Abstract
PURPOSE
The aim of this Fourier transform infrared (FTIR) spectroscopic study was to measure the degree of conversion (DC) of dual-cured resin cements light-irradiated through zirconia ceramic disks with different thicknesses using various light-curing methods.
MATERIALS AND METHODS
Zirconia ceramic disks (KT12) with three different thicknesses (1.0, 2.0, and 4.0 mm) were prepared. The light transmittance of the disks was measured using ultraviolet visible near-infrared spectroscopy. Four different light-curing protocols were used by combining two curing light modes (Elipar TriLight (standard mode) and bluephase G2 (high power mode)) with light-exposure times of 40 and 120 seconds. The DCs of the two dual-cured resin cements (Duo-Link and Panavia F2.0) light-irradiated through the disks was analyzed at three time intervals (3, 7, and 10 minutes) by FTIR spectroscopy. The data was analyzed using repeated measures ANOVA (α=.05).Two-way ANOVA and Tukey post hoc test were used to analyze the 10 minute DC results.
RESULTS
The 1.0 mm thick disk exhibited low light transmittance (<25%), and the transmittance decreased considerably with increasing disk thickness. All groups exhibited significantly higher 10 minute DC values than the 3 or 7 minute values (P<.05), but some exceptions were observed in Duo-Link. Two-way ANOVA revealed that the influence of the zirconia disk thickness on the 10 minute DC was dependent on the light-curing methods (P<.001). This finding was still valid even at 4.0 mm thickness, where substantial light attenuation took place.
Zirconia ceramics based on yttria-stabilized tetragonal zirconia polycrystals (Y-TZP) have become an attractive framework material in dentistry, mainly because of their superior mechanical properties, fracture toughness, and biocompatibility.1-3 The clinical use of zirconia ceramics requires a reliable technique for cementation in combination with adequate luting agents.4 Full-coverage zirconia restorations and fixed partial prostheses not requiring high retention might still be luted using conventional cements such as resin-modified glass ionomer cements.5 On the other hand, the use of such conventional cements with high-strength zirconia ceramics does not appear to achieve durable bond strength, regardless of the pre-treatment procedure.4 Although an adequate adhesive cementation protocol for zirconia ceramics has not been established, resin-based luting agents tend to be preferred over conventional cements. A combination of sandblasting and resin luting agents containing organophosphate ester monomers, such as 10-methacryloyloxydecyl dihydrogenphosphate (MDP), has been reported to produce a relatively strong and durable bond to zirconia.6,7
In general, zirconia ceramics have high refractive indices, low absorption coefficients, and high opacity in the UV/vis spectrum.8 The maximal opaque effect of zirconia ceramic has been attributed to the dispersed particles being slightly greater in size than the given wavelength of light and the different refractive index of the matrix.8 Indeed, zirconia cores are less translucent than glass, lithium disilicate or alumina cores.9 Although this increased opacity can be useful for masking dischromatic abutment teeth or metal posts and cores, it compromises the photo-polymerization of dual-cured resin cements used under the restorations. If chemical polymerization cannot compensate sufficiently for the reduced photo-polymerization caused by such light attenuation, insufficient hardening of a dual-cured resin cement can lead to the early debonding failure of adhesive zirconia restorations as well as post-operative sensitivity, microleakage, and recurrent caries.10 However, there are few data available on the degree of conversion (DC) of dual-cured resin cements used for the adhesive cementation of relatively opaque zirconia ceramic-based indirect restorations.
Accordingly, the aim of this study was to quantify the DC of dual-cured resin cement light-irradiated through simulated zirconia ceramic disks with different thicknesses. The light transmittance of the zirconia ceramic disks was also investigated.
For this study, two commercially available dual-cured resin cements were examined: the conventional cement Duo-Link and the MDP containing cement Panavia F2.0. Table 1 lists the manufacturers, main compositions, and batch numbers. Zirconia ceramic disks (12 mm diameter) with three thicknesses (1.0, 2.0, and 4.0 mm) were prepared by sintering a commercial zirconia block (KT12; Katana, Noritake Dental Supply Co., Ltd., Miyoshi, Japan; batch number: bgydx) according to the manufacturer's instructions and polishing both surfaces of each disk with silicon carbide paper up to #2000.
The light transmittance of the zirconia ceramic disks was measured in the wavelength range of 350-550 nm with an ultraviolet visible near-infrared (UV-Vis-NIR) spectrophotometer (UV-3600, Shimadzu Co., Kyoto, Japan) equipped with an integrating sphere (ISR-3100, Shimadzu Co.). The measurement beam was set up to pass through a zirconia disk and then through a polyester film (Fig. 1A).
The degree of C=C conversion for the resin cements light-irradiated through the zirconia disks with different thicknesses was examined by Fourier transform infrared (FTIR, IRPrestige-21, Shimadzu Co., Japan) spectroscopy with an attenuated total reflectance (ATR, MIRacle, Pike Technologies Inc., USA) unit. Prior to analysis, adhesive tape was placed around the ATR germanium surface to act as a vertical spacer, ensuring a standard specimen thickness (approximately 50 µm).11 A small amount of freshly mixed (uncured) material (n=5) was pressed against the ATR surface and covered with polyester film. The absorbance spectrum was then acquired by scanning the specimens 10 times over a 1670-1550 cm-1 range with a resolution of 4 cm-1.
In this study, one quartz tungsten halogen (QTH) curing light (Elipar TriLight, 3M ESPE, Germany) and one light-emitting diode (LED) curing light (bluephase G2, Ivoclar Vivadent, Liechtenstein) were used. The two curing lights were used in standard mode and highpower mode (code designations: TS and BH), respectively. The output intensity of the curing lights (750 and 1150 mW/cm2 for TS and BH, respectively) was constantly measured during the experiment by the respective radiometers. Four different light-curing methods were used by combining one of the two curing lights (TS or BH) with one of the two light-exposure time options (40 or 120 seconds) (code designations: TS-40, TS-120, BH-40, and BH-120). The deposited material was light-irradiated by placing the light guide tip onto the polyester film (disk thickness 0). A zirconia disk with various thicknesses (1.0, 2.0 or 4.0 mm) was placed over the polyester film12 and the deposited materials were light-irradiated by placing the light guide tip onto the top of the zirconia disk so that light could pass through the disk and polyester film over the material (Fig. 1B). Each light-irradiated material was left on the ATR surface and further spectra were obtained at 3, 7, and 10 minutes after post-mixing.
The DC was determined from the aliphatic C=C peak at 1638 cm-1, and the aromatic C=C peak at 1608 cm-1 was used for internal calibration. The DC was then calculated by comparing the heights of the peaks for the methacrylate vinyl group in the cured material with those in the uncured material using the formula13: DC (%) = (1-C/U) × 100, where C and U are the normalized absorption peak heights for the cured and uncured materials, respectively.
The differences between the DCs at each time point (3 or 7 minutes) and the value at 10 minutes under the same experimental conditions were analyzed using repeated measures ANOVA.12 Two-way ANOVA was also performed on the 10 minute DC results using the light-curing method and zirconia disk thickness as discriminating variables. The group differences were investigated further using a Tukey post hoc test.12 All statistical analyses were performed using SPSS 17.0 for Windows (SPSS Inc., Chicago, IL, USA) at a significance level of 0.05.
Fig. 2 shows the light transmittance of the KT12 zirconia disks with different thicknesses. The 1.0 mm thick disk exhibited low light transmittance (<25%) over all wavelengths tested, and the transmittance decreased drastically with increasing disk thickness.
Tables 2 and 3 list the mean DCs and standard deviations for the two dual-cured resin cements light-irradiated through the KT12 zirconia disks as a function of time. All the groups exhibited significantly higher 10 minute DC values than the 3 or 7 minute values except for Duo-Link (TS-120 and BH-120/2.0 mm; BH-40 and BH-120/4.0 mm), which showed no significant differences between the 7 and 10 minute values.
Fig. 3 shows the 10 minute DCs of the two dual-cured resin cements. For both materials, two-way ANOVA showed that the effect of the zirconia disk thickness on the decrease in DC was dependent on the light-curing method. At a thickness of 0 mm, the mean DC values for Duo-Link and Panavia F2.0 varied in the range of 59.2-61.9% and 39.1-52.6%, respectively. The values decreased gradually with increasing disk thickness for all groups. The tendency depended on the material, curing method, and disk thickness. At 4.0 mm thickness, the mean DC values for Duo-Link and Panavia F2.0 decreased up to the range of 27.5-41.6% and 21.7-30.3%, respectively. At this thickness, BH-120 yielded the highest DC values of any of the light-curing methods in both materials.
Resin composites based on bisphenol A diglycidyl methacrylate (Bis-GMA)or similar monomers have been reported to produce a 50 to 75% DC after polymerization.13-16 Although a minimum clinically acceptable DC has not yet been established,17 the present study showed that the DC of dual-cured resin cements under zirconia restorations can vary greatly depending on the light-curing technique used.
Fig. 2 shows that even the thin zirconia disk exhibited low light transmittance. This is not surprising considering that zirconia frameworks have a level of opacity even with a limited core thickness due mainly to their high density and homogeneity.8 The light transmittance of the zirconia disks increased at higher wavelengths according to the Rayleigh scattering equation.18,19 Therefore, under zirconia restorations, dual-cured resin cement containing camphorquinone (CQ), which has an absorbance peak at approximately 470 nm,20,21 photo-polymerize more properly than those containing photo-initiators with absorbance peaks at lower wavelengths.17 Both dual-cured resin cements used in this study appear to have a CQ/amine photo-initiation system (Table 1),12,22,23 which are potentially compatible with all types of curing lights.24,25
Curing lights at high intensity appear preferable for increasing the amount of light reaching the dual-cured resin cements.26 In the present study, two curing lights with two high intensity modes were selected. The light transmittance results (Fig. 2) suggest that the light delivered to the resin cement is substantially attenuated. Even in these situations, therefore, additional chemical polymerization of dual-cured resin cements should be sufficient to compensate for the limited photo-polymerization and ensure a final maximum cure.22
In light-cured or dual-cured resin composites, 90% of the DC measured after 24 hours develops within the first 10 minutes.12,13,27 Therefore, an experimental period of 10 minutes, corresponding to the initial setting phase, in the present study is likely to be sufficient and clinically relevant for estimating the setting characteristics of the dual-cured materials.13,17 When two pastes of a dual-cured resin cement are mixed, the radicals formed cannot react with the monomers due to the action of inhibitors and oxygen during the induction period.24 After the inhibitors of the dual-cured resin cement are consumed rapidly by the photo-initiated radicals under subsequent light irradiation, chemical polymerization and photo-polymerization begin to proceed,13 the former tending to be slower than the latter to ensure an extended working time.12,28 The DC values obtained at each time interval should be considered to be a result of both polymerization mechanisms.
Overall, the 10 minute DC values in Panavia F2.0 were lower than those in Duo-Link (Fig. 3). At 0-mm thickness, the four light-curing methods did not produce any significant differences in the 10 minute DC in the case of Duo-Link, whereas TS-40 produced a significantly lower value than the other three methods in Panavia F2.0. This suggests that the curing of Panavia F2.0 is more dependent on light-irradiation and might not cure well unless properly light-irradiated.22 This may be because the Panavia F2.0 paste contains the acidic functional monomer, MDP, which interacts chemically with the amine initiator and can impair chemical polymerization,17 and a low amount of amine, which is a co-initiator for chemical initiation (Table 1).23 Although the Panavia F2.0 paste also incorporates the sodium salt of aryl sulfinic acid to overcome this incompatibility,17,23 its efficiency is unclear at present.25 In clinical dental practice, the combined use of amine-containing ED Primer in the Panavia F2.0 system might enhance the polymerization reaction and increase the final DC.12,23
At 1.0 mm thickness, the 10 minute DC values for TS-120, BH-40, and BH-120 in Duo-Link were equivalent to those obtained without the zirconia disk (Fig. 3). This suggests that an additional chemical polymerization mechanism compensates for the decreased photo-polymerization of the material under the three light-curing methods.29 In Panavia F2.0, however, the 10 minute DC values at 1.0 mm thickness were significantly lower than those at a thickness of 0 mm, irrespective of the light curing-protocols. This confirms that chemical polymerization is more efficient in Duo-Link than in Panavia F2.0, even though the latter material contains two photo-initiation systems, including the CQ/amine system.23
Most experimental groups in both materials exhibited significantly higher 10 minute DC values than the 3 or 7 minute DC values (Table 2 and Table 3). However, certain groups in Duo-Link (TS-120 and BH-120/2.0 mm, BH-40 and BH-120/4.0 mm) exhibited different curing rates, suggesting that light-curing methods alter the curing kinetics of dual-cured resin cements. The 10 minute DC values at 2.0 mm thickness did not decrease consistently compared to those at 1.0 mm thickness, but rather depended on the resin cement and light-curing method used (Fig. 3). In particular, the value of Duo-Link light-irradiated in BH-120 was higher at 2.0 mm than at 1.0 mm at 3 minute observation time; the values between the two thicknesses were statistically similar at 10 minutes (Table 2 and Fig. 3). Under the light-curing condition (BH-120), the 7 minute DC did not differ significantly from the 10 minute DC at 2.0 mm thickness. This indicates a faster curing rate of the material at 2.0 mm thickness than at 1.0 mm thickness. Therefore, the curing kinetics of dual-cured resin cements appears to be more complex than a simple process of photo-polymerization followed by additional chemical polymerization. The light-activated dual-cured resin cements can interfere with the self-curing (chemical polymerization) mechanism.22
In most zirconia-based restorations, a thinner zirconia coping is veneered with other ceramic materials. Therefore, a pure 4.0 mm thick zirconia ceramic might be rare in clinical situations and should be considered an experimental means of evaluating the dual-curing capacity of the resin cement. The 10 minute DC values at 4.0 mm thickness were significantly lower than those at 2.0 mm thickness in all groups (Fig. 3). Nevertheless, the light was not obscured completely even at the 4.0 mm thickness.8 As a result, even a small amount of light on the dual-cured resin cement can make a significant difference in the final DC values. The BH-120 mode consistently yielded the highest DC values at 4.0 mm thickness for both materials. In Panavia F2.0, TS-120 achieved a statistically similar value to BH-120. This suggests that longer light exposure raises the contribution of photo-polymerization by increasing slightly the amount of light required to form radical initiating species for the reaction.30
The experimental design of this study had certain limitations. Only one zirconia brand was used in a very simplified form. In reality, zirconia copings have different levels of light transmittance,9 and outer treatments, such as veneering, and inner treatments, such as sandblasting, can cause some changes in the optical properties of the restoration. These changes can affect the conversion of dual-cured resin cements used under zirconia restorations. Although the two curing lights used have similar wavelength ranges, their emission spectra were not identical.31,32 Finally, all specimens were prepared at room temperature, not at body temperature, which can increase the mobility of the molecules during a chemical reaction and increase the DC in clinical situations.17
The results of this in vitro study suggest that the additional chemical polymerization mechanism of dual-cured resin cements work effectively when photo-polymerization is impaired by insufficient light reaching the material. Nevertheless, a proper light-curing technique is still important for maximizing the monomer to polymer conversion of dual-cured resin cements because such chemical polymerization might not completely compensate for the insufficient photo-polymerization, particularly under thick zirconia restorations. Long-term clinical studies will be needed to determine the final behavior of bonded zirconia restorations in an oral environment. Further research will also be needed to clarify the advantages and disadvantages of the inherent opacity of zirconia ceramics in terms of esthetics and bonding.
Although the inherent optical properties of zirconia ceramic may be esthetically pleasing, they impair the photopolymerization of dual-cured resin cement beneath zirconia-based indirect restorations due to light attenuation. This study suggests that the additional chemical polymerization mechanism works effectively when photo-polymerization is impaired. Nevertheless, proper light-curing of dual-cured resin cements will be needed to optimize the photo-polymerization along with chemical polymerization.
Figures and Tables
![]() | Fig. 1Schematic diagrams showing the measurements of the light transmittance of zirconia disk (A) and the degree of conversion for the resin cements light-irradiated through zirconia disk. |
![]() | Fig. 3Ten minute degree of conversion of the two resin cements. The vertical bar indicates the standard deviation. Within a material, the same lower case letters indicate statistically similar values within each thickness; the horizontal lines above the adjacent bars indicate statistically similar values within each curing mode (ANOVA and Tukey' test, P>.05). TS: Elipar TriLight, standard mode; BH: bluephase G2,high power mode; 40 or 120: light-exposure time (seconds). |
Table 2
Degree of conversion (%) of Duo-Link light-irradiated through KT12 zirconia disks (mean ± SD, n=5)
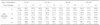
ACKNOWLEDGEMENTS
The authors are grateful to spectrometer specialist Kwansoo Kim (Dong-il SHIMADZU Co., Seoul, Korea) for his technical support with the spectroscopy work.
References
1. Attia A, Lehmann F, Kern M. Influence of surface conditioning and cleaning methods on resin bonding to zirconia ceramic. Dent Mater. 2011; 27:207–213.
2. Matinlinna JP, Lassila LV. Enhanced resin-composite bonding to zirconia framework after pretreatment with selected silane monomers. Dent Mater. 2011; 27:273–280.
3. Piconi C, Maccauro G. Zirconia as a ceramic biomaterial. Biomaterials. 1999; 20:1–25.
4. Piwowarczyk A, Lauer HC, Sorensen JA. The shear bond strength between luting cements and zirconia ceramics after two pre-treatments. Oper Dent. 2005; 30:382–388.
5. Roediger M, Gersdorff N, Huels A, Rinke S. Prospective evaluation of zirconia posterior fixed partial dentures: fouryear clinical results. Int J Prosthodont. 2010; 23:141–148.
6. Blatz MB, Sadan A, Kern M. Resin-ceramic bonding: a review of the literature. J Prosthet Dent. 2003; 89:268–274.
7. Tanaka R, Fujishima A, Shibata Y, Manabe A, Miyazaki T. Cooperation of phosphate monomer and silica modification on zirconia. J Dent Res. 2008; 87:666–670.
8. Vagkopoulou T, Koutayas SO, Koidis P, Strub JR. Zirconia in dentistry: Part 1. Discovering the nature of an upcoming bioceramic. Eur J Esthet Dent. 2009; 4:130–151.
9. Baldissara P, Llukacej A, Ciocca L, Valandro FL, Scotti R. Translucency of zirconia copings made with different CAD/CAM systems. J Prosthet Dent. 2010; 104:6–12.
10. El-Mowafy OM, Rubo MH. Influence of composite inlay/onlay thickness on hardening of dual-cured resin cements. J Can Dent Assoc. 2000; 66:147.
11. Kim MJ, Kim YK, Kim KH, Kwon TY. Shear bond strengths of various luting cements to zirconia ceramic: surface chemical aspects. J Dent. 2011; 39:795–803.
12. Aguiar TR, Di Francescantonio M, Arrais CA, Ambrosano GM, Davanzo C, Giannini M. Influence of curing mode and time on degree of conversion of one conventional and two self-adhesive resin cements. Oper Dent. 2010; 35:295–299.
13. Yan YL, Kim YK, Kim KH, Kwon TY. Changes in degree of conversion and microhardness of dental resin cements. Oper Dent. 2010; 35:203–210.
14. Bayne SC. Dental biomaterials: where are we and where are we going? J Dent Educ. 2005; 69:571–585.
15. Gerth HU, Dammaschke T, Züchner H, Schäfer E. Chemical analysis and bonding reaction of RelyX Unicem and Bifix composites-a comparative study. Dent Mater. 2006; 22:934–941.
16. Ferracane JL, Greener EH. The effect of resin formulation on the degree of conversion and mechanical properties of dental restorative resins. J Biomed Mater Res. 1986; 20:121–131.
17. Vrochari AD, Eliades G, Hellwig E, Wrbas KT. Curing efficiency of four self-etching, self-adhesive resin cements. Dent Mater. 2009; 25:1104–1108.
18. Dias MC, Piva E, de Moraes RR, Ambrosano GM, Sinhoreti MA, Correr-Sobrinho L. UV-Vis spectrophotometric analysis and light irradiance through hot-pressed and hot-pressed-veneered glass ceramics. Braz Dent J. 2008; 19:197–203.
19. Brodbelt RH, O'Brien WJ, Fan PL. Translucency of dental porcelains. J Dent Res. 1980; 59:70–75.
20. Leonard DL. Light-emitting diode curing lights, Part II. J Esthet Restor Dent. 2003; 15:251–255.
21. Kim YK, Kim SK, Kim KH, Kwon TY. Degree of conversion of dual-cured resin cement light-cured through three fibre posts within human root canals: an ex vivo study. Int Endod J. 2009; 42:667–674.
22. Pegoraro TA, da Silva NR, Carvalho RM. Cements for use in esthetic dentistry. Dent Clin North Am. 2007; 51:453–471.
23. Faria-e-Silva AL, Moraes RR, Ogliari FA, Piva E, Martins LR. Panavia F: the role of the primer. J Oral Sci. 2009; 51:255–259.
24. Van Landuyt KL, Snauwaert J, De Munck J, Peumans M, Yoshida Y, Poitevin A, Coutinho E, Suzuki K, Lambrechts P, Van Meerbeek B. Systematic review of the chemical composition of contemporary dental adhesives. Biomaterials. 2007; 28:3757–3785.
25. Kwon TY, Bagheri R, Kim YK, Kim KH, Burrow MF. Cure mechanisms in materials for use in esthetic dentistry. J Investig Clin Dent. 2012; 3:3–16.
26. Mills RW, Jandt KD, Ashworth SH. Dental composite depth of cure with halogen and blue light emitting diode technology. Br Dent J. 1999; 186:388–391.
27. Rueggeberg FA, Caughman WF. The influence of light exposure on polymerization of dual-cure resin cements. Oper Dent. 1993; 18:48–55.
28. Arrais CA, Giannini M, Rueggeberg FA. Effect of sodium sulfinate salts on the polymerization characteristics of dual-cured resin cement systems exposed to attenuated light-activation. J Dent. 2009; 37:219–227.
29. Pazin MC, Moraes RR, Gonçalves LS, Borges GA, Sinhoreti MA, Correr-Sobrinho L. Effects of ceramic thickness and curing unit on light transmission through leucite-reinforced material and polymerization of dual-cured luting agent. J Oral Sci. 2008; 50:131–136.
30. Jakubiak J, Allonas X, Fouassier JP, Sionkowska A, Andrzejewska E, Linden LÅ, Rabek JF. Camphorquinone-amines photoinitating systems for the initiation of free radical polymerization. Polymer. 2003; 44:5219–5226.
31. Kameyama A, Hatayma H, Kato J, Haruyama A, Teraoka H, Takase Y, Yoshinari M, Tsunoda M. Spectral characteristics of light-curing units and dental adhesives. J Photopolym Sci Technol. 2011; 24:411–416.
32. Miletic V, Santini A. Micro-Raman spectroscopic analysis of the degree of conversion of composite resins containing different initiators cured by polywave or monowave LED units. J Dent. 2012; 40:106–113.