Abstract
PURPOSE
The purpose of this study was to assess the effect of systemically administered oxytocin (OT) on the implant-bone interface by using histomorphometric analysis and the removal torque test.
MATERIALS AND METHODS
A total of 10 adult, New Zealand white, female rabbits were used in this experiment. We placed 2 implants (CSM; CSM Implant, Daegu, South Korea) in each distal femoral metaphysis on both the right and left sides; the implants on both sides were placed 10 mm apart. In each rabbit, 1 implant was prepared for histomorphometric analysis and the other 3 were prepared for the removal torque test (RT). The animals received intramuscular injections of either saline (control group; 0.15 M NaCl) or OT (experimental group; 200 µg/rabbit). The injections were initiated on Day 3 following the implant surgery and were continued for 4 subsequent weeks; the injections were administered twice per day (at a 12-h interval), for 2 days per week.
RESULTS
While no statistically significant difference was observed between the two groups (P=.787), the control group had stronger removal torque values. The serum OT concentration (ELISA value) was higher in the OT-treated group, although no statistically significant difference was found. Further, the histomorphometric parameter (bone-toimplant contact [BIC], inter-thread bone, and peri-implant bone) values were higher in the experimental group, but the differences were not significant.
CONCLUSION
We postulate that OT supplementation via intramuscular injection weakly contributes to the bone response at the implant-bone interface in rabbits. Therefore, higher concentrations or more frequent administration of OT may be required for a greater bone response to the implant. Further studies analyzing these aspects are needed.
Osseointegration is a vital process that occurs during bone healing and formation of new bone. It is defined as "a direct structural and functional connection between ordered, living bone and the surface of the load-carrying implant".1 The conventional 2-stage approach for implantation was first reported by Bränemark et al. in 1969. In the first stage, the mucosa is reflected, implants and cover screws are placed, and the surgical site is sutured. After approximately 4 to 6 months, a second surgery is performed in which the transmucosal abutments are connected. However, this conventional implant protocol proposed by Bränemark has undergone changes over time. Currently, patients whose conditions are favorable for implantation (bone volume and height) undergo the immediate loading protocol in which installation and prosthetic activation are carried out within a 48-h period.2 This treatment option has been developed in order to assuage the patient's masticatory disability and for esthetic issues during the healing period. The osseointegration rate of titanium dental implants is related to their composition and surface roughness.3 With respect to the composition, a recent advancement involves loading the surface of implants with molecules such as recombinant human bone morphogenetic protein-2 (rhBMP-2) that controls the bone remodeling process and thereby enhances the healing process.4 In addition, growth hormone (GH) is used as a growth factor to promote implant integration.5,6,7,8 In this regard, the importance of oxytocin (OT) as a potential growth factor that can be used to promote bone healing has been suggested.9,10,11
In vivo, OT has long been shown to be one of the most important regulators of uterine contraction during parturition and milk ejection throughout lactation.12 This peptide is produced in the hypothalamus, whereupon it passes to the neurohypophysis via the 100,000 nerve fibers comprising the hypothalamo-hypophyseal tract. From the posterior lobe, the hormone is released into the general blood circulation in response to electrical activity at the axon endings.12 Oxytocin has effects in addition to uterine contraction and lactation, such as social bonding, appetite regulation, sexual behavior, trust, and bone regulation.13 In bone regulation, the absence of OT or the OT receptor (Oxtr) in male or female subjects results in reduced bone formation, leading to osteoporosis. OT signal is transduced via Oxtr, which is express in variety of tissues including myoblasts, osteoclasts and osteoblasts.14 OT stimulates the formation of bone by stimulating osteoblasts to a mineralizing phenotype by upregulating BMP-2 through the Schnurri-2, Osterix and ATF-4 expression.9 OT also plays an important role in osteoclast formation by activating NF-kappaB and MAP kinase pathway directly, and by up-regulating receptor activator nuclear factor-kappaB ligand (RANK-L) indirectly.9 The positive effects of OT on rat and human osteoblast-like cells in OTand Oxtr-deficient bone defects suggest the fundamentally important role of this peptide in skeletal physiology.9,15,16 Thus, OT could also promote bonding between the implant and bone, and could be considered as a growth factor. Recently, Colli et al.11 reported that intraperitoneal (ip) injection of OT promoted bone formation and inhibited bone resorption in aged, acyclic female rats during the alveolar healing process. Therefore, OT might be considered as a growth factor in the alveolar bone healing process. We hypothesized that systemically administered OT could promote bone formation at the implant-bone interface, thereby improving implant stability during the early healing period.
The objectives of the present study were to assess whether histological differences appear in the peri-implant bone with the intramuscular injection of OT after surgery, estimate the plasma OT level, evaluate quantitatively the peri-implant bone response via morphometric analysis (such as bone-to-implant contact [BIC] area), and perform the removal torque test at the implant-bone interface (Fig. 1).
A total of 10 adult, New Zealand white, female rabbits were used in this experiment, which was approved by the local animal ethics committee at the Kyungpook National University, South Korea (IRB 2012-129). The rabbits were divided into two groups (5 rabbits in each group) and were assigned to plastic cages (1 rabbit/cage). The mean weight was 2.8 (± 0.5) kg before the surgery and 3.0 (± 0.5) kg when the animals were killed. The animals were anesthetized using intramuscular injections of ketamine (Ketar; Yuhan Corporation, Seoul, South Korea; 44 mg/kg) and xylazine (Rompun; Bayer Korea, Seoul, South Korea; 68 mg/kg). Before surgery, 1.8 mL of 2% lidocaine (Yuhan Corporation, Seoul, South Korea) was injected locally into the surgical sites. Postoperatively, each rabbit received enofloxacine (Baytril; Bayer Korea), methampyrone (Novin-50; Bayer Korea), and butaphosphan (Catosal; Bayer Korea), each at a dose of 0.3 mL per animal for 3 days.
The sequence of implant placement, oxytocin administration, and blood culture are followings. Prior to the surgery, the skin was cleaned with a mixture of iodine and 75% ethanol, and the femoral metaphysis was exposed. The skin and fascia, muscle, and periosteal flap were reflected, and the bone was denuded. Low speed rotary handpiece and saline cooling were used during all surgical procedures. An implant (3.75 × 4 mm) with a resorbable blasted media (RBM) surface was used. A 3-mm-diameter drill was used to drill a hole with 4 mm in depth in the bone. Tapping or countersink drills were not used in this study. We placed 2 implants (CSM; CSM Implant, Daegu, South Korea) in each distal femoral metaphysis on the right and left sides (Ru = right upper, Rl = right lower, Lu = left upper, Ll = left lower); the implants on the right and left sides were placed 10 mm apart. In each rabbit, one implant (Ru) was prepared for histomorphometric analysis, and the other 3 were used for the removal torque test (RT). All implants penetrated only the first cortical layer, never engaging the opposite cortical bone. After insertion, the fascia and skin were closed in separate layers by using absorbable sutures (4-0 Vicryl®; Ethicon Inc., Somerville, MA, USA). Intramuscular injections of saline (control group; 0.15 M NaCl) or OT (experimental group; 200 µg/rabbit) were administered 3 days after implant surgery for 4 subsequent weeks; the injections were administered twice per day (at a 12-h interval) for 2 days per week.11 The OT dose (Sigma-Aldrich, St. Louis, MO, USA) was determined based on the findings of a previous study.11 OT was diluted with distilled water (200 µg/mL), and 1 mL was administered intramuscularly to each rabbit (2 times/2 days/week). At 31 days after surgery, blood was collected from the ear vessel, and the animals were subsequently sacrificed using an overdose of carbon dioxide (Fig. 1).
The removal torque forces of 15 dental implants were measured, while 5 implants (Ru) were used for histological analysis in each group. After a healing period of 31 days, all 10 rabbits were sacrificed, and the removal torque test was subsequently conducted. An incision was made, the soft tissues were reflected, and the implant site was denuded. After careful removal of the overgrown bone above the screw, an MGT-12 digital torque gauge (Mark-10 Corp, New York, NY, USA) was used to measure the removal torque with a connector specifically made to connect the torque gauge and the fixture. The results were recorded by measuring the maximum torque at which fracture occurred between the implant and the bone (Fig. 2).
Serum OT was measured using an OT ELISA kit (Assay Designs/Enzo Life Sciences, Ann Arbor, MI, USA) according to the manufacturer's instructions. To explain the process briefly, 50 µL of rabbit serum was diluted in 150 µL of assay buffer and was subsequently incubated with the OT ELISA plate at 4℃ overnight. Excess reagents were washed away and the substrate was added. Various concentrations of standard OT were also incubated for the calculation. After incubation, the enzymatic reaction was stopped and the color intensity was read on a microplate reader at 405 nm. The OT concentrations (pg/mL) were calculated from the standard curve.
In Histological and histomorphometic analysis, a total of 10 implants were used for histological evaluation. The implant-tissue block was removed, fixed with 10% neutral formalin solution, and stained with Villanueva bone stain for 7 days. After dehydration in a graded ethanol series (70, 80, 95, and 100% infiltration with a mixture of isopropanol and epoxy resin 812), the sample was embedded in epoxy resin 812 and polymerized in an oven at 60℃ for 7 days. The polymerized block was cut using a hard tissue-cutting machine (Accutom-50; Struers, Copenhagen, Denmark) under constant cooling. The non-decalcified sections had an initial thickness of approximately 150 µm and were successively ground to a thickness of approximately 50 µm by using a grinding machine (Rotopol-35; Struers, Copenhagen, Denmark). The sections were then examined under a microscope (Olympus, Tokyo, Japan).
The BIC area was determined by linear measurement of direct bone contact with the implant surface. The BIC image was captured using a digital camera (Olympus, Tokyo, Japan) connected to the microscope at a magnification of 40× (Fig. 2). Using the IMT image analysis software (i-Solution; IMTechnology Inc., Daejeon, Korea), the sum of the linear bone contact with the implant surface was calculated and was expressed as a percentage of the total implant length. The inter-thread bone density was defined as the area of bone inside the threads (×100%) by using the 4 most central threads in the lateral and median sections (Fig. 3). The percentage of peri-implant bone area was defined as the area of bone that had formed after implant insertion/rectangular area (×100%) (1 mm × 1.2 mm rectangle; 2 rectangles per implant) (Fig. 3).
The Mann-Whitney U test was used to identify the difference in removal torque values between the experimental and the control groups. The paired t-test was used to evaluate the difference in the level of serum OT between the two groups. The t-test was subsequently performed to examine the differences in BIC, peri-implant bone area, and inter-thread bone density values between the control and experimental groups. The values are reported as mean ± SD. All statistical analyses were performed using PASW 18.0 for Windows (SPSS Inc., Chicago, IL, USA), with P<.05 set as the level of significance.
The measured removal torque values are summarized in Table 1. The mean value of the removal torque force for the control group was 30.04 ± 17.02 N·cm, whereas that of the experimental group was 28.06 ± 10.21 N·cm. No significant difference was observed between the two groups (P=.787). However, the control group had higher values than those of the experimental group (Fig. 4).
The measured serum OT concentration values are summarized in Table 2. The OT concentrations (pg/mL) were calculated from the standard curve. The mean value of the experimental group was 936.81 pg/mL and that of the control group was 705.89 pg/mL. No significant difference was observed between the two groups (Fig. 5).
The values of the different histomorphometric parameters of osseointegration at 31 days (4 weeks + 3 days) after implantation are shown in Table 3. The values were higher in the experimental group than in the control group, but no significant differences were found between the two groups. The BIC values were higher in the experimental group (30.33% ± 13.11%) than in the control group (20.58% ± 9.81%), and the inter-thread bone density values were also higher in the experimental group (67.04% ± 12.06%) than in the control group (54.40% ± 29.66%); however, neither of these findings were statistically significant. The periimplant bone area value was 59.36% ± 11.74% in the experimental group compared to 48.39% ± 23.93% in the control group, but this difference was also not statistically significant
Representative images of the dental implant at 31 days in both the OT-treated and the control samples, with new bone formation between all threads are shown in Figures 6A and B. The histomorphometric cross-sectional view of an implant in an OT-treated sample (Fig. 6A) corresponding to the peri-implant bone area in which it was observed shows a similar percentage of bone tissue in contact with the implant when compared with similar sections in a control sample (Fig. 6B).
A variety of substances have been used to enhance the peri-implant bone response: growth factors, morphogenetic proteins, and recently, hormones such as growth hormone.6,17,18 Regarding the potential effect of OT on bone formation, Tamma et al.9 found that deletion of OT or the Oxtr in male or female mice causes osteoporosis resulting from reduced bone formation. OT stimulates osteoblast differentiation and increases osteoblastic bone formation; thus, reductions in these processes account for the osteopenic phenotype of OT deficiency, in which no discernible alterations in osteoclast numbers are observed on histomorphometry.9 This hormone has dual effects on the osteoclast: it stimulates osteoclast formation, but inhibits bone resorption by mature osteoclasts by triggering cytosolic Ca2+ release and NO synthesis.9 Colli et al.11 reported that OT promotes bone formation and inhibits bone resorption in aged, acyclic female rats during the alveolar healing process. Their study showed that the levels of the plasma biochemical bone formation markers, alkaline phosphatase (ALP) and osteocalcin were significantly higher in the experimental group. The experimental group had the highest mean post-extraction bone formation values on histomorphometric analyses, and tartrate-resistant acid phosphatase (TRAP) levels were significantly reduced in this group, representing the anti-resorptive effect of OT.11 Another study showed that mice lacking OT and Oxtr displayed severe osteoporosis due to the defective bone formation effect, suggesting the expanding roles of OT in bone remodeling.19
The optimal dosage and the side effects of OT, however, must also be considered. Excessive dosage or long-term administration (over a period of 24 hours or longer) have been shown to result in tetanic uterine contractions, uterine rupture, postpartum hemorrhage, and water intoxication in humans, whereas little is known regarding the effects on animals. The pharmacology and the possible side effects of intravenous infusion of synthetic OT are accessible from the United States Food and Drug Association (USFDA) database. However, relatively high doses of intranasal OT in humans seem to have few side effects, indicating that OT is safe to use clinically.20 Injection of 5 to 20 IU (1 IU of OT is the equivalent of approximately 2 µg of pure peptide) is commonly used clinically to induce labor and milk ejection.21 In rats, 1000 µg/kg of OT was used to demonstrate the anti-inflammatory functions of OT, and no significant side effects were observed.21,22 Further, OT is destroyed in the gastrointestinal tract, and it has a typical half-life of about 3 to 5 minutes in the blood.23 Moreover, the injected OT does not cross the blood-brain barrier. This indicates that oral administration of OT would not be sufficient and repeated injection of OT may be required to have an effect on peripheral tissues.
Regarding another local delivery method of OT, Park et al.8 reported that the OT-loaded β-tricalcium phosphate (β-TCP) delivered directly as a bone substitute promoted bone formation via an osteoinductive mode of action; thus, this method offers the possibility of achieving predictable bone regeneration in osseous defects (such as through sinus bone graft and the guided bone regeneration [GBR] procedure).
In our study, we evaluated the bone-forming capacity of OT using the removal torque test and histomorphometric analyses in rabbits that were administered with systemic OT. Intramuscular injections of saline (control group; 0.15 M NaCl) or OT (experimental group; 200 µg/rabbit) were administered 3 days after implant surgery for 4 subsequent weeks; the injections were given twice per day (at a 12-h interval) for 2 days per week.11 The intramuscular route of administration was chosen because it is convenient for evaluating the effects of OT on the interface between the implant and bone. As a method of determining implantbone bonding, the removal torque test is a destructive technique that provides a direct reading of the stability at the implant-tissue interface. The peak torque necessary to loosen the implant from the bone bed was measured in N·cm, which is a rough estimate of the interfacial shear strength of the implant attachment. The present study showed no difference between the experimental and control groups, although the torque value was slightly higher in the control group. In the histomorphometric analysis, the histomorphometric parameter values were higher in the experimental group, although the difference did not reach statistical significance.
Several factors may explain the lack of significant differences in the removal torque test and histomorphometric analysis results. While the serum OT concentration (ELISA value) was higher in the OT-treated group, the increase was not significant, which could be attributed to the degradation of OT in the blood and serum. Further, the injected OT might not have been high or frequent enough to cross the threshold level. As mentioned earlier, the half-life of OT is typically about 3 to 5 minutes in the blood; therefore, the amount of OT that reached the implant site may have been too low to have sufficient influence on the bone at the implant-bone interface. An optimum high concentration or more frequent administration of OT, which should be safe enough for human, may be required to elicit a greater bone response to the implant and further studies are needed to elucidate these aspects. We observed several increments in the histomorphometric parameter values, suggesting that OT contributed weakly to the bone response at the implantbone interface. Therefore, further studies using other hormones or an Oxtr agonist are needed to confirm the increase in the removal torque and the implant-bone interface response.
In this study of the influence of systemically administered OT on the implant-bone interface, no significant differences in the removal torque test and histomorphometric analysis results were observed. We observed some increments in the histomorphometric parameter values. An optimum high concentration or more frequent administration of OT, which should be safe enough for human, may be required to elicit a greater bone response to the implant and further studies are needed to understand these aspects. This study is the first trial to investigate the effect of systemically administered OT on the implant-bone interface and can therefore serve as an experimental model to improve further OT-related investigations.
Figures and Tables
Fig. 1
Intramuscular injections (oxytocin or saline) were given 2 times/2 days/week and animals were sacrificed at 31 days after surgery.

Fig. 2
An MGT-12 digital torque gauge (Mark-10 Corp, New York, NY, USA) was used to measure the removal torque with a connector specifically made to connect the torque gauge and the fixture.
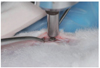
Fig. 3
The inter-thread bone density was defined as the area of bone inside the 4 most-central threads (×100%). The percentage of peri-implant bone area was defined as the area of bone that had formed after implant insertion/rectangular area (×100%) (1 mm × 1.2 mm rectangle; 2 rectangles per implant). The bone-to-implant contact (BIC) was determined by linear measurement of direct bone contact with the implant surface.
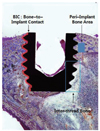
Fig. 4
Mean values of the removal torque force for the control and experimental groups. No statistically significant difference was observed between the two groups (P=.787), although the control group showed higher values.
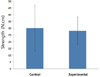
Fig. 5
Oxytocin concentrations (pg/mL) were calculated from the standard curve. The mean value for the experimental group was 936.81 pg/mL and that of the control group was 705.89 pg/mL; the values were not significantly different.
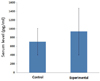
Fig. 6
Representative images at 31 days after dental implantation in an oxytocin (OT)-treated and a control sample with new bone formation between all threads. The histomorphometric cross-sectional view of an implant in an OT-treated sample (A) corresponding to the peri-implant bone area in which it was observed shows a similar percentage of bone tissue in contact with the implant when compared with similar sections in a control sample (B).
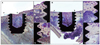
Table 1
Summary of the measured removal torque values

Table 2
Summary of the measured serum oxytocin concentration values
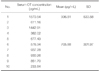
No. | Serum OT concentration (pg/mL) | Mean (pg/mL) | SD |
---|---|---|---|
1 | 1570.54 | 936.81 | 533.58 |
2 | 611.16 | ||
3 | 1442.91 | ||
4 | 382.02 | ||
5 | 677.43 | ||
6 | 576.34 | 705.88 | 301.97 |
7 | 937.28 | ||
8 | 930.26 | ||
9 | 851.70 | ||
10 | 233.84 |
Table 3
Values of the histomorphometric parameters of osseointegration at 31 days (4 weeks + 3 days) after implantation. Although the values were higher in the experimental group, no significant differences were observed between the two groups
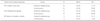
References
1. Adell R, Lekholm U, Rockler B, Brånemark PI. A 15-year study of osseointegrated implants in the treatment of the edentulous jaw. Int J Oral Surg. 1981; 10:387–416.
2. Cochran DL, Morton D, Weber HP. Consensus statements and recommended clinical procedures regarding loading protocols for endosseous dental implants. Int J Oral Maxillofac Implants. 2004; 19:109–113.
3. Le Guéhennec L, Soueidan A, Layrolle P, Amouriq Y. Surface treatments of titanium dental implants for rapid osseointegration. Dent Mater. 2007; 23:844–854.
4. Sykaras N, Woody RD, Lacopino AM, Triplett RG, Nunn ME. Osseointegration of dental implants complexed with rh-BMP-2: a comparative histomorphometric and radiographic evaluation. Int J Oral Maxillofac Implants. 2004; 19:667–678.
5. Blom EJ, Verheij JG, de Blieck-Hogervorst JM, Di Silvio L, Klein CP. Cortical bone ingrowth in growth hormone-loaded grooved implants with calcium phosphate coatings in goat femurs. Biomaterials. 1998; 19:263–270.
6. Stenport VF, Olsson B, Morberg P, Törnell J, Johansson CB. Systemically administered human growth hormone improves initial implant stability: an experimental study in the rabbit. Clin Implant Dent Relat Res. 2001; 3:135–141.
7. Tresguerres IF, Clemente C, Donado M, Gómez-Pellico L, Blanco L, Alobera MA, Tresguerres JA. Local administration of growth hormone enhances periimplant bone reaction in an osteoporotic rabbit model. Clin Oral Implants Res. 2002; 13:631–636.
8. Park JW, Kim JM, Lee HJ, Jeong SH, Suh JY, Hanawa T. Bone healing with oxytocin-loaded microporous β-TCP bone substitute in ectopic bone formation model and critical-sized osseous defect of rat. J Clin Periodontol. 2014; 41:181–190.
9. Tamma R, Colaianni G, Zhu LL, DiBenedetto A, Greco G, Montemurro G, Patano N, Strippoli M, Vergari R, Mancini L, Colucci S, Grano M, Faccio R, Liu X, Li J, Usmani S, Bachar M, Bab I, Nishimori K, Young LJ, Buettner C, Iqbal J, Sun L, Zaidi M, Zallone A. Oxytocin is an anabolic bone hormone. Proc Natl Acad Sci USA. 2009; 106:7149–7154.
10. Colaianni G, Di Benedetto A, Zhu LL, Tamma R, Li J, Greco G, Peng Y, Dell›Endice S, Zhu G, Cuscito C, Grano M, Colucci S, Iqbal J, Yuen T, Sun L, Zaidi M, Zallone A. Regulated production of the pituitary hormone oxytocin from murine and human osteoblasts. Biochem Biophys Res Commun. 2011; 411:512–515.
11. Colli VC, Okamoto R, Spritzer PM, Dornelles RC. Oxytocin promotes bone formation during the alveolar healing process in old acyclic female rats. Arch Oral Biol. 2012; 57:1290–1297.
12. Amar AP, Weiss MH. Pituitary anatomy and physiology. Neurosurg Clin N Am. 2003; 14:11–23.
13. Lee HJ, Macbeth AH, Pagani JH, Young WS 3rd. Oxytocin: the great facilitator of life. Prog Neurobiol. 2009; 88:127–151.
14. Colaianni G, Tamma R, Di Benedetto A, Yuen T, Sun L, Zaidi M, Zallone A. The oxytocin-bone axis. J Neuroendocrinol. 2014; 26:53–57.
15. Breuil V, Amri EZ, Panaia-Ferrari P, Testa J, Elabd C, Albert-Sabonnadière C, Roux CH, Ailhaud G, Dani C, Carle GF, Euller-Ziegler L. Oxytocin and bone remodelling: relationships with neuropituitary hormones, bone status and body composition. Joint Bone Spine. 2011; 78:611–615.
16. Petersson M, Lagumdzija A, Stark A, Bucht E. Oxytocin stimulates proliferation of human osteoblast-like cells. Peptides. 2002; 23:1121–1126.
17. Luo T, Zhang W, Shi B, Cheng X, Zhang Y. Enhanced bone regeneration around dental implant with bone morphogenetic protein 2 gene and vascular endothelial growth factor protein delivery. Clin Oral Implants Res. 2012; 23:467–473.
18. Gómez-Moreno G, Cutando A, Arana C, Worf CV, Guardia J, Muñoz F, Lopez-Peña M, Stephenson J. The effects of growth hormone on the initial bone formation around implants. Int J Oral Maxillofac Implants. 2009; 24:1068–1073.
19. Liu X, Shimono K, Zhu LL, Li J, Peng Y, Imam A, Iqbal J, Moonga S, Colaianni G, Su C, Lu Z, Iwamoto M, Pacifici M, Zallone A, Sun L, Zaidi M. Oxytocin deficiency impairs maternal skeletal remodeling. Biochem Biophys Res Commun. 2009; 388:161–166.
20. MacDonald E, Dadds MR, Brennan JL, Williams K, Levy F, Cauchi AJ. A review of safety, side-effects and subjective reactions to intranasal oxytocin in human research. Psychoneuroendocrinology. 2011; 36:1114–1126.
21. Işeri SO, Sener G, Saglam B, Gedik N, Ercan F, Yegen BC. Oxytocin protects against sepsis-induced multiple organ damage: role of neutrophils. J Surg Res. 2005; 126:73–81.
22. Petersson M, Lagumdzija A, Stark A, Bucht E. Oxytocin stimulates proliferation of human osteoblast-like cells. Peptides. 2002; 23:1121–1126.
23. Rydén G, Sjöholm I. Half-life of oxytocin in blood of pregnant and non-pregnant women. Acta Endocrinol (Copenh). 1969; 61:425–431.