Abstract
PURPOSE
The objective of this study was to investigate the biologic effects of enamel matrix derivative (EMD) with different concentrations on cell viability and the genetic expression of human gingival fibroblasts (HGF) to zirconia surfaces.
MATERIALS AND METHODS
Immortalized human gingival fibroblasts (HGF) were cultured (1) without EMD, (2) with EMD 25 µg/mL, and (3) with EMD 100 µg/mL on zirconia discs. MTT assay was performed to evaluate the cell proliferation activity and SEM was carried out to examine the cellular morphology and attachment. The mRNA expression of collagen type I, osteopontin, fibronectin, and TGF-β1 was evaluated with the real-time polymerase chain reaction (RT-PCR).
RESULTS
From MTT assay, HGF showed more proliferation in EMD 25 µg/mL group than control and EMD 100 µg/mL group (P<.05). HGFs showed more flattened cellular morphology on the experimental groups than on the control group after 4h culture and more cellular attachments were observed on EMD 25 µg/mL group and EMD 100 µg/mL group after 24h culture. After 48h of culture, cellular attachment was similar in all groups. The mRNA expression of type I collagen increased in a concentration dependent manner. The genetic expression of osteopontin, fibronectin, and TGF-β1 was increased at EMD 100 µg/mL. However, the mRNA expression of proteins associated with cellular attachment was decreased at EMD 25 µg/mL.
Zirconia has adequate mechanical properties similar to those of stainless steel, such as high flexural and tensile strength, high resistance to abrasion and corrosion, low thermal conductivity.1,2 Survival rate and fracture load after chewing simulation of zirconia abutments showed to be similar to that of titanium abutments.3
The biocompatibility of zirconia is as comparable to that of titanium. Accumulation of bacteria on Y-TZP proved to be satisfactorily lower than titanium, which is an important characteristic of zirconia in maintaining prosthodontic restorations free of periodontal problems.4,5 Stable soft tissue attachment which has influence on the periodontal health is affected by the material composition of the implant abutments.6,7,8 To obtain the most esthetic results as in maxillary anterior implant restorations, soft tissue regeneration may be needed in cases with even zirconia abutments. In this study, we discuss positive effects of enamel matrix derivatives on soft tissue regeneration around zirconia abutments with which periodontal tissue formation can be promoted.
Enamel matrix derivative (EMD, Emdogain®, Straumann, Malmö, Sweden), is a commercially available extract of enamel matrix proteins, introduced for periodontal regeneration. EMD is predominantly composed of amelogenins and partly of peptides such as enamelin, tuftelin, ameloblastin, and proteases. Enamel matrix proteins secreted by cells of Hertwig's epithelial root sheath are deposited on the developing root surface and start the formation of acellular cementum, a process known as cementogenesis. Development of periodontal ligaments and alveolar bone follows subsequently.9,10,11,12 EMD can be employed on denuded root surfaces to stimulate regenerative responses and restore periodontal attachment successfully.13 Therefore, EMD facilitates the adhesion, proliferation, mineralization, and differentiation of osteoblasts and contribute to enhanced osteogenesis and periodontal regeneration.14,15,16,17,18,19 Practically, the use of EMD may offer positive benefits in treatment of periodontal defects and guided bone regeneration.20,21,22,23,24 Thus, beneficial outcomes of EMD on periodontal regeneration have been proven through clinical and histological data. However, effects of EMD on human gingival fibroblasts need more evaluation. Gingival fibroblasts are major cells of the gingival connective tissue and play a major role in the soft tissue sealing in the trans-mucosal part of implants. Based on the previous reports of EMD on the treatment of gingival recession and the ability to improve clinical parameters,25,26,27,28,29 we can speculate that EMD may have direct benefits on the gingival fibroblasts. Studies on EMD have reported that EMD has concentration dependent stimulatory effects on human gingival fibroblasts and the amount of matrix produced.30,31
Studies on proliferation and attachment characteristics of human gingival fibroblasts on titanium surfaces have been reported.32,33,34 However, promoted attachment of human gingival fibroblasts may be achieved on zirconia surfaces also.35,36 With the additional use of EMD, protective barrier between attached gingiva and transmucosal abutment with zirconia surface may be enhanced leading to final esthetic results with implants.
This study was designed to characterize the proliferation, attachment, and gene expression of immortalized human gingival fibroblasts on zirconia by means of MTT assay, SEM, and real-time polymerase chain reaction (RT-PCR).
The sixty zirconium discs (Ø 12 mm × 4 mm height) were sectioned from a cylinder block of zirconia (LAVA, 3M ESPE, St. Paul, MN, USA). Zirconia discs were washed twice in absolute alcohol and once in demineralised water ultrasonically, and sterilized in an autoclave.
The immortalized human gingival fibroblasts (HGF; Applied Biological Materials Inc., Richmond, BC, Canada) were grown at 37℃ in an atmosphere of 5% CO2 using Dulbecco's modified Eagle's medium (DMEM, Gibco BRL, Grand Island, NY, USA) supplemented with 10% fetal bovine serum (FBS) and 1% antibiotics. The cells with typical fibroblastic morphology from the 4-8th passage were used.
The cell proliferation of cells cultured under different concentrations of EMD on cell proliferation was examined with an MTT test assay (Sigma, St. Lois, Mo., USA). In the control group, cells were cultured with DMEM, 10% FBS, and 1% antibiotics only; in the EMD 25 group, Emdogain® (25 µg/mL) was added to the control group media; in the EMD 100 group, Emdogain® (100 µg/mL) was added to the control group media.
The substance used for the MTT test was a 3-(4,5-dimethylthiazol-2-yl)-2,5-diphenyl-tetrazolium salt, which turns into a blue formazan product due to the viable mitochondria in active cells. HGF cells were seeded at a density of 2×104 cells/ml on the discs. Cells were cultured at 37℃ in 5 % CO2 for 4, 24, and 48 hours. The discs were moved to well plates after incubation, and new media were added. Diluted MTT (5 mg/ml) solution was added, and the incubation was continued at 37℃ in 5% CO2 for 4 hours. After the incubation medium removed, 400 µL of isopropanol with 0.04 N HCl was added to each well. The resulting formazan crystal products, which reflect the level of cell metabolism, were dissolved. The absorbance of the formazan product at 490 nm was measured in triplicate with a microplate reader (Bio-kinetics reader, EL312e, Winooski, VT, USA).
The cellular adhesion and morphology of the cells were analyzed using SEM. HGF cells were seeded at a density of 2*104 cells/mL and grown for 4, 24 and 48 hours in an incubator of 37℃ in 5% CO2. The experiment wells were rinsed twice with a 0.1 M Phosphate Buffered Saline (PBS) buffer (pH 7.4) after loosely bound cells were aspirated and removed. The bound cells were fixed with 4% glutaraldehyde for 6 hours. The excess glutaraldehyde solution was removed from the cells by rinsing with PBS one more time. Dehydration in ethanol baths of progressively higher concentrations (50, 60, 70, 80, 90, 95, and 100%) for 10 min in each bath was performed. The cells were dried to a critical point and the discs were gold sputter coated with a thickness of 100 nm using an ion coater (Eiko IB-3, Eiko Engineering Ltd., Tokyo, Japan). The discs with the cultured cells were evaluated and the images were recorded by SEM (Tescan VEGA II LMU, Brno, Czech Republic) at 100× and 500× magnification.
To quantify the expression levels of collagen type I, osteopontin, fibronectin, and TGF-β1, real-time polymerase chain reaction (RT-PCR) was done. The cells were incubated for 24 hours. A thermal cycler and a laser with fiber optics were used with the 384-well plates (AB gene, Hamburg, Germany) for intensification of the fluorescent signals on this system. The fluorescence emission from each sample is collected by a charge-coupled device-camera and the quantitative data were analyzed using the ABI Prism 7900 Sequence Detection System (Applied Biosystems, San Francisco, CA, USA) and the Sequence Detection System software (SDS version 2.2, PE Applied Biosystems). Reaction regimes were composed of mixtures 10 pmol/µL of the primers, 2x SYBR Green PCR Master Mix (Applied Biosystems) with HotStarTaqt DNA-Polymerase included, the dNTP mix with dUTP added, the SYBRs Green I fluorescent dye, and ROX dye as a passive reference. To produce the relative standard curves of each gene, serial dilutions (1, 1/2, 1/4, 1/8, and 1/16) of cDNA were included in the real-time quantitative PCR plates. Glyceraldehyde-3-phoshpate dehydrogenase (GAPDH) was used as the housekeeping gene. The amplification program consisted of 50℃ for 2 min and 95℃ for 10 min followed by 40 cycles of initial denaturation at 95℃ for 30 seconds, followed by annealing at 60℃ for 30 seconds and extension at 72℃ for 30 seconds. All primers were amplified using the same conditions. The amplifications were quantified using ABI 7900HT (Applied Biosystems). Real-time PCR cycle numbers were modified to molecular size (ng) according to the equation. Experiments were repeated independently in triplicate. The name and primer sequences of the genes used are listed (Table 1).
The mean (MV) and standard deviation (SD) values of the MTT test were presented. Kruskal Wallis test was conducted for statistical evaluation of the differences between the groups. The subsequent multiple comparisons between the groups were performed using the Mann-Whitney test (P<.05).
The cell proliferation of control, EMD 25, and EMD 100 group were improved as the culture time increased. After 4 hours of cell culture, HGF proliferation of EMD 100 group decreased in comparison with the control group. After 24 hours of culture, cell proliferation of EMD 100 group increased to be comparable with the control group. After 48 hours of cell culture, cell proliferation of EMD 100 group considerably increased compared to that of the control group. Cellular viability of EMD 25 group showed to be the greatest of all groups at 4, 24, and 48 hours of cell culture. Cell proliferation was not affected by the concentration of the EMD. Cell proliferation of the control group and EMD 25 group was found to be statistically significant (Fig. 1).
After 4 hours of culture, EMD 25 group showed more cellular attachments with lamellipodia and spreading of the cellular matrix (Fig. 2). The control group and the EMD 100 µg/mL group showed stretching of the cells. Both experimental groups showed more flattening of the cells in a concentration-dependent manner compared to the control group (Fig. 3).
At 24 hours of culture, EMD 25 group showed the greatest amount of cellular attachments. However, EMD 100 group showed more flattened cells compared to the control and EMD 25 group with coherent vesicles of EMD with extracellular matrix covering the cells and the surface of the matrix (Fig. 4 and Fig. 5).
At 48 hours of culture, cells of all control and experimental groups showed higher adhesion properties. In the EMD 25 group, cellular processes showed to be longer and thinner (Fig. 6). Under higher magnification, HGF of experimental groups showed to be more flat and more cell to cell contact with each other was shown in the EMD 100 group (Fig. 7).
The amount of type I collagen expression was remarkably increased in a concentration-dependent manner and was found to be statistically significant (P<.05). Expression of osteopontin decreased with EMD 25 group. However, it was increased with significance in the EMD 100 group. Fibronectin and integrin both decreased with EMD 25 group and then increased with EMD 100 group, but did not show any significant differences. TGF-β1 showed to be decreased with EMD 25 group and then increased with EMD 100 group with statistical significance (Fig. 8).
For long-term stability of dental implants, biological seal of soft tissue and implant interface is very important. Transmucosal part of dental implant requires sufficient connective tissue attachment and inhibition of bacterial invasion and proliferation. Titanium has been used successfully as transmucosal part of dental implants. Titanium is the predominant metal used because of its biocompatibility and stability of the attachment of surrounding hard and soft tissues.37 However, titanium abutments may sometimes exhibit esthetic problems, especially in the anterior region. To improve the esthetics of dental implants, zirconia abutments with the biocompatibility and mechanical strength of titanium can be used.6,7,8,38,39,40 Previous investigations support this and thus, zirconia abutments are now used successfully in clinical use. Raffaeli suggested that zirconia is a more biocompatible material compared to feldspathic porcelain and can strongly increase the adhesion competence and the proliferation rate of fibroblasts.41 Teté proposed that zirconia showed collagen fiber orientation that was similar to that of machined titanium surface and demonstrated less plaque formation resulting in periodontal health and improved esthetics.42 Recent studies on zirconia surfaces with HGF show good attachment and cell proliferation and support the clinical significance of zirconia abutments. However, soft-tissue regeneration procedures for further esthetics and treatment of peri-implantitis may lead to need for increased attachment and growth of HGF on zirconia surfaces. Thus, enamel matrix proteins, which have been found to have positive effects on regeneration of periodontal defects, may be applied to enhance fibroblast attachment and proliferation.
Previous research has reported that enamel matrix derivative may enhance periodontal wound regeneration which has direct effect on the gingival fibroblasts and human periodontal ligament cells.25,26,27,28,29 Rincon reported that EMD of 20 µg/mL enhanced wound healing by promoting proliferation and migration of gingival and periodontal ligament fibroblast.43
In this study, the concentration of EMD was based on the study of Gestrelius et al.44 in which EMD 25 group showed the highest cellular proliferation.14,30 Our results of MTT assay of human gingival fibroblasts at 4 hours show that the concentration of EMD 25 group showed the greatest cellular viability whereas EMD 100 group showed decreased cellular viability. These in vitro results imply that high concentration may not have positive effect at short cell culture period.
Human gingival fibroblasts cultured on the samples with EMD were more spread out and evenly attached to the surface of the zirconia discs. Flattening of cytoplasm increased with higher concentration of EMD. Thus, EMD has positive influence on the cell morphology. The spreading of cytoplasm between lamellipodia showed to be remarkable in the EMD 25 group. From the SEM results, it can be speculated that lower concentration of EMD has more positive influence on initial cellular attachment.
Type I collagen exist in extracellular matrix of gingival fibroblasts and promote the attachment of gingival fibroblasts.45 In this study, expression of type I collagen in the experimental groups with EMD significantly improved in a concentration dependent manner. The results of this study support previous studies where EMD increased the number of gingival fibroblast and the amount of cellular matrix produced.30
Osteopontin is a cell adhesion protein expressed in bones which is biosynthesized by preosteoblasts, osteoblasts, and osteoclasts. However, elevated mRNA expression of osteopontin may be used to identify periodontal fibroblasts with the potential to facilitate periodontal regeneration.46 In this study, expression of osteopontin was suppressed in the EMD 25 group but increased significantly in the EMD 100 group. Thus, higher concentration of EMD increases the mRNA expression of osteopontin. Expression of osteopontin is increased with mineralization of the bone matrix. Previous studies have indicated possibilities of differentiation and mineralization of nonosteogenic cells which might have inherent roles in formation of cementum or bone.47 The results of our study comply with these findings and EMD of high concentration may stimulate osteogenic induction of human gingival fibroblasts.
Fibronectin is a protein related to cellular attachment. Normally, with increased expression of this protein, cellular attachment would increase. The gene expression results of our study showed that although cellular morphology for attachment was favorable, expression levels of fibronectin were decreased for EMD 25 group. Increased expression level of fibronectin is known to be related to rapid cell attachment.48 Therefore, at lower concentration of EMD, cell attachment may occur slowly and at higher concentration of EMD, cell attachment may occur rapidly.
TGF-β1 is a growth factor controlling cellular proliferation, differentiation, apoptosis, and integrin expression.49 In this study, mRNA expression of TGF-β1 was stimulated in the EMD 100 group. These data comply with previous studies where EMD had stimulatory effect on expression of TGF-β1 of HGF.14
EMD had stimulatory effect on type I collagen in a concentration dependent manner. This indicates the possibility of HGF cell differentiation to osteoblast-like phenotype. Overall, more cellular attachment occurred with lower concentration of EMD. However, as gene expression of proteins investigated in this study was promoted with higher concentration of EMD, the adequate concentration of EMD effective on attachment and proliferation of gingival fibroblasts on zirconia surface needs further investigation. Also, further study must be directed on whether high concentration of EMD would possibly transfer human gingival fibroblasts into cells with osteogenic potential and possibility of bone and tissue regeneration with gingival fibroblasts.
Through this short term culture of HGF on zirconium discs, we conclude that enamel matrix derivative contribute to proliferation and attachment of HGF cells. Also, enamel matrix derivative stimulates production of extracellular matrix collagen, osteopontin, and TGF-β1 in high dose. But more investigation is required to determine appropriate concentration of enamel matrix derivative for utmost cell proliferation and attachment and further investigation on differentiation potential of HGF to osteoblast-like cell would be necessary.
Figures and Tables
Fig. 1
MTT assay results of HGF over 4h, 24h and 48h. The data shows higher level of cellular viability on EMD 25 µg/mL group than control and EMD 100 µg/mL group. Significant differences between the control group and EMD 25 µg/mL group were demonstrated (*: P<.05).
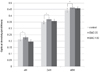
Fig. 2
SEM photomicrographs of HGF on zirconia discs at 4h (×100). (A) control group, (B) EMD 25 µg/mL group, (C) EMD 100 µg/mL group. Human gingival fibroblasts in EMD 25 µg/mL group show more cellular attachment. Spreading of cytoplasm between lamellipodia was observed (white arrow). In control and EMD 100 µg/mL group, stretched cytoplasm was observed.
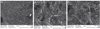
Fig. 3
SEM photomicrographs of HGF on zirconia discs at 4h (×500). (A) control group, (B) EMD 25 µg/mL group, (C) EMD 100 µg/mL group. Human gingival fibroblasts were more flattened on EMD 25 µg/mL and EMD 100 µg/mL group compared to control group after 4h culture and the width of cytoplasm increased with EMD concentration (bidirectional arrows).
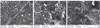
Fig. 4
SEM photomicrographs of HGF on zirconia discs at 24h (×100). (A) control group, (B) EMD 25 µg/mL group, (C) EMD 100 µg/mL group. SEM picture shows more cellular attachment observed on EMD 25 µg/mL group than control and EMD 100 µg/mL group.
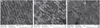
Fig. 5
SEM photomicrographs of HGF on zirconia discs at 24h (×500). (A) control group, (B) EMD 25 µg/mL group, (C) EMD 100 µg/mL group. SEM picture shows more flattened cellular morphology in EMD 100 µg/mL group than control and EMD 25 µg/mL group and aggregation of extracellular matrix and EMD vehicles in EMD 100 µg/mL group.
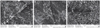
Fig. 6
SEM photomicrographs of HGF on zirconia discs at 48h (×100). (A) control group, (B) EMD 25 µg/mL group, (C) EMD 100 µg/mL group. More cellular attachments were similar in EMD groups. Cellular processes in EMD 25 µg/mL group were thin and long.
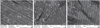
Fig. 7
SEM photomicrographs of HGF on zirconia discs at 48h (×500). (A) control group, (B) EMD 25 µg/mL group, (C) EMD 100 µg/mL group. More flattened shape of human gingival fibroblast morphology was shown in EMD groups. In EMD 100 µg/mL group, cell to cell attachment was observed.
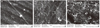
Fig. 8
mRNA expression of type I collagen, osteopontin, fibronectin, and TGF-β1. EMD increased the mRNA expression of type I collagen by human gingival fibroblasts dose dependently. The expression of osteopontin and TGF-β1 increased with significant differences in the EMD 100 µg/mL group (*: P<.05). The mRNA expression of osteopontin, fibronectin, and TGF-β1 decreased in the EMD 25 µg/mL group. The decreased amount of osteopontin and TGF-β1 was statistically significant in EMD 25 µg/mL group (*: P<.05).
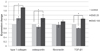
Table 1
Genes and primer sequences of the genes used

ACKNOWLEDGEMENTS
The authors would like to thank the Institut Straumann AG for providing the Emdogain® for this study.
References
1. Piconi C, Maccauro G. Zirconia as a ceramic biomaterial. Biomaterials. 1999; 20:1–25.
2. Manicone PF, Rossi Iommetti P, Raffaelli L. An overview of zirconia ceramics: basic properties and clinical applications. J Dent. 2007; 35:819–826.
3. Butz F, Heydecke G, Okutan M, Strub JR. Survival rate, fracture strength and failure mode of ceramic implant abutments after chewing simulation. J Oral Rehabil. 2005; 32:838–843.
4. Scarano A, Piattelli M, Caputi S, Favero GA, Piattelli A. Bacterial adhesion on commercially pure titanium and zirconium oxide disks: an in vivo human study. J Periodontol. 2004; 75:292–296.
5. Rimondini L, Cerroni L, Carrassi A, Torricelli P. Bacterial colonization of zirconia ceramic surfaces: an in vitro and in vivo study. Int J Oral Maxillofac Implants. 2002; 17:793–798.
6. Degidi M, Artese L, Scarano A, Perrotti V, Gehrke P, Piattelli A. Inflammatory infiltrate, microvessel density, nitric oxide synthase expression, vascular endothelial growth factor expression, and proliferative activity in peri-implant soft tissues around titanium and zirconium oxide healing caps. J Periodontol. 2006; 77:73–80.
7. Welander M, Abrahamsson I, Berglundh T. The mucosal barrier at implant abutments of different materials. Clin Oral Implants Res. 2008; 19:635–641.
8. van Brakel R, Cune MS, van Winkelhoff AJ, de Putter C, Verhoeven JW, van der Reijden W. Early bacterial colonization and soft tissue health around zirconia and titanium abutments: an in vivo study in man. Clin Oral Implants Res. 2011; 22:571–577.
9. Hammarström L. Enamel matrix, cementum development and regeneration. J Clin Periodontol. 1997; 24:658–668.
10. Hammarström L, Heijl L, Gestrelius S. Periodontal regeneration in a buccal dehiscence model in monkeys after application of enamel matrix proteins. J Clin Periodontol. 1997; 24:669–677.
11. Gestrelius S, Andersson C, Johansson AC, Persson E, Brodin A, Rydhag L, Hammarström L. Formulation of enamel matrix derivative for surface coating. Kinetics and cell colonization. J Clin Periodontol. 1997; 24:678–684.
12. Gestrelius S, Lyngstadaas SP, Hammarström L. Emdogain-periodontal regeneration based on biomimicry. Clin Oral Investig. 2000; 4:120–125.
13. Sculean A, Windisch P, Keglevich T, Fabi B, Lundgren E, Lyngstadaas PS. Presence of an enamel matrix protein derivative on human teeth following periodontal surgery. Clin Oral Investig. 2002; 6:183–187.
14. Van der Pauw MT, Van den Bos T, Everts V, Beertsen W. Enamel matrix-derived protein stimulates attachment of periodontal ligament fibroblasts and enhances alkaline phosphatase activity and transforming growth factor beta1 release of periodontal ligament and gingival fibroblasts. J Periodontol. 2000; 71:31–43.
15. Lyngstadaas SP, Lundberg E, Ekdahl H, Andersson C, Gestrelius S. Autocrine growth factors in human periodontal ligament cells cultured on enamel matrix derivative. J Clin Periodontol. 2001; 28:181–188.
16. Jiang J, Goodarzi G, He J, Li H, Safavi KE, Spångberg LS, Zhu Q. Emdogain-gel stimulates proliferation of odontoblasts and osteoblasts. Oral Surg Oral Med Oral Pathol Oral Radiol Endod. 2006; 102:698–702.
17. He J, Jiang J, Safavi KE, Spångberg LS, Zhu Q. Emdogain promotes osteoblast proliferation and differentiation and stimulates osteoprotegerin expression. Oral Surg Oral Med Oral Pathol Oral Radiol Endod. 2004; 97:239–245.
18. Lossdörfer S, Sun M, Götz W, Dard M, Jäger A. Enamel matrix derivative promotes human periodontal ligament cell differentiation and osteoprotegerin production in vitro. J Dent Res. 2007; 86:980–985.
19. Jue SS, Lee WY, Kwon YD, Kim YR, Pae A, Lee B. The effects of enamel matrix derivative on the proliferation and differentiation of human mesenchymal stem cells. Clin Oral Implants Res. 2010; 21:741–746.
20. Heden G, Wennström J, Lindhe J. Periodontal tissue alterations following Emdogain treatment of periodontal sites with angular bone defects. A series of case reports. J Clin Periodontol. 1999; 26:855–860.
21. Trombelli L, Bottega S, Zucchelli G. Supracrestal soft tissue presentation with enamel matrix proteins in the treatment of deep intrabony defects. A report of 35 consecutively treated cases. J Clin Periodontol. 2002; 29:433–439.
22. Yilmaz S, Kuru B, Altuna-Kiraç E. Enamel matrix proteins in the treatment of periodontal sites with horizontal type of bone loss. J Clin Periodontol. 2003; 30:197–206.
23. Sculean A, Chiantella GC, Arweiler NB, Becker J, Schwarz F, Stavropoulos A. Five-year clinical and histologic results following treatment of human intrabony defects with an enamel matrix derivative combined with a natural bone mineral. Int J Periodontics Restorative Dent. 2008; 28:153–161.
24. Sculean A, Kiss A, Miliauskaite A, Schwarz F, Arweiler NB, Hannig M. Ten-year results following treatment of intra-bony defects with enamel matrix proteins and guided tissue regeneration. J Clin Periodontol. 2008; 35:817–824.
25. Modica F, Del Pizzo M, Roccuzzo M, Romagnoli R. Coronally advanced flap for the treatment of buccal gingival recessions with and without enamel matrix derivative. A splitmouth study. J Periodontol. 2000; 71:1693–1698.
26. Berlucchi I, Francetti L, Del Fabbro M, Testori T, Weinstein RL. Enamel matrix proteins (Emdogain) in combination with coronally advanced flap or subepithelial connective tissue graft in the treatment of shallow gingival recessions. Int J Periodontics Restorative Dent. 2002; 22:583–593.
27. Hägewald S, Spahr A, Rompola E, Haller B, Heijl L, Bernimoulin JP. Comparative study of Emdogain and coronally advanced flap technique in the treatment of human gingival recessions. A prospective controlled clinical study. J Clin Periodontol. 2002; 29:35–41.
28. Nemcovsky CE, Artzi Z, Tal H, Kozlovsky A, Moses O. A multicenter comparative study of two root coverage procedures: coronally advanced flap with addition of enamel matrix proteins and subpedicle connective tissue graft. J Periodontol. 2004; 75:600–607.
29. Cueva MA, Boltchi FE, Hallmon WW, Nunn ME, Rivera-Hidalgo F, Rees T. A comparative study of coronally advanced flaps with and without the addition of enamel matrix derivative in the treatment of marginal tissue recession. J Periodontol. 2004; 75:949–956.
30. Keila S, Nemcovsky CE, Moses O, Artzi Z, Weinreb M. In vitro effects of enamel matrix proteins on rat bone marrow cells and gingival fibroblasts. J Dent Res. 2004; 83:134–138.
31. Zeldich E, Koren R, Nemcovsky C, Weinreb M. Enamel matrix derivative stimulates human gingival fibroblast proliferation via ERK. J Dent Res. 2007; 86:41–46.
32. Mustafa K, Silva Lopez B, Hultenby K, Wennerberg A, Arvidson K. Attachment and proliferation of human oral fibroblasts to titanium surfaces blasted with TiO2 particles. A scanning electron microscopic and histomorphometric analysis. Clin Oral Implants Res. 1998; 9:195–207.
33. Grössner-Schreiber B, Herzog M, Hedderich J, Dück A, Hannig M, Griepentrog M. Focal adhesion contact formation by fibroblasts cultured on surface-modified dental implants: an in vitro study. Clin Oral Implants Res. 2006; 17:736–745.
34. Zhang F, Huang Y, Li X, Zhao S. Surface modification and its effect on attachment, spreading, and proliferation of human gingival fibroblasts. Int J Oral Maxillofac Implants. 2011; 26:1183–1192.
35. Pae A, Lee H, Kim HS, Kwon YD, Woo YH. Attachment and growth behaviour of human gingival fibroblasts on titanium and zirconia ceramic surfaces. Biomed Mater. 2009; 4:025005.
36. Yamano S, Ma AK, Shanti RM, Kim SW, Wada K, Sukotjo C. The influence of different implant materials on human gingival fibroblast morphology, proliferation, and gene expression. Int J Oral Maxillofac Implants. 2011; 26:1247–1255.
37. Abrahamsson I, Berglundh T, Glantz PO, Lindhe J. The mucosal attachment at different abutments. An experimental study in dogs. J Clin Periodontol. 1998; 25:721–727.
38. Glauser R, Sailer I, Wohlwend A, Studer S, Schibli M, Schärer P. Experimental zirconia abutments for implant-supported single-tooth restorations in esthetically demanding regions: 4-year results of a prospective clinical study. Int J Prosthodont. 2004; 17:285–290.
39. Canullo L. Clinical outcome study of customized zirconia abutments for single-implant restorations. Int J Prosthodont. 2007; 20:489–493.
40. Zembic A, Sailer I, Jung RE, Hämmerle CH. Randomized-controlled clinical trial of customized zirconia and titanium implant abutments for single-tooth implants in canine and posterior regions: 3-year results. Clin Oral Implants Res. 2009; 20:802–808.
41. Raffaelli L, Rossi Iommetti P, Piccioni E, Toesca A, Serini S, Resci F, Missori M, De Spirito M, Manicone PF, Calviello G. Growth, viability, adhesion potential, and fibronectin expression in fibroblasts cultured on zirconia or feldspatic ceramics in vitro. J Biomed Mater Res A. 2008; 86:959–968.
42. Tetè S, Mastrangelo F, Bianchi A, Zizzari V, Scarano A. Collagen fiber orientation around machined titanium and zirconia dental implant necks: an animal study. Int J Oral Maxillofac Implants. 2009; 24:52–58.
43. Rincon JC, Haase HR, Bartold PM. Effect of Emdogain on human periodontal fibroblasts in an in vitro wound-healing model. J Periodontal Res. 2003; 38:290–295.
44. Gestrelius S, Andersson C, Lidström D, Hammarström L, Somerman M. In vitro studies on periodontal ligament cells and enamel matrix derivative. J Clin Periodontol. 1997; 24:685–692.
45. Giannopoulou C, Cimasoni G. Functional characteristics of gingival and periodontal ligament fibroblasts. J Dent Res. 1996; 75:895–902.
46. Ivanovski S, Li H, Haase HR, Bartold PM. Expression of bone associated macromolecules by gingival and periodontal ligament fibroblasts. J Periodontal Res. 2001; 36:131–141.
47. Carmona-Rodríguez B, Alvarez-Pérez MA, Narayanan AS, Zeichner-David M, Reyes-Gasga J, Molina-Guarneros J, García-Hernández AL, Suárez-Franco JL, Chavarría IG, Villarreal-Ramírez E, Arzate H. Human Cementum Protein 1 induces expression of bone and cementum proteins by human gingival fibroblasts. Biochem Biophys Res Commun. 2007; 358:763–769.
48. van der Pauw MT, Van den Bos T, Everts V, Beertsen W. Phagocytosis of fibronectin and collagens type I, III, and V by human gingival and periodontal ligament fibroblasts in vitro. J Periodontol. 2001; 72:1340–1347.
49. Heino J, Ignotz RA, Hemler ME, Crouse C, Massagué J. Regulation of cell adhesion receptors by transforming growth factor-beta. Concomitant regulation of integrins that share a common beta 1 subunit. J Biol Chem. 1989; 264:380–388.