Abstract
PURPOSE
To investigate the microtensile bond strength between two all-ceramic systems; lithium disilicate glass ceramic and zirconia core ceramics bonded with their corresponding glass veneers.
MATERIALS AND METHODS
Blocks of core ceramics (IPS e.max® Press and Lava™ Frame) were fabricated and veneered with their corresponding glass veneers. The bilayered blocks were cut into microbars; 8 mm in length and 1 mm2 in cross-sectional area (n = 30/group). Additionally, monolithic microbars of these two veneers (IPS e.max® Ceram and Lava™ Ceram; n = 30/group) were also prepared. The obtained microbars were tested in tension until fracture, and the fracture surfaces of the microbars were examined with fluorescent black light and scanning electron microscope (SEM) to identify the mode of failure. One-way ANOVA and the Dunnett's T3 test were performed to determine significant differences of the mean microtensile bond strength at a significance level of 0.05.
RESULTS
The mean microtensile bond strength of IPS e.max® Press/IPS e.max® Ceram (43.40 ± 5.51 MPa) was significantly greater than that of Lava™ Frame/Lava™ Ceram (31.71 ± 7.03 MPa)(P<.001). Fluorescent black light and SEM analysis showed that most of the tested microbars failed cohesively in the veneer layer. Furthermore, the bond strength of Lava™ Frame/Lava™ Ceram was comparable to the tensile strength of monolithic glass veneer of Lava™ Ceram, while the bond strength of bilayered IPS e.max® Press/IPS e.max® Ceram was significantly greater than tensile strength of monolithic IPS e.max® Ceram.
CONCLUSION
Because fracture site occurred mostly in the glass veneer and most failures were away from the interfacial zone, microtensile bond test may not be a suitable test for bonding integrity. Fracture mechanics approach such as fracture toughness of the interface may be more appropriate to represent the bonding quality between two materials.
All-ceramic restorations have been introduced to fixed prosthodontics according to the increasing demand for esthetic restorations. There are many types of ceramic materials being used for the fabrication of all-ceramic restorations. The higher-strength ceramics serve as substructure materials upon which more translucent glass is veneered to achieve highly esthetic prostheses.1 Several studies showed that crack or chipping of glass veneer from core ceramics was one of the most common failure modes of glass-ceramic and zirconia all-ceramic fixed dental prostheses,2,3,4,5,6 thus the bond between core and veneer might be one of the weaknesses in all-ceramic restorations and plays a significant role in their long-term success.7 There is some information available on the bond strength values between the core and veneering materials, however, no standardized bond strength test for core-veneer all-ceramic materials has been proposed.8 Various tests have been recommended for testing the core-veneer bond strength, e.g. shear bond test, three-point, four-point and biaxial flexure strength tests, tensile and microtensile bond tests, however, each test method has its own advantages and disadvantages. Shear bond test tends to develop crack in a substrate instead of along the interface due to the non-uniform stress distribution.9 For the microtensile bond strength test, it creates more uniform stress distribution, and it has been proved to be a reliable test for evaluation of bonding quality of composite materials on a variety of substrates.10 The purpose of this study was to investigate the microtensile bond strength of lithium disilicate glass-ceramic and zirconia core materials bonded with their corresponding glass veneers.
Two groups of bilayered core-veneer ceramic blocks (IPS e.max® Press/ IPS e.max® Ceram and Lava™ Frame/Lava™ Ceram) and two groups of monolithic glass veneer blocks (IPS e.max® Ceram and Lava™ Ceram) were fabricated into the dimension of 10 mm × 10 mm × 8 mm according to manufacturers' instruction (Table 1).
Bilayered blocks were serially cut into microbars (1 mm × 1 mm × 8 mm) using low-speed diamond saw (Isomet®, BUEHLER®, Lake Bluff, IL, USA) under water-cooling. Cross-sectional area of each specimen was measured using a digital vernier caliper (Model CD-15CW, Mitutoyo Corp., Kawasaki, Japan), and microbars that had cross-sectional area of 1.00 ± 0.05 mm2 were selected for testing. Adhesive (Model Repair II Blue, Dentsply-Sankin, Otahara, Japan) was used to attach the microbars to an attachment unit on both ends at approximately of 2 mm from the interface, and the microtensile bond test was carried out in tension using Bisco Microtensile Tester (Bisco Inc., Schaumburg, IL, USA). The core-veneer tested bars were observed under fluorescent black light in a darkroom and were subsequently examined with the scanning electron microscope (JSM-5410LV; JEOL Ltd., Tokyo, Japan) to locate the origin of the critical flaw. The failure modes were classified as either cohesive in glass veneer or as interfacial failure between ceramic core and glass veneer. One-way ANOVA and Dunnett's test were used to determine statistical differences among the mean microtensile strength of each group at a significant level of 0.05 using SPSS 13 (SPSS Inc., Chicago, IL, USA).
The bond strength of IPS e.max® Press/ IPS e.max® Ceram was significantly greater than that of Lava™ Frame/ Lava™ Ceram (P<.001). The core-veneer bond strength of Lava™ Frame/ Lava™ Ceram was not statistically different from the tensile strength of monolithic Lava™ Ceram, while the bond strength of bilayered IPS e.max® Press/ IPS e.max® Ceram was significantly greater than that of monolithic IPS e.max® Ceram. There was no difference in the microtensile strength between veneering materials (IPS e.max® Ceram and Lava™ Ceram)(Table 2).
After examination of fractured specimens under fluorescent black light and scanning electron microscope (SEM), it was shown that all IPS e.max® core-veneer specimens failed cohesively in the veneering material, similarly, the failure mode of zirconia Lava™ core-veneer specimens was more than 90% cohesive in the veneer (Fig. 1 and Fig. 2). In addition, for the cohesively failed specimens, fracture occurred mostly near the attachment point at the end of the free space and seldom in the center of the microbars. Fig. 3 shows the fracture surface of a Lava™ core-veneer specimen where the crack was originated from the core-veneer interface.
Core-veneer bond strength of bilayered all-ceramic restorations has become a major consideration since most of the failure was found in veneering material.2,3,4 Several testing methods have been introduced to evaluate the bond strength between core and veneering materials including microtensile bond strength test, which has been developed to overcome disadvantages of the shear and tensile bond strength tests of adhesive systems on tooth surface.11 Microtensile bond strength test provides some advantages; the applied force is more perpendicular and uniformly distributed to the tested interface; and small specimen size excludes large structural flaws, which resulted in more accurate estimation of the core-veneer bond strength.12,13,14 Nevertheless, no particular test has been proved to be the most relevant, and thus, careful attention in testing methodology helps promote the reliable outcome. This study used microtensile test with controlled quality of specimen preparation and careful attachment of the specimen on the testing device, as a result the bond strength values were comparable to previous studies with less scatter of the data.
Depending on esthetic or functional purposes, the most widely used all-ceramic systems are lithium silicate glass-ceramic and zirconia-based ceramics. Microtensile bond strength of bilayered zirconia from different studies ranged between 28-39 MPa.15,16,17 Aboushelib et al.12 reported microtensile bond strength of Lava™ zirconia framework that was veneered with veneering porcelain from different manufacturers. The bond strength value was in the range of 23-29 MPa, and it was shown that the failure was 70% interfacial. In the present study, the microtensile bond strength of Lava™ zirconia veneered with its corresponding glass veneer was 31.71 ± 7.03 MPa, and the failure pattern was rarely interfacial (<10%). The microtensile bond strength of veneered IPS e.max® Press in the present study was 43.40 ± 5.51 MPa, and the failure pattern was totally cohesive in the veneer.
Since most of the fracture occurred in the veneer, therefore these strength values should be counted as the strength of the veneering material and the actual core-veneer bond strength may be purported to be greater than the observed values. However, this may be misleading because the probability of finding the critical flaw in glass veneer is greater than that of interfacial zone due to the larger volume of glass veneer compared with interfacial zone.
Supporting the estimation, the microtensile strength of the veneering material itself was tested. IPS e.max® Ceram and Lava™ Ceram blocks were made, cut into microbars and tested in tension until fracture. The mean microtensile strength of IPS e.max® Ceram and Lava™ Ceram were 32.80 ± 4.01 MPa and 32.87 ± 4.83 MPa respectively, and they were not significantly different. The mean microtensile strength of monolithic Lava™ Ceram was comparable to that of the bilayered core-veneer Lava™ Frame/ Lava™ Ceram, of which the fracture occurred mostly in the veneer. However, the mean microtensile strength of monolithic IPS e.max® Ceram was significantly lower than the microtensile bond strength of IPS e.max® Press/ IPS e. max® Ceram, of which the fracture pattern was found to be entirely cohesive in the veneering material. This may imply that the core-veneer bond strength of IPS e.max® Press/IPS e.max® Ceram was stronger than the strength of monolithic glass veneer (IPS e.max® Ceram). In previous study, Aboushelib et al.15 reported likewise that the microtensile bond strength of veneered lithium disilicate core material was significantly higher than the microtensile strength of the veneer itself. The remarkable increase in strength of the bilayered over monolithic glass veneer was explained by some rationales, including dissimilarity of thermal expansion coefficient between veneer and core materials, and the difference in elastic behaviors of the two materials. These lead to generation of global residual compressive stress in the veneer layer.18,19 Although porcelains designed for application to ceramic frameworks may have appropriate coefficient of thermal expansion (CTE), considerable internal stresses can still develop.20 CTE of IPS e.max® Press core material is 10.15 × 10-6·K-1 and that of IPS e.max® Ceram veneering material is 9.5 × 10-6·K-1 giving the CTE difference of 0.65 × 10-6·K-1, which is in the acceptable range of -0.61 to +1.02 × 10-6·K-1.20 Difference in viscoelastic structural relaxation of IPS e.max® Ceram veneer and IPS e.max® Press core, together with the CTE difference, resulted in presence of compressive residual stress in IPS e.max® Ceram veneer of bilayered specimens during the cooling process. This compressive residual stress, which occurred in longitudinal direction parallel to the bonded interface, may be helpful for resisting the crack propagation. This may lead to the increase of microtensile strength of bilayered IPS e.max® Press/ IPS e.max® Ceram core-veneer specimens. In our study, the bond strength of this bilayered group was retested to ascertain the outcome, however, the mean value was comparable to the result reported in this study.
The present study showed significant lower microtensile bond strength of veneered Lava™ Frame than that of veneered IPS e.max® Press. Clinical performance could be estimated from in vitro evaluation that failure of veneering porcelain or minor chipping might occur more often in veneered zirconia restorations than in veneered lithium disilicate glass-ceramic restorations. In agreement, it was found that the annual rate of minor chipping of the veneering ceramic in zirconia based prosthesis was reported in the range of 1.98-12.2 per 100 fixed partial dentures years, whereas that of the glass-ceramic was found to be lower within the range of 0.83-1.55 per 100 fixed partial dentures years.5
The fracture site of microtensile specimens was expected to be at the interface, which was recognized as one of the weakest parts of the core-veneer all-ceramic system. However, fracture occurred mostly in the veneer closed to the core-veneer interface that was in agreement with previous studies.15,16,21 These might be due to greater probability of finding the critical flaw in glass veneer than that of interfacial zone because of the larger volume of glass veneer compared with interfacial zone. Besides, the veneer fracture could be explained by non-homogeneous stress distribution throughout the bilayered bar-shaped specimens. Finite element analysis of previous studies demonstrated that in microtensile test, forces passed through the bulk of material before reaching the interface and stresses mostly concentrated at the corner of the microbars. Stress was found in the lowest magnitude at the bonded interface, therefore interfacial failure was uncommonly occurred.13,22 Moreover, stress concentration was found to be localized at approximately 0.2 mm from the attached site, which resulted in fracture in the veneer at the fixed site closed to the bonded interface.10
Several factors involve in mechanical failure of bilayered all-ceramic restorations, particularly glass veneer fracture, such as restoration's geometry and fabrication processes as well as the bond between core and veneer materials.22,23 Moreover, core-veneer interface can be influenced by surface roughness of the substructure; residual stresses generated by the difference in coefficient of thermal expansion/contraction between core and veneer; presence of defects; wetting properties; bond strength values; cooling rate effects; and elastic and viscoelastic properties.22 Thus, evaluation of bond strength value alone would provide limited information, unless other related factors are considered in the field of study.
Microtensile bond strength testing method is one of the practical and popular methods to study bond strength of biomaterial interface, however, the obtained stress may be meaningless to represent the actual bond strength value when failures do not occur at or originate from the interfacial zone. Fracture mechanics approach may be more appropriate for analyzing the bonding quality between two materials.24 Some studies applied this concept to ensure that most the cracks initiated and propagated through the bonded interface in stable manner using chevron notch or modified chevron notch design in dentin bonding and bilayered ceramic.25,26,27,28
Although bond strength test value might not be highly accurate representative of the clinical outcome, in vitro microtensile bond strength test could be used as a qualitative screening test due to its convenient procedure. Defined qualitative result should be obtained by other tests using fracture mechanics approach.29,30 Although the in vitro model cannot be directly related to clinical situation, however, it could be assumed from the bond strength results of the present study that cohesive failure of the veneering porcelain is a common failure mode for all-ceramic prostheses, moreover, veneered zirconia restorations tend to have higher porcelain-veneer failure rate than veneered lithium disilicate glass-ceramic restorations.
From the result of this study, most specimens failed cohesively in the glass veneer, and such failure was not originated from the interfacial zone, therefore microtensile bond strength test may not be a good representative of bonding quality. There should be further studies that focus on fracture mechanics approach such as fracture toughness of the interface. Those tests may be more appropriate than traditional bond strength tests to represent the bonding quality between two materials.
Figures and Tables
Fig. 1
Schematics of fractured microbar of IPS e.max® Press/ IPS e.max® Ceram under fluorescent black light shows difference in appearance between the core and veneering materials. The veneer appears brighter (right), whereas the core is darker. The failure pattern is classified as cohesive failure.
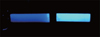
Fig. 2
Schematics of fractured microbar of Lava™ Frame/ Lava™ Ceram under fluorescent black light shows similar appearance as IPS e.max® microbars. Zirconia cores appear darker (left), and failure modes are classified as (A) cohesive failure (B) interfacial failure.

Fig. 3
SEM micrographs of fracture surface of Lava™ Frame/ Lava™ Ceram. The fracture pattern is classified as interfacial, which was found less than 10% of the tested specimens as the fracture originated in the zirconia core material at the interfacial zone. (A) the surface of veneer with exposed core material (arrows) at core side (at 85x magnification), (B) veneer side (at 90x magnification).
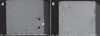
Table 1
Materials information according to manufacturer's instruction
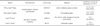
Table 2
Mean microtensile strength (MPa), standard deviation and the mode of failure

References
1. Raigrodski AJ. Contemporary materials and technologies for all-ceramic fixed partial dentures: a review of the literature. J Prosthet Dent. 2004; 92:557–562.
2. Conrad HJ, Seong WJ, Pesun IJ. Current ceramic materials and systems with clinical recommendations: a systematic review. J Prosthet Dent. 2007; 98:389–404.
3. Odman P, Andersson B. Procera AllCeram crowns followed for 5 to 10.5 years: a prospective clinical study. Int J Prosthodont. 2001; 14:504–509.
4. Raigrodski AJ, Chiche GJ, Potiket N, Hochstedler JL, Mohamed SE, Billiot S, Mercante DE. The efficacy of posterior three-unit zirconium-oxide-based ceramic fixed partial dental prostheses: a prospective clinical pilot study. J Prosthet Dent. 2006; 96:237–244.
5. Sailer I, Pjetursson BE, Zwahlen M, Hämmerle CH. A systematic review of the survival and complication rates of all-ceramic and metal-ceramic reconstructions after an observation period of at least 3 years. Part II: Fixed dental prostheses. Clin Oral Implants Res. 2007; 18:86–96.
6. Vult von Steyern P, Carlson P, Nilner K. All-ceramic fixed partial dentures designed according to the DC-Zirkon technique. A 2-year clinical study. J Oral Rehabil. 2005; 32:180–187.
7. Guazzato M, Proos K, Sara G, Swain MV. Strength, reliability, and mode of fracture of bilayered porcelain/core ceramics. Int J Prosthodont. 2004; 17:142–149.
8. Dündar M, Ozcan M, Gökçe B, Cömlekoğlu E, Leite F, Valandro LF. Comparison of two bond strength testing methodologies for bilayered all-ceramics. Dent Mater. 2007; 23:630–636.
9. Della Bona A, van Noort R. Shear vs. tensile bond strength of resin composite bonded to ceramic. J Dent Res. 1995; 74:1591–1596.
10. El Zohairy AA, de Gee AJ, de Jager N, van Ruijven LJ, Feilzer AJ. The influence of specimen attachment and dimension on microtensile strength. J Dent Res. 2004; 83:420–424.
11. Sano H, Shono T, Sonoda H, Takatsu T, Ciucchi B, Carvalho R, Pashley DH. Relationship between surface area for adhesion and tensile bond strength-evaluation of a micro-tensile bond test. Dent Mater. 1994; 10:236–240.
12. Aboushelib MN, Kleverlaan CJ, Feilzer AJ. Effect of zirconia type on its bond strength with different veneer ceramics. J Prosthodont. 2008; 17:401–408.
13. El Zohairy AA, Saber MH, Abdalla AI, Feilzer AJ. Efficacy of microtensile versus microshear bond testing for evaluation of bond strength of dental adhesive systems to enamel. Dent Mater. 2010; 26:848–854.
14. Ferrari M, Goracci C, Sadek F, Eduardo P, Cardoso C. Microtensile bond strength tests: scanning electron microscopy evaluation of sample integrity before testing. Eur J Oral Sci. 2002; 110:385–391.
15. Aboushelib MN, de Jager N, Kleverlaan CJ, Feilzer AJ. Microtensile bond strength of different components of core veneered all-ceramic restorations. Dent Mater. 2005; 21:984–991.
16. Aboushelib MN, de Kler M, van der Zel JM, Feilzer AJ. Microtensile bond strength and impact energy of fracture of CAD-veneered zirconia restorations. J Prosthodont. 2009; 18:211–216.
17. Aboushelib MN, Kleverlaan CJ, Feilzer AJ. Microtensile bond strength of different components of core veneered all-ceramic restorations. Part II: Zirconia veneering ceramics. Dent Mater. 2006; 22:857–863.
18. Taskonak B, Borges GA, Mecholsky JJ Jr, Anusavice KJ, Moore BK, Yan J. The effects of viscoelastic parameters on residual stress development in a zirconia/glass bilayer dental ceramic. Dent Mater. 2008; 24:1149–1155.
19. Taskonak B, Mecholsky JJ Jr, Anusavice KJ. Residual stresses in bilayer dental ceramics. Biomaterials. 2005; 26:3235–3241.
20. Tan JP, Sederstrom D, Polansky JR, McLaren EA, White SN. The use of slow heating and slow cooling regimens to strengthen porcelain fused to zirconia. J Prosthet Dent. 2012; 107:163–169.
21. Aboushelib MN, Kleverlaan CJ, Feilzer AJ. Microtensile bond strength of different components of core veneered all-ceramic restorations. Part 3: double veneer technique. J Prosthodont. 2008; 17:9–13.
22. Benetti P, Della Bona A, Kelly JR. Evaluation of thermal compatibility between core and veneer dental ceramics using shear bond strength test and contact angle measurement. Dent Mater. 2010; 26:743–750.
23. Benetti P, Pelogia F, Valandro LF, Bottino MA, Bona AD. The effect of porcelain thickness and surface liner application on the fracture behavior of a ceramic system. Dent Mater. 2011; 27:948–953.
24. Scherrer SS, Cesar PF, Swain MV. Direct comparison of the bond strength results of the different test methods: a critical literature review. Dent Mater. 2010; 26:e78–e93.
25. Tam LE, Pilliar RM. Fracture toughness of dentin/resin-composite adhesive interfaces. J Dent Res. 1993; 72:953–959.
26. Ruse ND, Troczynski T, MacEntee MI, Feduik D. Novel fracture toughness test using a notchless triangular prism (NTP) specimen. J Biomed Mater Res. 1996; 31:457–463.
27. Tam LE, Khoshand S, Pilliar RM. Fracture resistance of dentin-composite interfaces using different adhesive resin layers. J Dent. 2001; 29:217–225.
28. Anunmana C, Anusavice KJ, Mecholsky JJ Jr. Interfacial toughness of bilayer dental ceramics based on a short-bar, chevron-notch test. Dent Mater. 2010; 26:111–117.
29. Pashley DH, Sano H, Ciucchi B, Yoshiyama M, Carvalho RM. Adhesion testing of dentin bonding agents: a review. Dent Mater. 1995; 11:117–125.
30. Phrukkanon S, Burrow MF, Tyas MJ. The influence of cross-sectional shape and surface area on the microtensile bond test. Dent Mater. 1998; 14:212–221.