Abstract
PURPOSE
The purpose of this study was to evaluate and compare the effects of different surface pretreatment techniques on the surface roughness and shear bond strength of a new self-adhering flowable composite resin for use with lithium disilicate-reinforced CAD/CAM ceramic material.
MATERIALS AND METHODS
A total of one hundred thirty lithium disilicate CAD/CAM ceramic plates with dimensions of 6 mm × 4 mm and 3 mm thick were prepared. Specimens were then assigned into five groups (n=26) as follows: untreated control, coating with 30 µm silica oxide particles (Cojet™ Sand), 9.6% hydrofluoric acid etching, Er:YAG laser irradiation, and grinding with a high-speed fine diamond bur. A self-adhering flowable composite resin (Vertise Flow) was applied onto the pre-treated ceramic plates using the Ultradent shear bond Teflon mold system. Surface roughness was measured by atomic force microscopy. Shear bond strength test were performed using a universal testing machine at a crosshead speed of 1 mm/min. Surface roughness data were analyzed by one-way ANOVA and the Tukey HSD tests. Shear bond strength test values were analyzed by Kruskal-Wallis and Mann-Whitney U tests at α=.05.
Because of their high esthetic properties, adhesively luted inlays and onlays, laminate veneers, and full ceramic crown restorations, which are generally fabricated using feldspathic-, leucite-, or lithium disilicate-reinforced glassy matrix ceramics, are widely used in dentistry.1 However, in spite of the desirable esthetic characteristics of these mater-ials, they are more susceptible to fracture and chipping than high-strength ceramic materials such as alumina- and/or zirconia-reinforced ceramics.1,2 It has been reported that glassy matrix ceramics present approximately three times lower fracture toughness and four times lower flexural strength than alumina- and/or zirconia-reinforced high-strength ceramics.1
Advances in dentistry have led to use of computer-aided design/computer-aided manufacturing (CAD/CAM) systems, for alternating the impression and casting procedure steps in order to produce indirect restorations faster and easier, without the need for provisional restorations and dental laboratories.3,4 Because these machinable ceramic restorations are made from highly uniform quality crystalline content as compared to laboratory-fabricated restorations, the bond strength to the hard tooth tissues and the clinical longevity of these machinable restorations have also increased.4,5,6
Because of the adhesive cementation of glassy matrix ceramic materials to the hard tooth tissues, it is difficult to remove chipped and/or fractured restorations for indirect repair. Thus, in recent years there has been a growing interest in the direct repair of ceramic restorations without removal of the entire restoration. The use of direct repair would help avoid trauma and conserve either the dental hard tissues or the restoration, thus increasing the longevity of the restorations.1,7 Currently recommended ceramic repairs in clinical procedures include the pretreatment of the chipped and/or fractured surface and the use of a repair material such as a resin composite,8,9 which is commonly used owing to its "low cost, good esthetic and easy handling" characteristics.10 To obtain an optimal and durable bond between the ceramic and the resin, various pretreatment techniques have been suggested, such as abrasion with diamond burs,11 tribochemical silica coating,12,13 sandblasting,13,14,15 airborne particle abrasion with aluminum oxide,9 chemical etching with hydrofluoric acid,10,16 laser13,14,16 treatment, and combinations of any of these techniques. Using these pretreatment techniques, the ceramic surface is roughened to create microporosities and cleaned in order to achieve sufficient activation to facilitate micromechanical and chemical bonding between the ceramic material and the resin material.3,17
The most preferred surface pretreatment technique to achieve high bond strength for silica-based all-ceramic restorations is etching with hydrofluoric acid (HF) solutions and following application of a silane coupling agent.10,14,17,18 Silane coupling agents promote adhesion and form a chemical bond with organic and inorganic surfaces, thereby increasing the wettability of the ceramic surfaces and the flow characteristics of low-viscosity resins.3,9 In addition to the HF acid treatment technique, modifications of silica-based ceramic surfaces by using a tribochemical process and laser treatment have also been investigated.13,14,16,19 Among the various recommended laser types for ceramic surface pretreatments such as carbon dioxide (CO2) and neodymium: yttrium-aluminum-garnet (Nd:YAG) lasers13,20 and tribochemical silica coatings,13,19 there are few reports on the effect of erbium: yttrium-aluminum-garnet (Er:YAG) lasers and the tribochemical process on machinable lithium disilicate-reinforced ceramic surface modification.
In addition to the ceramic surface pretreatment and chemical bonding, tight contact between the resin-based material and the ceramic surface is also an important factor for producing a durable bond.20,21 For repairing ceramic materials, resin-based luting cements, composite resins are selected because of their mechanical, physical, and esthetic properties.3,9,10,22 Recently, an adhesive-free, self-adhering, flowable composite resin, Vertise Flow (Kerr, Orange, CA, USA), was introduced into the dental market. With the simplified application procedure of this new flowable composite, it has been claimed to be indicated for the restoration of Class V cavities, small Class I cavities, lining material, pit and fissure sealing, restoration of non-carious cervical lesions, and ceramic repair.23 There are findings in the literature about in-vitro and clinical studies evaluating the performance of Vertise Flow as a restorative material. However, no studies have been found on the use of this self-adhering flowable composite as a ceramic repair material, especially with the increasingly used silicate-based CAD/CAM ceramics. Therefore, the present study aims to evaluate and compare the effects of different surface pretreatment techniques [untreated control (C), tribochemical silica coating (SC), hydrofluoric acid etching (HF), Er:YAG laser irradiation (L), diamond bur (DB) grinding] on the surface roughness and shear bond strength of this new self-adhering flowable composite resin for lithium disilicate-reinforced CAD/CAM ceramic material (IPS e. max CAD, Ivoclar-Vivadent, Schaan, Liechtenstien).
The null hypotheses are that different surface pretreatment techniques would increase the surface roughness as compared to the untreated control surfaces and that there would be no significant effect of surface pretreatment technique on the repair bond strength of the self-adhering flowable composite resin to lithium disilicate-reinforced CAD/CAM ceramics.
One hundred thirty lithium disilicate-reinforced ceramic plates with dimensions of 6 × 4 mm and 3 mm thick were prepared from CAD/CAM ceramic blocks (IPS e.max CAD; Ivoclar-Vivadent, Schaan, Liechtenstien; Lot no: R30553, Shade: HT A1/C14) using a low-speed diamond precision saw (Isomet 1000; Buehler, Lake Bluff, IL, USA). All the ceramic plates were crystallized in a ceramic furnace (P500, Ivoclar-Vivadent) in accordance with the manufacturer's recommendations. Thereafter, the ceramic plates were embedded in self-curing acrylic resin (Simplex cold cure acrylic, Associated Dental Products Ltd., Swindon, UK, Lot No.: 2221969). The repair surface of the ceramic plates remained uncovered by the acrylic resin. The repair surfaces of all the ceramic plates were then sequentially polished on a metallographic polishing device (Metaserv 250, Buehler, Lake Bluff, IL, USA) by using 400-, 600-, 800-, and 1000-grit silicon carbide paper for surface standardization under water cooling. The ceramic plates were cleaned in distilled water for 15 min in an ultrasonic bath (Ultrasonic Cleaner; Biem Ultrasonic Makina San. Ltd. Şti., Turkey) in order to remove any surface residues due to the silicon carbide papers and then air-dried. The ceramic plates were randomly assigned into five groups (n=26 for each group) depending on the surface pretreatment technique employed. One operator carried out all the procedures to avoid any operator-induced differences in the results.
Group C-untreated (control): No treatment was applied to the repair surfaces of these ceramic plates, and this group served as a control as reported by the manufacturers in the technical bulletin of the self-adhering composite resin material.
Group SC-tribochemical silica coating: The repair surfaces of the ceramic plates in this group were subjected to a silica coating process by using an intraoral air-abrasion device (RondoFlex Plus, KaVo, Biberach, Germany) filled with 30 µm silica-coated alumina particles (Cojet™ Sand, 3M ESPE, St. Paul, MN, USA, Lot No.: 380208). The silica coating was performed at 2.5 bar (250 kPa) air pressure from a distance of 10 mm for 10 seconds in circular movements. Thereafter, the specimens were thoroughly rinsed with a water spray for 30 seconds to clean the surface residual sand particles and then dried with oil-free air. The conditioned ceramic plates were then coated with a silane (ESPE-Sil; 3M ESPE, Lot No.: 47096) specifically for the Cojet system. The reaction time was 5 minutes.
Group HF-hydrofluoric acid: The repair surfaces of the ceramic plates in this group were etched with 9.6% hydrofluoric acid (Ultradent Porcelain Etch, Ultradent Dental Products, South Jordan, UT, USA, Lot No.: B62HV) for 20 seconds and the gel was rinsed off with water for 20 seconds. The samples were then dried with oil-free compressed air. Following acid application, a silane coupling agent (Ultradent Silane, Ultradent Dental Products) was applied with a mini-sponge to the repair surfaces of the ceramics for 60 seconds and allowed to air-dry for 30 seconds.
Group L-Er:YAG laser irradiation: The repair surfaces of the ceramic plates in this group were irradiated by using an Er:YAG laser (Fotona, At Fidelis, Ljubljana, Slovenia) with a wavelength of 2.940 nm, a power setting of 300 mJ, and a 20 Hz repetition rate at an average power output of 6 W.16 Laser energy was delivered to the repair surface with a 10-mm-long and 1-mm-diameter sapphire tip terminal and the beam was aligned perpendicular to the ceramic surface at a distance of 1 mm with a 20 seconds exposure period over the whole surface in a sweeping motion. During the irradiation, a custom-made apparatus was used to keep the laser perpendicular and at a constant working distance from the working surface of the ceramic plates. Thereafter, the irradiated ceramic surfaces were dried for 20 seconds with oil-free air and the silane coupling agent (Ultradent Silane) was applied as described for group HF.
Group DB-diamond bur: The repair surfaces of the ceramic plates in this group were ground by using a red-band 30-µm-grit-size round-ended cylindrical diamond bur (Komet Dental, Lemgo, Germany) in a high-speed hand piece with water irrigation for 10 seconds. Thereafter, the specimens were thoroughly rinsed with a water spray for 30 seconds to clean the surface, following by drying with oil-free air. The silane coupling agent (Ultradent Silane) was applied as described for group HF. The diamond bur was renewed after every five specimens.
For the surface microtopography characterization, ceramic plates from each group (n=10/per group) were selected and prepared for the AFM analysis. A Universal Scanning Probe Microscope (Ambios Technology, CA, USA) was used in air at room temperature for this purpose. Phase-mode imaging was performed using a silicon nitride cantilever probe with a nominal tip radius of 5-10 nm. Phase images were obtained in tapping mode, in which the cantilever was oscillated at its resonance frequency of 170 kHz at resolution of 512 × 512 pixels per image and a scanning area of 40 × 40 µm. The surface roughness of each group was quantitatively evaluated as the root mean square (RMS) roughness value (Sq) in nanometers of the height distribution in the AFM images.
For the composite resin bonding to all pretreated ceramic plates (n=80), a self-adhering, flowable composite resin (Vertise Flow, Kerr Corp, Orange, CA, USA, Lot No.: 3430381) was applied onto the ceramic surfaces according to the manufacturer's instructions as follows. A 0.5-mm-thick layer of self-adhering composite resin was condensed onto the ceramic surface by using a cylindrical Teflon mold (Ultradent Products Inc., South Jordan, UT, USA) that was 2.38 mm in diameter and 2 mm in height. The self-adhering composite resin was rubbed for 15-20 seconds with a microbrush that was provided by the manufacturer and light cured for 20 s with a quartz-tungsten-halogen light Optilux 501 (Kerr, Orange, CA, USA; output 850 mW/cm2). Then, a 1.5-mm-thick layer of composite resin was condensed and light cured for 20 seconds. The prepared specimens were removed from the mold and immersed in distilled water at 37℃ for 24 h prior to the bond strength test procedures.
The embedded ceramic plates (n=16 per group) in acrylic resin molds were placed into the universal testing machine (Autograph, Shimadzu Corp, Japan) and shear bond strength tests were performed with a crosshead speed of 1 mm/min by using a knife-edge-shaped apparatus placed between the ceramic plates and the composite resin. Shear load was applied until failure occurred and the value was recorded in Newtons (N). Thereafter, the obtained shear bond strength values were expressed in megapascals (MPa) by dividing the recorded peak load at failure (N) by the adhesive surface area (mm2).
In order to perform a qualitative surface morphology evaluation, an additional representative specimen was prepared for each group and evaluated at 10 kV in conventional mode (high-vacuum conditions) by using FE-SEM microscopy (ESEM-FEG XL-30; Phillips, Eindhoven, The Netherlands). Samples without a coating were examined using the secondary electron mode at ×1,000 magnification.
The obtained results were primarily analyzed by the Kolmogorov-Smirnov test to evaluate for normal distribution. For the surface roughness, data were normally distributed and analyzed by one-way analysis of variance (ANOVA) and the Tukey HSD test for pairwise comparisons among the groups. Because the shear bond strength test values did not follow a normal distribution, nonparametric Kruskal-Wallis and Mann-Whitney U tests were carried out for statistical analysis in order to assess any significant differences among the groups. In the statistical analyses, the significance level was established at α=.05. All statistical analyses were carried out using the Statistical Package for Social Sciences (SPSS) software, version 21 (IBM SPSS Statistics, Chicago, IL, USA).
Mean surface roughness values and standard deviations for all pretreatment groups are presented in Table 1 and graphically illustrated in Fig. 1. Among the pretreatment groups, one-way ANOVA demonstrated that groups HF (607.30 ± 6.05 nm) and DB (602.10 ± 11.41 nm) had the highest surface roughness and groups C (113.20 ± 8.88 nm) and L (110.80 ± 6.70 nm) had the lowest surface roughness. Pairwise comparisons by Tukey HSD test showed that the surface roughness values of groups HF and DB were significantly higher than those of the other groups (P<.05). However, differences between these two groups were insignificant (P>.05). Group SC (472.10 ± 15.08 nm) showed significantly higher mean surface roughness than groups C and L (P<.001), whereas it showed lower surface roughness than groups HF and DB (P<.05). There were no significant differences between groups C and L (P>.05).
The representative AFM images for the control and pretreated ceramic groups are presented in Fig. 2 (A-E). The group L and group C specimens (Fig. 2A, Fig. 2D, respectively) revealed relatively smooth surfaces and exhibited minimal irregularities, with a few valleys in the AFM images. Group DB exhibited macroirregularities on the ceramic surfaces, with deep valleys and peaks in the AFM images (Fig. 2E) as compared to groups C and L. Groups SC and HF had the most distinct surface characteristics as compared to the other groups, and they exhibited considerably more irregularities with deeper valleys and higher peaks than groups DB and L (Fig. 2B, Fig. 2C, respectively).
The mean shear bond strength values, standard deviations, statistical differences, and number of premature failures of the control and pretreated ceramic groups that were repaired with a self-adhering composite resin are listed in Table 2 and illustrated as a box-plot graph in Fig. 3. As shown in Table 2, the mean bond strength values (MPa) and standard deviations of the tested groups were Group L = 0.91 ± 1.48, Group SC = 5.36 ± 2.89, Group HF = 6.82 ± 4.41, Group C = 1.36 ± 1.86 and Group DB = 0.82 ± 0.48. Among the pretreated groups, groups HF and SC showed significantly higher bond strength values as compared to the other pretreatment groups (P<.001), suggesting a strong interaction between the HF acid-etched and silica-coated samples and the silane-applied surface. No statistically significant differences were observed among groups SC and HF (P>.05). The lowest bond strength values were measured in group DB, and differences between this group and groups L and C were also insignificant (P>.05).
Representative SEM photographs of the pretreated ceramic surfaces are presented in Fig. 4 (A-E). SEM observations of the specimens demonstrated different surface morphologies and irregularities depending on the pretreatment technique. The SEM observations revealed that the surface morphologies of the ceramic plates were consistent with the bond strength test results except for group DB. Group C did not show any surface morphology alterations (Fig. 4D). Group L also exhibited relatively the same surface morphology with group C, some microcracks and spotted areas on the surface (Fig. 4A). The group DB specimens (Fig. 4E) showed more surface irregularities on the ceramic surfaces than did groups C and L, whereas it demonstrated bond strength values similar to those of groups C and L. In the SEM images of group HF, the acidetched surfaces of the specimens exhibited a microporous structure with numerous microporosities and grooves on the surface (Fig. 4C). The specimens in group SC conditioned with 30 µm silica-coated alumina particles exhibited small irregularities on the ceramic surfaces and attached silica particles could be seen on the surface even after rinsing with water for 30 s (Fig. 4B).
Increasing esthetic demands of patients and improvements in adhesive dentistry have led to the use of an increasing number of all-ceramic restorations in dentistry, particularly silica-based ones such as crowns, inlay-onlays, and laminate veneers. Although these ceramic materials show improved esthetics, they are also brittle, such that chipping or fracture of these materials due to trauma, the parafunctional habits of the patients, and repeated chewing function stresses is frequently observed.24,25 When a fracture or chip occurs, it is important to create a high-quality and durable repair restoration on the ceramic surface by roughening and cleaning the surface in order to achieve sufficient activation to obtain micromechanical retention and chemical bonding.6,13,14,15,17,18,21 In addition, ceramic repairs for these types of chips or fractures by using composite resins intraorally may provide an easy, practical, and cost-effective solution without having to replace the entire restoration, thus saving the damaged restoration or tooth.1,7
In the present study, the effects of different surface pretreatment techniques on surface roughness and shear bond strength of a new, self-adhering, flowable composite material, Vertise Flow (Kerr Corporation), which can be used for ceramic repair to lithium disilicate-reinforced CAD/CAM ceramic as recommended by the manufacturer, was assessed. The results show that the surface pretreatment techniques used for intraoral repair tested in the present study demonstrated different surface roughness values. Therefore, the first null hypothesis of the study which stated that different surface pretreatment techniques would increase the surface roughness when compared to the untreated control surfaces was partially accepted.
When comparing the shear bond strength values after different surface pretreatments, the lowest shear bond strength value of the self-adhering, flowable composite resin to the ceramic material was measured in group DB (0.82 ± 0.48 MPa), which involved the surface pretreatment of the CAD/CAM ceramic with a 30-µm-grit-sized fine diamond bur followed by application of a silane coupling agent. The ceramic surfaces pretreated with hydrofluoric acid followed by the application of a silane coupling agent in group HF yielded the highest bond strength value (6.82 ± 4.4 MPa) and this was statistically different from groups C, DB, and L. Therefore, the second null hypothesis of the study, which stated that there would be no significant effect of surface pretreatment technique on the repair bond strength of the self-adhering, flowable composite resin to a lithium disilicate-reinforced CAD/CAM ceramic, was rejected.
The results of the study demonstrated that group HF, in which hydrofluoric acid was applied to the ceramic surfaces, resulted in the highest surface roughness and bond strength values as compared to the other pretreatment groups. When considering the effects of surface pretreatments on the roughness of the ceramic material, group HF yielded the highest surface roughness values, and AFM images of the hydrofluoric acid-applied specimens showed distinct surface characteristics as compared to the other groups, i.e., these specimens exhibited considerably more irregularities, with deeper valleys and higher peaks. In the SEM analysis of this group, a microporous structure with numerous microporosities and grooves on the surface can be seen, which corresponds to the findings from surface roughness analysis. These findings are in accordance with those of previous studies, showing similar surface characteristics.14,26,27 Additionally, the bond strength values obtained for this group are significantly different from those of groups C, L, and DB; there was no significant difference with group SC. Similar high bond strength results have also been confirmed in previous HF acid-etched glassy matrix ceramic studies.4,11,12,18 HF acid application to the ceramic surface can create numerous microporosities, undercuts, and grooves by dissolution of the glassy matrix to expose the crystalline phase, which facilitates an increase in the surface area and micromechanical bonding.4,17,18 However, due to the hazardous effect of this acid gel for both patient and operator,4,26 it must be used very carefully during the repair of ceramic surfaces. Regarding the bonding performance of this new self-adhering flowable composite resin to the ceramic surface, there were no sufficient literatures until now for comparison of the results. In a recently published study, contrary to our findings Garcia et al.28 reported that self-adhering flowable composite resin material showed 32.3 MPa bond strength to leucite-reinforced ceramic by using microshear testing method. This difference could be due to hot pressed ceramic material for repairing substrate and microshear testing method used in their study.
Ceramic surfaces pretreated with 30-µm silica-coated alumina particles in group SC demonstrated moderate surface roughness values, showing deep valleys and high peaks in the AFM images, and they exhibited small irregularities on the ceramic surfaces in the SEM pictures as compared to groups HF and DB. When the bond strength values were evaluated, this group showed significantly higher bond strength among all the pretreatment groups except for group HF, suggesting that silica-coated alumina particles can be used effectively for intraoral repair of the ceramics. In accordance with the current study findings, Melo et al.29 and Frankenberger et al.30 also reported that a composite resin applied to glassy matrix ceramic surfaces pretreated with a tribochemical silica coating (Cojet™ Sand) showed a bond strength similar to that of HF acid etching. However, in contrast to our findings, Rüttermann et al.19 showed that ceramic surfaces conditioned with a silica coating had significantly higher bond strength than HF acid etching. By surface conditioning with a silica coating, silica particles cover the ceramic surface with an "adhesively bound layer," which has a high affinity to the silane coupling agent.19,31 Because of very good integration of this silica layer onto the ceramic surface, application of a silane coupling agent enhances the bond strength.29,31
The surface roughness and repair bond strength of group L, in which the ceramic surfaces were irradiated with an Er:YAG laser, did not increase as compared to the control group. This could be because the laser irradiation did not create a rough ceramic surface to increase the micromechanical retention of the composite resin; no surface irregularities were identified by AFM and SEM. Similar to our findings, previous studies in which high Er:YAG laser outputs were used also exhibited low surface roughness values.6 Although the laser parameters used in this study were the same as those used by Gökçe et al.16, very low bond strength values were observed. This could be attributed to their use of a hot-pressed material for the production of the ceramic specimens rather than the CAD/CAM ceramic material used in the present study, resulting in differences in the physical properties of the two ceramic materials. findings from the present study is also similar to Shiu et al.32's study which reported that the bond strengths of resin material to the glassy matrix ceramic surface after Er:YAG laser irradiation had a low bond strength as compared to HF acid etching and Cojet™ Sand.
Although group DB showed the second highest surface roughness values with irregular and rough surfaces, as verified by AFM and SEM observations, the bond strength surprisingly showed no significant enhancement. This could be attributed to the fact that the 30-µm-grit-size fine diamond bur created macrosurface irregularities without undercuts and pores for micromechanical retention, and hence no strong adhesion was obtained. Saraçoğlu et al.11 also reported that although use of a high-speed diamond bur exhibited distinct surface characteristics on IPS Empress Ceramic, the bond strength of this texture was questionable. Therefore, we can assume that high roughness values for ceramic surfaces do not always mean that good bond strength will be obtained, and the geometric characteristics of the surface rather than the surface roughness should be one of the most important factors.
In the present study, after all the surface pretreatments (except for the control), a silane coupling agent was applied onto the ceramic surfaces. It has been reported that silanization of the glassy matrix ceramic surface prior to cementation or repair is an essential step for achieving chemical adhesion between the ceramic and resin material.11,16,18,33 Silane coupling agents act as adhesion promoters that bond to silica particles with hydroxyl groups on the ceramic surface.17,29,33 After a silane material is applied to the ceramic surfaces, the resin material and silane contacts. When the resin is polymerized by light activation, the methacrylate groups within the resin copolymerize with the silane, forming a bond between the composite resin and ceramic.3,34,35
Mechanical properties of composite resin as a repair material are important factor especially in stress bearing areas. Regarding the mechanical properties of novel flowable composite resins, this new self-adhering flowable composite resin has some differences in the inorganic composition. According to the Technical Bulletin of Vertise Flow, the material contains 1 µm barium glass filler, prepolymerized filler, nano-sized colloidal silica and nanosized ytterbium fluoride. All together these inorganic fillers represent 70 wt% of its total weight.36,37 In their study Czasch and Ilie36 compared the mechanical properties of self-adhering composite resin with those of four novel flowable composites and reported that self-adhering flowable composite resin presented best micromechanical properties among the tested flowable composites. Salerno et al.38 also reported that self-adhering flowable composite resin exhibited the highest modulus and hardness among the tested flowable composite resins which is presented similar stiffness to that of non-flowable composite resin tested. With regarding these favorable mechanical properties the authors of the study decided to use this self-adhering composite resin as a repair material in the study.
The functional monomer of the self-adhering, flowable composite material used in the present study is glycerol phosphate dimethacrylate (GPDM), which is also used in the three-step total-etch adhesive OptiBond FL (Kerr Corp.), which has been reported in both laboratory and clinical research to be a well-performing adhesive among the currently using adhesive systems.39,40 The acidic phosphate group of the material is capable of etching and providing chemical adhesion to the calcium ions of tooth tissue, as well as to "a variety of intaglio surfaces," including silica-based ceramics.41
With regard to the minimum clinical bond strength values of resin materials to ceramic surfaces in the literature, Thurmond et al.42 and Piwowarczyk et al.43 reported that it must not be less than 10-12 MPa for clinical service in the oral cavity. However, in the present study, the self-adhering, flowable composite resin exhibited bond strength values less than the suggested minimum for lithium disilicate-reinforced CAD/CAM ceramic materials, irrespective of the surface pretreatment used. One explanation for the obtained low bond strength values in the present study could be that the formation of siloxane bonds between the silanol and hydroxyl groups in the ceramic, a process that is accelerated by acid catalysis.44 Hence, the acidic functional components of the self-adhering composite may not provide enhanced siloxane bonds, resulting in decreased bond strength values.44,45 In addition, the relatively viscous characteristics of the flowable composite39 cannot penetrate into the irregular surfaces of the ceramic plates to create a stronger bond.
In most of the previous studies, adhesion between the composite resin and ceramic materials was investigated and in particular, shear bond strength test methodologies were most frequently used.3,11,13,14,19,26,27,30 It has been reported that shear stresses are major stresses that generated in-vivo bonding failures of restorative materials.46,47 Therefore, in the present study, the repair bond strength of a new, self-adhering, flowable composite resin for lithium disilicate-reinforced CAD/CAM ceramic material was assessed with the shear bond strength test method.
Within the limitations of this study, hydrofluoric acid etching and tribochemical silica coating seems to be the effective surface pretreatments for repairing the lithium disilicate-reinforced CAD/CAM ceramics. On the other hand, Er:YAG laser irradiation and high-speed diamond bur pretreatments tested in this study are considered inadequate for intraoral repair. The self-adhering, flowable composite resin (Vertise Flow) used as a repair composite resin exhibited very low bond strength to lithium disilicate-reinforced CAD/CAM ceramic materials, irrespective of the surface pretreatments used. However, further studies are needed in order to correlate the obtained results. Therefore, more in vitro studies are needed before drawing conclusions on the repair performance of this self-adhering flowable composite.
Figures and Tables
![]() | Fig. 1Mean surface roughness values (Sq in nm), standard deviations and significance for all pretreatment groups. Different capital letters indicate that data are statistically significant difference (P<.05). |
![]() | Fig. 2The representative Atomic Force Microscopy (AFM) images for the control and pretreated ceramic groups. (A) Er:YAG laser irradiation, (B) Tribochemical silica coating (Cojet-Sand), (C) 9.6% Hydrofluoric acid etching, (D) Untreated-control, (E) High-speed diamond bur. |
![]() | Fig. 3Box-and-whisker plots of the mean shear bond strength values of control and pretreated ceramic groups that repaired with a self-adhering composite resin. The horizontal line within box indicates median maximum and minimum values are shown by the upper and lower bars. Circles indicate outliners. |
![]() | Fig. 4The representative scanning electron microscope photographs of the control and pretreated ceramic surfaces (Magnification ×1,000). (A) Er:YAG laser irradiation, (B) Tribochemical silica coating (Cojet-Sand), (C) 9.6% Hydrofluoric acid etching, (D) Untreated-control, (E) High-speed diamond bur. |
Table 1
Mean surface roughness values (Sq in nm) and standard deviations (SD) of each pretreatment group on lithium disilicate reinforced CAD/CAM ceramic
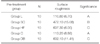
Pre-treatment group | N | Surface roughness | Significance |
---|---|---|---|
Group L | 10 | 110.80 (6.70) | A |
Group SC | 10 | 472.10 (15.08) | B |
Group HF | 10 | 607.30 (6.05) | C |
Group C | 10 | 113.20 (8.88) | A |
Group DB | 10 | 602.10 (11.41) | C |
Table 2
Mean shear bond strength values (MPa), standard deviations (SD), statistical difference and the number of the pre-test failures of the tested specimens

References
1. Ozcan M, Valandro LF, Amaral R, Leite F, Bottino MA. Bond strength durability of a resin composite on a reinforced ceramic using various repair systems. Dent Mater. 2009; 25:1477–1483.
2. Tinschert J, Natt G, Mautsch W, Augthun M, Spiekermann H. Fracture resistance of lithium disilicate-, alumina-, and zirconia-based three-unit fixed partial dentures: a laboratory study. Int J Prosthodont. 2001; 14:231–238.
3. Graiff L, Piovan C, Vigolo P, Mason PN. Shear bond strength between feldspathic CAD/CAM ceramic and human dentine for two adhesive cements. J Prosthodont. 2008; 17:294–299.
4. Peumans M, Hikita K, De Munck J, Van Landuyt K, Poitevin A, Lambrechts P, Van Meerbeek B. Effects of ceramic surface treatments on the bond strength of an adhesive luting agent to CAD-CAM ceramic. J Dent. 2007; 35:282–288.
5. Manhart J, Chen H, Hamm G, Hickel R. Buonocore Memorial Lecture. Review of the clinical survival of direct and indirect restorations in posterior teeth of the permanent dentition. Oper Dent. 2004; 29:481–508.
6. Kara HB, Dilber E, Koc O, Ozturk AN, Bulbul M. Effect of different surface treatments on roughness of IPS Empress 2 ceramic. Lasers Med Sci. 2012; 27:267–272.
7. Rathke A, Tymina Y, Haller B. Effect of different surface treatments on the composite-composite repair bond strength. Clin Oral Investig. 2009; 13:317–323.
8. Robbins JW. Intraoral repair of the fractured porcelain restoration. Oper Dent. 1998; 23:203–207.
9. Fabianelli A, Pollington S, Papacchini F, Goracci C, Cantoro A, Ferrari M, van Noort R. The effect of different surface treatments on bond strength between leucite reinforced feldspathic ceramic and composite resin. J Dent. 2010; 38:39–43.
10. Panah FG, Rezai SM, Ahmadian L. The influence of ceramic surface treatments on the micro-shear bond strength of composite resin to IPS Empress 2. J Prosthodont. 2008; 17:409–414.
11. Saraçoğlu A, Cura C, Cötert HS. Effect of various surface treatment methods on the bond strength of the heat-pressed ceramic samples. J Oral Rehabil. 2004; 31:790–797.
12. Boscato N, Della Bona A, Del Bel Cury AA. Influence of ceramic pre-treatments on tensile bond strength and mode of failure of resin bonded to ceramics. Am J Dent. 2007; 20:103–108.
13. Saraç D, Saraç YS, Külünk S, Erkoçak A. Effect of various surface treatments on the bond strength of porcelain repair. Int J Periodontics Restorative Dent. 2013; 33:e120–e126.
14. Yavuz T, Dilber E, Kara HB, Tuncdemir AR, Ozturk AN. Effects of different surface treatments on shear bond strength in two different ceramic systems. Lasers Med Sci. 2013; 28:1233–1239.
15. Kiyan VH, Saraceni CH, da Silveira BL, Aranha AC, Eduardo Cda P. The influence of internal surface treatments on tensile bond strength for two ceramic systems. Oper Dent. 2007; 32:457–465.
16. Gökçe B, Ozpinar B, Dündar M, Cömlekoglu E, Sen BH, Güngör MA. Bond strengths of all-ceramics: acid vs laser etching. Oper Dent. 2007; 32:173–178.
17. Blatz MB, Sadan A, Kern M. Resin-ceramic bonding: a review of the literature. J Prosthet Dent. 2003; 89:268–274.
18. Ozcan M, Vallittu PK. Effect of surface conditioning methods on the bond strength of luting cement to ceramics. Dent Mater. 2003; 19:725–731.
19. Rüttermann S, Fries L, Raab WH, Janda R. The effect of different bonding techniques on ceramic/ resin shear bond strength. J Adhes Dent. 2008; 10:197–203.
20. Paranhos MP, Burnett LH Jr, Magne P. Effect Of Nd:YAG laser and CO2 laser treatment on the resin bond strength to zirconia ceramic. Quintessence Int. 2011; 42:79–89.
21. Meng X, Yoshida K, Atsuta M. Microshear bond strength of resin bonding systems to machinable ceramic with different surface treatments. J Adhes Dent. 2008; 10:189–196.
22. Attar N, Tam LE, McComb D. Mechanical and physical properties of contemporary dental luting agents. J Prosthet Dent. 2003; 89:127–134.
23. Goracci C, Margvelashvili M, Giovannetti A, Vichi A, Ferrari M. Shear bond strength of orthodontic brackets bonded with a new self-adhering flowable resin composite. Clin Oral Investig. 2013; 17:609–617.
24. Ozcan M, Niedermeier W. Clinical study on the reasons for and location of failures of metal-ceramic restorations and survival of repairs. Int J Prosthodont. 2002; 15:299–302.
25. Ozcan M. Fracture reasons in ceramic-fused-to-metal restorations. J Oral Rehabil. 2003; 30:265–269.
26. Kursoglu P, Motro PF, Yurdaguven H. Shear bond strength of resin cement to an acid etched and a laser irradiated ceramic surface. J Adv Prosthodont. 2013; 5:98–103.
27. Akova T, Aytutuldu N, Yoldas O. The evaluation of different surface treatment methods for porcelain-composite bonding. Int J Adhes Adhes. 2007; 27:20–25.
28. Garcia RN, Silva CS, Silva GG, Mocellin G, Ozelame J, Fracasso L, Ozelame MB, Nascimento RF, Gomes ACR. Bonding performance of a self-adhering flowable composite to indirect restorative materials. RSBO. 2014; 11:6–12.
29. de Melo RM, Valandro LF, Bottino MA. Microtensile bond strength of a repair composite to leucite-reinforced feldspathic ceramic. Braz Dent J. 2007; 18:314–319.
30. Frankenberger R, Krämer N, Sindel J. Repair strength of etched vs silica-coated metal-ceramic and all-ceramic restorations. Oper Dent. 2000; 25:209–215.
31. Janda R, Roulet JF, Wulf M, Tiller HJ. A new adhesive technology for all-ceramics. Dent Mater. 2003; 19:567–573.
32. Shiu P, De Souza-Zaroni WC, Eduardo Cde P, Youssef MN. Effect of feldspathic ceramic surface treatments on bond strength to resin cement. Photomed Laser Surg. 2007; 25:291–296.
33. Aboushelib MN, Ghoneim M, Mirmohammadi H, Salameh Z. General principles for achieving adequate bond to all ceramic restorations. J Dent Oral Hyg. 2009; 1:36–41.
34. Shen C, Oh WS, Williams JR. Effect of post-silanization drying on the bond strength of composite to ceramic. J Prosthet Dent. 2004; 91:453–458.
35. Della Bona A, Shen C, Anusavice KJ. Work of adhesion of resin on treated lithia disilicate-based ceramic. Dent Mater. 2004; 20:338–344.
36. Czasch P, Ilie N. In vitro comparison of mechanical properties and degree of cure of a self-adhesive and four novel flowable composites. J Adhes Dent. 2013; 15:229–236.
37. Wei YJ, Silikas N, Zhang ZT, Watts DC. Hygroscopic dimensional changes of self-adhering and new resin-matrix composites during water sorption/desorption cycles. Dent Mater. 2011; 27:259–266.
38. Salerno M, Derchi G, Thorat S, Ceseracciu L, Ruffilli R, Barone AC. Surface morphology and mechanical properties of new-generation flowable resin composites for dental restoration. Dent Mater. 2011; 27:1221–1228.
39. Poitevin A, De Munck J, Van Ende A, Suyama Y, Mine A, Peumans M, Van Meerbeek B. Bonding effectiveness of selfadhesive composites to dentin and enamel. Dent Mater. 2013; 29:221–230.
40. De Munck J, Mine A, Poitevin A, Van Ende A, Cardoso MV, Van Landuyt KL, Peumans M, Van Meerbeek B. Meta-analytical review of parameters involved in dentin bonding. J Dent Res. 2012; 91:351–357.
41. Ahmad I. Compobond: Evolution of a new restorative dental material. Cosmetic Dent Eng. 2011; 2:26–37.
42. Thurmond JW, Barkmeier WW, Wilwerding TM. Effect of porcelain surface treatments on bond strengths of composite resin bonded to porcelain. J Prosthet Dent. 1994; 72:355–359.
43. Piwowarczyk A, Lauer HC, Sorensen JA. The shear bond strength between luting cements and zirconia ceramics after two pre-treatments. Oper Dent. 2005; 30:382–388.
44. Senyilmaz DP, Palin WM, Shortall AC, Burke FJ. The effect of surface preparation and luting agent on bond strength to a zirconium-based ceramic. Oper Dent. 2007; 32:623–630.
45. Aida M, Hayakawa T, Mizukawa K. Adhesion of composite to porcelain with various surface conditions. J Prosthet Dent. 1995; 73:464–470.
46. Ersu B, Yuzugullu B, Ruya Yazici A, Canay S. Surface roughness and bond strengths of glass-infiltrated alumina-ceramics prepared using various surface treatments. J Dent. 2009; 37:848–856.
47. Swift EJ Jr, Perdigão J, Heymann HO. Bonding to enamel and dentin: a brief history and state of the art, 1995. Quintessence Int. 1995; 26:95–110.