures of chicken osteoblasts. Dev Biol. 1987; 122:49–60.
30. Ganss B, Kim RH, Sodek J. Bone sialoprotein. Crit Rev Oral Biol Med. 1999; 10:79–98.
31. Hunter GK, Goldberg HA. Nucleation of hydroxyapatite by bone sialoprotein. Proc Natl Acad Sci U S A. 1993; 90:8562–8565.
32. Paredes R, Arriagada G, Cruzat F, Olate J, Van Wijnen A, Lian J, Stein G, Stein J, Montecino M. The Runx2 transcription factor plays a key role in the 1alpha,25-dihydroxy Vitamin D3-dependent upregulation of the rat osteocalcin (OC) gene expression in osteoblastic cells. J Steroid Biochem Mol Biol. 2004; 89-90:269–271.
33. Bacakova L, Filova E, Parizek M, Ruml T, Svorcik V. Modulation of cell adhesion, proliferation and differentiation on materials designed for body implants. Biotechnol Adv. 2011; 29:739–767.