Abstract
PURPOSE
To evaluate adherence of human gingival fibroblasts (HGFs) to transmucosal abutment of dental implant with different surface conditions with time and to investigate the roles of focal adhesion linker proteins (FALPs) involved in HGFs adhesion to abutment surfaces.
MATERIALS AND METHODS
Morphologies of cultured HGFs on titanium and ceramic discs with different surface were observed by scanning electron microscopy. Biocompatibility and focal adhesion were evaluated by ultrasonic wave application and cell viability assay. FALPs expression levels were assessed by RT-PCR and western blot.
RESULTS
There seemed to be little difference in biocompatibility and adhesion strength of HGFs depending on the surface conditions and materials. In all experimental groups, the number of cells remaining on the disc surface after ultrasonic wave application increased more than 2 times at 3 days after seeding compared to 1-day cultured cells and this continued until 7 days of culture. FALPs expression levels, especially of vinculin and paxillin, also increased in 5-day cultured cells compared to 1-day cultured fibroblasts on the disc surface.
Dental implants are mostly preferred in the dental prosthetic field for partial or complete edentulous patients. In spite of their popularity, many failure cases due to infection such as post-operative peri-implantitis have been reported.1 There are several common clinical and histological features between the free marginal gingiva and the mucosa surrounding an implant.2-4 The tooth-gingiva interface in the natural tooth consists of 3 parts, gingival sulcus, epithelial attachment, connective tissue attachment. Dental implants also normally keep the epithelial attachment and submucosal attachment intact, which serves as a biological seal and plays an important role as a blocking barrier preventing food impaction and bacterial infiltration into the implant-tissue interface. The epithelial attachment on the implant and tooth is composed of hemidesmosomes and a basal lamina, and attachment of fibroblasts is maintained by focal adhesion complex, consisting of proteins that communicate with extracellular matrix (ECM) and intracellular cytoskeleton.5,6 Integrin, one of the transmembrane proteins, indirectly connects ECM with actin filaments through a series of linker proteins.7 Linker proteins, represented by talin, paxillin, vinculin etc. not only stabilize the focal adhesion complex by regulating gene expression through the feedback system, but they also transfer various signals from ECM to cytosol.8
Osseointegration is the most critical factor contributing to dental implant stabilization. On the other hand, the firm attachment of the epithelium and connective tissue to the dental implant results in complete sealing and protection of subgingival bony tissues from the oral environment in the end, and it is the most influential factor for long-term maintenance of dental implants.9 Many current studies on dental implants are being conducted with the aim to improve osseointegration at the bone-implant interface through regulation of osteocalcin and alkaline phosphatase during osteoblast differentiation.10,11 Although some studies have also been conducted on the main protein of the attachment apparatus such as integrin for improving soft tissue attachment, they were only limited to morphological analysis of focal contacts containing integrin and vinculin.12-15 Studies with the aim of quantification of cell adhesion strength to the implant and evaluation of the roles of linker proteins are very rare.
Biocompatibility and bioadhesive property of an abutment which has a supragingival and gingival-passing structure not only makes it possible to form the functional soft tissue, but also play an important role in maintaining osseointegration. To improve the esthetic appeal and to make the dental laboratory process easier, materials such as ceramics, gold alloy except for titanium are used as abutment materials. Although the demand for the use of ceramic in anterior teeth replacement is increasing,16,17 the soft tissue healing process and successful attachment of the mucosa to the ceramic abutment material have not been clearly elucidated.17,18 Therefore, firstly, this study was aimed at comparing the differences in attachment of gingival fibroblasts to the transmucosal abutment and proliferation depending on different materials and surface roughness of the transmucosal abutment. Next, adhesion strength of fibroblasts to different abutment with various surface conditions was estimated and then changes in expression of linker proteins such as talin, paxillin, vinculin etc. was analysed.
In order to fit into the well of 24-well tissue culture plates, 15 mm-diameter, 1 mm-thickness discs using titanium (Grade III commercially pure titanium) and ceramics (Ceramic abutment®: ZrO2 94.4%, Y2O3 5.25%, Al2O3 0.1%, H2O 0.1%) were manufactured by Megagen Implant Co. (Daegu, Korea). To represent 5 different transmucosal abutment surfaces, the discs were polished to give a glassy appearance and were used as the polished surface and the discs were cut without polishing and were used as the machined surface. Additionally, titanium coated with titanium nitride was used. Nine specimens were used for each individual group respectively. After surface treatment of each specimen, ultrasonic cleaning in methanol and distilled water respectively and autoclave sterilization were performed. To identify the surface roughness, surfaces of each specimen were observed and photographed using a JSM-5400 (Jeol, Japan) scanning electron microscopy (SEM).
Healthy gingival tissue was obtained from Korean patients at Chonnam National Hospital and HGFs were cultured from it. All procedures were performed with informed consent from the subjects, and the Ethics Committee of Chonnam National University and Hospital approved the protocol. HGFs were cultured in a growth medium comprised of Dulbeco's Modified Eagle's Medium (DMEM), 10% fetal bovine serum (Gibco-BRL, Grand Island, NY, USA), 100 U/mL penicillin, and 100 mg/mL streptomycin in an atmosphere containing 5% CO2 at 37℃. At 1 day and 5 days after seeding respectively, cells were fixed with 4% paraformaldehyde for 10 minutes. Phosphate Buffered saline (PBS) washing and dehydration using ethanol were followed. After air-drying, surfaces were sputter-coated with gold and observed by SEM. HGFs on tissue culture plates were used as controls.
The MTT[3-(4,5-dimethylthiazol-2yl)-2,5-diphenyl-2H-tetrazolium bromide, Invitrogen, Carlsbad, CA, USA] assay was used to indirectly measure the cell viability at 1, 3, 5 and 7 days after seeding respectively. To compare the cell adhesion strength after 2 × 106 HGFs were plated onto each disc fitted for 24-well tissue culture plates, ultrasonic waves were applied for 10 minutes using SD-80W® ultrasonic cleaner (SD-ultrasonic Co., LTD, Korea) After the treatments, MTT was added to the growth medium at a final concentration of 0.1 mg/mL for each times and cultivated at 37℃ for 4 hours. The reaction product of MTT was detected using dimethylsulfoxide (DMSO, Invitrogen, Carlsbad, CA, USA) and the optical density (OD) was spectrophotometrically measured at 570 nm using an ELISA reader (BIO Tek Instruments, Winooski, VM, USA) with DMSO as the blank. The results were compared with the values obtained from fibroblasts grown on disc surfaces without ultrasonic wave application.
The total mRNA was extracted using a Trizol® Reagent (Gibco-BRL, Grand Island, NY, USA). Before PCR was performed, contaminating DNA, if any, was removed by treating extracted DNA with DNase I (Gibco-BRL, Grand Island, NY, USA). RNA quantification was conducted using a UV spectrophotometer and those RNA samples were qualified by obtaining OD 260/280 ratios > 1.8. AccPower® RT PreMix (Bioneer, Daejeon, Korea) and AccPower® PCR PreMix (Bioneer, Daejeon, Korea) were used for reverse transcription and the PCR reaction respectively. Perkin-Elmer GeneAmp PCR system 2400 (Applied Biosystems/Perkin Elmer, Foster City, CA, USA) was used for PCR reaction. PCR conditions were as follows: denaturation for 30 seconds at 95℃, annealing for 30 seconds at 57℃ and a 30 second extension step at 72℃. Preliminary experiments were performed to determine the optimal number of PCR cycles. Products were resolved on a 1% agarose gel and visualized using SYBR®Safe DNA gel stain (Invitrogen, Carlsbad, CA, USA). The product size was estimated based on a 100 bp marker (Takara, Otsu, Shiga, Japan). Primer sequences used are presented in Table 1.
After the cold PBS washing, cell lysation and homogenization in a lysis buffer were followed. Cell lysates centrifugation was conducted and protein concentrations in the supernatants were determined using Amersham GeneQuant Pro (Amersham-Pharmacia Biotech, Arlington Heights, IL, USA). After 5 min boiling of equal amounts of the proteins in a 3X SDS sample buffer and loading onto 15% SDS-polyacrylamide gel, the resolved proteins were transferred to a Protran nitrocellulose membrane (Whatman GmbH, Dassel, Germany). The membrane blocking was conducted with a TBS-T buffer [10 mM Tris-buffered isotonic saline (pH 7.0), 0.1% merthiolate, 0.1% Tween-20] including 5% skim milk for 1 hour at room temperature. The membrane was then incubated with the rabbit polyclonal primary antibody raised against paxillin or vinculin (1:1000 dilution, Cell signaling Technology, Beverly, MA, USA) for overnight at 4℃ with gentle shaking, respectively. β-actin primary antibody (Sigma-Aldrich Co., ST Louis, MO, USA) was used as the guidance. After the several TBS-T washing, the membranes were incubated with HRP-labeled anti-rabbit secondary Ab (1:3000 dilutions, Cell signaling Technology, Beverly, MA, USA) for paxillin or vinculin, anti-mouse secondary Ab (1:3000 dilutions, Cell Signaling Technology, Beverly, MA, USA) for β-actin, for 1 hour at room temperature. The reaction product was visualized with HRP substrate luminol reagent (Millipore Corporation, Billerica, MA, USA). LAS4000 mini loaded with image Reader LAS-4000 software (Fujifilm, Minatoku, Tokyo, Japan) was used for photograph.
Data are expressed as mean standard deviation. Statistic analysis was performed using an ANOVA test. The results were taken to be significant at a probability level of P<.05. For all experiments, three independent experiments were performed.
Photographs taken through a SEM of each modified surface disc are depicted in Fig. 1 (From A to E). In case of the machined titanium surface (MT) group, several thick groove patterns running in concentric circles were observed. The polished titanium surface (PT) group revealed relatively smoother surfaces than the MT group, but some surfaces with irregularly distributed grooves presenting several scratching and pitting were still observed. Titanium nitride coating on a polished titanium surface (CT) did not significantly affect the original surface texture and showed a similar picture as the PT group. While the machined ceramic surface (MC) group showed irregular texture with multiple concavities and convexities, the surfaces of polished ceramic surface (PC) group were the smoothest among all the above experimental groups.
HGFs on days 1 and 5 after seeding on five different disc surfaces were demonstrated by SEM (Fig. 1 from F to J and from K to O). Overall, favorable adhesion and good spread of fibroblasts on all modified surfaces tested were observed. However, there were some differences in detailed cellular morphology between each experimental group depending on the surface type and growing period. One day cultured fibroblasts on the MT surfaces were entrapped primarily in the gap between the thick macrogrooves and elongated along the groove patterns. In contrast, diverse cellular shapes such as spindle, round, elongated or multipolar with cellular processes, appeared on the PT surfaces. Cells with similar morphology were observed on the CT and MC surfaces with the exception of size and the number of cellular processes of each cell. In case of the cells cultured on the MC surfaces, the majority of cells had a flat and short morphology and the cell density was lower than that in the other groups. Meanwhile, after 5 days of culture, cellular growth seemed to be denser in all the groups. Each cell appeared larger, more elongated, more spread out and more firmly adhering to the surfaces than the 1-day cultured fibroblasts.
To compare the influence of the various surface modifications on cell growth and proliferation, MTT tests were performed after cells were cultured for 1, 3, 5 and 7 days on each disc. The mitochondrial dehydrogenase activities demonstrated similar trends in all the groups without significant differences (Fig. 2). In other words, the mitochondrial dehydrogenase activities increased in a time-dependent manner. When compared with the cells grown on all modified surfaces tested, the activity of the fibroblasts cultured on 24-well culture plates which were used as a control was higher. There were no statistically significant differences between all groups with modified surfaces.
To compare the adhesion strength of cells cultured on various modified surfaces, ultrasonic waves were applied to 24-well tissue culture plates including the discs on which HGFs were cultured for 1, 3, 5 and 7 days respectively as explained in 'Materials and Methods' section. After the mechanical forces were applied, mitochondrial dehydrogenase activities of the fraction of adherent cells were measured. The values obtained were then compared with the groups in which the mechanical forces were not applied. Fibroblasts cultured on 24-well culture plates were used as the control. Fibroblasts cultured for 1 day on various modified surfaces were easily detached up to more than twothirds, while, after 3 days of culture, the rate of detachment was significantly decreased. This trend was common in all tested groups including the control. In addition, although generally data obtained from the experimental groups were lower than that of the control, there were no significant differences among the tested groups (Fig. 3).
To investigate the contribution of focal adhesion linker proteins in HGFs adhesion on the abutment surfaces, after the cells were cultured for 1, 3, 5 and 7 days on modified surfaces, semi-quantitative RT-PCR assay was conducted to detect the expressions of several focal adhesion linker proteins such as vinculin, paxillin, tensin, zyxin, talin, actinin and FAK. The mRNA expression levels of most focal adhesion linker proteins in 5-day and 7-day cultured cells were little different in each experimental group with the exception of tensin and FAK which showed very faint expressions (Fig. 4A). However, the results of changes in mRNA expression levels of focal adhesion linker proteins with time showed that mRNA expression of some focal adhesion linker proteins, especially, vinculin, paxillin, tensin and FAK increased in a time-dependent manner irrespective of the culture conditions used. The expression pattern of the other focal adhesion linker proteins such as zyxin, talin and actinin was not affected by time (Fig. 4B). To confirm the correlation between the focal adhesion linker proteins and HGFs adhesion on abutment surfaces, after the cells were cultured for 1 day and 5 days on modified surfaces respectively, western blot was performed to compare the expressions of focal adhesion linker proteins, especially, vinculin and paxillin which showed a distinctive increase as time passed, on RT-PCR assay. As expected, both vinculin and paxillin protein expressions increased in all 5-day cultured groups tested including the control compared with the 1-day cultured groups (Fig. 5).
Surface characteristics including roughness and morphology are the most influencing factors in cellular behavior such as survival, attachment and growth. Although there have been extensive investigations of cellular response to dental implant according to surface topography, it still remains controversial. To date several lines of evidence indicate that the implant surfaces with microrough topographies exhibit positive effects such as increased bone-to-implant contact and increased osteoblastic differentiation and osteogenesis in vitro and vivo.19-23 In contrast, some researchers demonstrated that the smooth surfaces allowed for a closer contact between the cells and the substrate than the rougher surfaces do.24,25 Although it is truly believed that surface roughness can influence cellular adhesion, it appears probable that there is a critical scale of surface topography which can change the cellular response. Recently, Park et al.26,27 demonstrated that adhesion, proliferation, migration, and differentiation of mesenchymal stem cells as well as osteoblasts and osteoclasts were maximally induced on 15-nm nanotubes. Moreover, from the clinical study, it was suggested that 30-90 nm average roughness might be most proper to achieve a stable soft tissue sealing around transmucosal abutments.25 Although the surface roughness of specimens used in this study have not been properly calculated, considering SEN observation results, they seemed to be outside the range of critical scale which could have changed the cellular response as suggested above, and therefore, fibroblast adhesion and growth were not relatively dependent on the surface roughness. In practice, similar trends were seen in Meredith et al.'s work.28 They compared biocompatibilities of standard, electropolished titanium, Ti-6Al-7Nb, and stainless steel. All samples in their experiment showed a general trend of increase in cell number with time, with the exception of cells grown on titanium alloy.
A quantification of cell adhesion seems to be one of the most plausible ways to evaluate the surface of biomaterials such as dental implants. To date, several quantitative adhesion assays have been developed. They are primarily based on measurement of the force or energy required to detach a cell from the substrate using the mechanical forces such as centrifugal force or liquid flow shear stress.29-31 Although valuable data for cell attachment can be obtained from these methods, most of the assays need specialized devices or time-consuming and technique-sensitive processes. In contrast, the suggested ultrasonic wave application technique is a very simple and easy method being commonly used in the laboratory and allows for the comparison of adhesion strengths of cells to biomaterials with various surface conditions within a short time and with equal amount of ultrasonic wave application, as shown in the present results. However, the universal application of this method for quantifying cell adhesion is still questionable because only one type of intensity can be applied with the equipment used in this study for detaching the cells. Therefore, it is necessary to perform further studies in order to confirm the measurement of exact strength applied to each specimen and application of this technique to a variety of materials with diverse surface modifications for extended periods of time.
Another technique for quantification of cell adhesion was categorized as a non-mechanical method. The total focal adhesion contact area was measured using the microscope32 or the focal adhesion-related protein such as vinculin was identified using immunocytochemistry.8,33 Several gain- and loss-of-function studies showed that vinculin plays a crucial role in stabilizing focal adhesion, and its amount of expression might be indicative of cell motility on a substrate.34-37 Therefore, it has been considered as the most appropriate protein to target for the development of an immunocytochemistry-based cell adhesion assay method.8 Consistent with previous reports, findings of this study showed that both mRNA and protein expression levels of vinculin in fibroblasts grown on given substrates increased with time after seeding, and this changing pattern was collinear with the amount of remaining cells after mechanical force application. Therefore, vinculin expression may be related with the strengthening of cell attachment to the surface and it can be one of the suitable proteins for evaluating cell adhesion strength. In addition to vinculin, similar trends were seen in both the mRNA and protein expressions of paxillin. Paxillin is known as a downstream component of FAK signaling located at the cytoskeletal-membrane interface and contributes to stabilization of focal adhesion with vinculin and FAK.38,39 Therefore, paxillin may also be involved in increasing focal adhesion strength. Although tensin and FAK, the other components of FAK signaling, also showed changes in expression in some specimens after 5 days of culturing, their involvement in increasing focal adhesion strength is still questionable because there is a difference in timing between the changing patterns of gene expressions and results of mechanical force application.
The findings of this study revealed that various surface conditions and materials used have little influence on HGF survival and attachment. The strength of the adhesion of cells to the abutment surfaces was closely related to the amount of time for which they were in contact with the surface. Therefore clinically, precautions are necessary during the early phase of dental implant therapy when the attachment of cells to the abutment surfaces is weak. In addition, focal adhesion linker proteins, especially vinculin and paxillin, could contribute to good adhesion of fibroblasts to the abutment surfaces, and hence the measurement of expression of vinculin and paxillin is one of the easiest and useful methods for evaluating biocompatibility of materials.
These results might suggest that the strength of adhesion of fibroblasts to transmucosal abutment surfaces and expressions of FALPs increases with time. Therefore, thorough consideration is required during the early phase of dental implant therapy when the adhesion of fibroblasts to the abutment surfaces is incomplete.
Figures and Tables
Fig. 1
Photographs of surface modified titanium and ceramic discs with or without HGF taken using SEM: (A, F, K) machined titanium surface (MT), (B, G, I) polished titanium surface (PT), (C, H, M) titanium coated with titanium nitride (CT), (D, I, N) machined ceramic surface (MC), (E, J, O) polished ceramic surface (PC), (C, H, M). HGFs were cultured for 1 day (F-J) and for 5 days (K-O), respectively. Magnification: ×200.
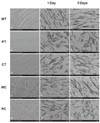
Fig. 2
Growth of HGFs on five discs with different modified surfaces was assessed using the MTT assay. Results are shown as the mean number of cells from four wells ±SD. Ctrl: control, MT: machined titanium surface, PT: polished titanium surface, CT: titanium coated with titanium nitride, MC: machined ceramic surface, PC: polished ceramic surface.
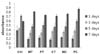
Fig. 3
Comparison of cell survival rates of HGFs cultured on five discs with different modified surfaces for 1, 3, 5 and 7 days before and after mechanical force application using ultrasonic waves. Results are shown as the mean number of cells from four wells ±SD. Ctrl: control, MT: machined titanium surface, PT: polished titanium surface, CT: titanium coated with titanium nitride, MC: machined ceramic surface, PC: polished ceramic surface.
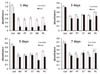
Fig. 4
mRNA expressions of focal adhesion linker proteins of cultured HGFs grown on five discs with different modified surfaces assessed by semi-quantitative RT-PCR assay. (A) Comparison of mRNA expression levels of focal adhesion linker proteins obtained from 5-day and 7-day cultured HGFs grown on five discs with different modified surfaces. (B) Changes in mRNA expression levels of focal adhesion linker proteins with time. Ctrl: control, MT: machined titanium surface, PT: polished titanium surface, CT: titanium coated with titanium nitride, MC: machined ceramic surface, PC: polished ceramic surface.
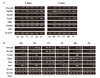
Fig. 5
Changes in expression of paxillin and vinculin proteins in HGFs cultured for 1 day and 5 days respectively on five discs with different modified surfaces assessed using western blot. Ctrl: control, MT: machined titanium surface, PT: polished titanium surface, CT: titanium coated with titanium nitride, MC: machined ceramic surface, PC: polished ceramic surface.
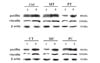
References
1. Palma-Carrió C, Balaguer-Martínez J, Peñarrocha-Oltra D, Peñarrocha-Diago M. Irritative and sensory disturbances in oral implantology. Literature review. Med Oral Patol Oral Cir Bucal. 2011; 16:e1043–e1046.
2. Berglundh T, Lindhe J, Ericsson I, Marinello CP, Liljenberg B, Thomsen P. The soft tissue barrier at implants and teeth. Clin Oral Implants Res. 1991; 2:81–90.
3. Listgarten MA, Buser D, Steinemann SG, Donath K, Lang NP, Weber HP. Light and transmission electron microscopy of the intact interfaces between non-submerged titanium-coated epoxy resin implants and bone or gingiva. J Dent Res. 1992; 71:364–371.
4. Berglundh T, Lindhe J. Dimension of the peri-implant mucosa: Biological width revisited. J Clin Periodontol. 1996; 23:971–973.
5. Ambrose EJ. The movements of fibrocytes. Exp Cell Res. 1961; 8:54–73.
6. Burridge K, Fath K, Kelly T, Nuckolls G, Turner C. Focal adhesions: transmembrane junctions between the extracellular matrix and the cytoskeleton. Annu Rev Cell Biol. 1988; 4:487–525.
7. Hynes RO. Integrins: bidirectional, allosteric signaling machines. Cell. 2002; 110:673–687.
8. Owen GR, Meredith DO, ap Gwynn I, Richards RG. Focal adhesion quantification - a new assay of material biocompatibility? Review. Eur Cell Mater. 2005; 9:85–96.
9. An N, Rausch-fan X, Wieland M, Matejka M, Andrukhov O, Schedle A. Initial attachment, subsequent cell proliferation/viability and gene expression of epithelial cells related to attachment and wound healing in response to different titanium surfaces. Dent Mater. 2012; 28:1207–1214.
10. Anselme K, Bigerelle M, Noel B, Dufresne E, Judas D, Iost A, Hardouin P. Qualitative and quantitative study of human osteoblast adhesion on materials with various surface roughnesses. J Biomed Mater Res. 2000; 49:155–166.
11. Boyan BD, Lohmann CH, Dean DD, Sylvia VL, Cochran DL, Schwartz Z. Mechanisms involved in osteoblast response to implant surface morphology. Annual Rev Mater Res. 2001; 31:357–371.
12. Garrod DR. Cell to cell and cell to matrix adhesion. BMJ. 1993; 306:703–705.
13. Giancotti FG, Ruoslahti E. Integrin signaling. Science. 1999; 285:1028–1032.
14. Räisänen L, Könönen M, Juhanoja J, Varpavaara P, Hautaniemi J, Kivilahti J, Hormia M. Expression of cell adhesion complexes in epithelial cells seeded on biomaterial surfaces. J Biomed Mater Res. 2000; 49:79–87.
15. Biggs MJ, Richards RG, Gadegaard N, Wilkinson CD, Dalby MJ. Regulation of implant surface cell adhesion: characterization and quantification of S-phase primary osteoblast adhesions on biomimetic nanoscale substrates. J Orthop Res. 2007; 25:273–282.
16. Arvidson K, Fartash B, Hilliges M, Köndell PA. Histological characteristics of peri-implant mucosa around Brånemark and single-crystal sapphire implants. Clin Oral Implants Res. 1996; 7:1–10.
17. Abrahamsson I, Berglundh T, Glantz PO, Lindhe J. The mucosal attachment at different abutments. An experimental study in dogs. J Clin Periodontol. 1998; 25:721–727.
18. Craig RG, Hanks CT. Cytotoxicity of experimental casting alloys evaluated by cell culture tests. J Dent Res. 1990; 69:1539–1542.
19. Li D, Ferguson SJ, Beutler T, Cochran DL, Sittig C, Hirt HP, Buser D. Biomechanical comparison of the sandblasted and acid-etched and the machined and acid-etched titanium surface for dental implants. J Biomed Mater Res. 2002; 60:325–332.
20. Martin JY, Schwartz Z, Hummert TW, Schraub DM, Simpson J, Lankford J Jr, Dean DD, Cochran DL, Boyan BD. Effect of titanium surface roughness on proliferation, differentiation, and protein synthesis of human osteoblast-like cells (MG63). J Biomed Mater Res. 1995; 29:389–401.
21. Kieswetter K, Schwartz Z, Hummert TW, Cochran DL, Simpson J, Dean DD, Boyan BD. Surface roughness modulates the local production of growth factors and cytokines by osteoblast-like MG-63 cells. J Biomed Mater Res. 1996; 32:55–63.
22. Batzer R, Liu Y, Cochran DL, Szmuckler-Moncler S, Dean DD, Boyan BD, Schwartz Z. Prostaglandins mediate the effects of titanium surface roughness on MG63 osteoblast-like cells and alter cell responsiveness to 1 alpha,25-(OH)2D3. J Biomed Mater Res. 1998; 41:489–496.
23. Lossdörfer S, Schwartz Z, Wang L, Lohmann CH, Turner JD, Wieland M, Cochran DL, Boyan BD. Microrough implant surface topographies increase osteogenesis by reducing osteoclast formation and activity. J Biomed Mater Res A. 2004; 70:361–369.
24. Könönen M, Hormia M, Kivilahti J, Hautaniemi J, Thesleff I. Effect of surface processing on the attachment, orientation, and proliferation of human gingival fibroblasts on titanium. J Biomed Mater Res. 1992; 26:1325–1341.
25. Grössner-Schreiber B, Herzog M, Hedderich J, Dück A, Hannig M, Griepentrog M. Focal adhesion contact formation by fibroblasts cultured on surface-modified dental implants: an in vitro study. Clin Oral Implants Res. 2006; 17:736–745.
26. Park J, Bauer S, von der Mark K, Schmuki P. Nanosize and vitality: TiO2 nanotube diameter directs cell fate. Nano Lett. 2007; 7:1686–1691.
27. Park J, Bauer S, Schlegel KA, Neukam FW, von der Mark K, Schmuki P. TiO2 nanotube surfaces: 15 nm-an optimal length scale of surface topography for cell adhesion and differentiation. Small. 2009; 5:666–671.
28. Meredith DO, Eschbach L, Wood MA, Riehle MO, Curtis AS, Richards RG. Human fibroblast reactions to standard and electropolished titanium and Ti-6Al-7Nb, and electropolished stainless steel. J Biomed Mater Res A. 2005; 75:541–555.
29. Derhami K, Wolfaardt JF, Wennerberg A, Scott PG. Quantifying the adherence of fibroblasts to titanium and its enhancement by substrate-attached material. J Biomed Mater Res. 2000; 52:315–322.
30. Groessner-Schreiber B, Neubert A, Müller WD, Hopp M, Griepentrog M, Lange KP. Fibroblast growth on surface-modified dental implants: an in vitro study. J Biomed Mater Res A. 2003; 64:591–599.
31. Reyes CD, García AJ. A centrifugation cell adhesion assay for high-throughput screening of biomaterial surfaces. J Biomed Mater Res A. 2003; 67:328–333.
32. Hunter A, Archer CW, Walker PS, Blunn GW. Attachment and proliferation of osteoblasts and fibroblasts on biomaterials for orthopaedic use. Biomaterials. 1995; 16:287–295.
33. Richards RG, Stiffanic M, Owen GR, Riehle M, Ap Gwynn I, Curtis AS. Immunogold labelling of fibroblast focal adhesion sites visualised in fixed material using scanning electron microscopy, and living, using internal reflection microscopy. Cell Biol Int. 2001; 25:1237–1249.
34. Grover A, Rosentraus MJ, Sterman B, Snook ME, Adamson ED. An adhesion-defective variant of F9 embryonal carcinoma cells fails to differentiate into visceral endoderm. Dev Biol. 1987; 120:1–11.
35. Rodríguez Fernández JL, Geiger B, Salomon D, Ben-Ze'ev A. Overexpression of vinculin suppresses cell motility in BALB/c 3T3 cells. Cell Motil Cytoskeleton. 1992; 22:127–134.
36. Rodríguez Fernández JL, Geiger B, Salomon D, Ben-Ze'ev A. Suppression of vinculin expression by antisense transfection confers changes in cell morphology, motility, and anchorage-dependent growth of 3T3 cells. J Cell Biol. 1993; 122:1285–1294.
37. Alenghat FJ, Fabry B, Tsai KY, Goldmann WH, Ingber DE. Analysis of cell mechanics in single vinculin-deficient cells using a magnetic tweezer. Biochem Biophys Res Commun. 2000; 277:93–99.
38. Turner CE. Paxillin and focal adhesion signalling. Nat Cell Biol. 2000; 2:e231–e236.
39. Brown MC, Turner CE. Paxillin: adapting to change. Physiol Rev. 2004; 84:1315–1339.