This article has been corrected. See "Erratum - Influence of various metal oxides on mechanical and physical properties of heat-cured polymethyl methacrylate denture base resins" in Volume 5 on page 502.
Abstract
PURPOSE
To evaluate the effect of various metal oxides on impact strength (IS), fracture toughness (FT), water sorption (WSP) and solubility (WSL) of heat-cured acrylic resin.
MATERIALS AND METHODS
Fifty acrylic resin specimens were fabricated for each test and divided into five groups. Group 1 was the control group and Group 2, 3, 4 and 5 (test groups) included a mixture of 1% TiO2 and 1% ZrO2, 2% Al2O3, 2% TiO2, and 2% ZrO2 by volume, respectively. Rectangular unnotched specimens (50 mm × 6.0 mm × 4.0 mm) were fabricated and droptower impact testing machine was used to determine IS. For FT, compact test specimens were fabricated and tests were done with a universal testing machine with a cross-head speed of 5 mm/min. For WSP and WSL, discshaped specimens were fabricated and tests were performed in accordance to ISO 1567. ANOVA and Kruskal-Wallis tests were used for statistical analyses.
RESULTS
IS and FT values were significantly higher and WSP and WSL values were significantly lower in test groups than in control group (P<.05). Group 5 had significantly higher IS and FT values and significantly lower WSP values than other groups (P<.05) and provided 40% and 30% increase in IS and FT, respectively, compared to control group. Significantly lower WSL values were detected for Group 2 and 5 (P<.05).
Acrylic resin polymethyl methacrylate (PMMA) is the most extensively used material in fabrication of dentures. Although it is very popular, this material is still insufficient in fulfilling the ideal mechanical requirements of such appliances.1 Clinicians still encounter fracture of this material due to low resistance to impact, flexural, or fatigue stresses.2 In order to prevent fracture of the dentures, the thickness of acrylic resin in susceptible regions, such as the palatal midline, and the mandibular lingual and labial frenal attachments has been increased.3 In addition, improvement on mechanical properties of denture base materials were tried to be achieved either by adding a polyfunctional crosslinking agent such as polyethylene glycol dimethacrylate4 or by incorporating a rubber phase,5 metal oxides, metal wire6,7 or fibres.8 The reinforcement of polymers used in dentistry with metal-composite systems has been a prime interest. Sehajpal and Sood9 added various amounts of powdered Cu, Ag and Al into the PMMA resin and reported increased compressive strength but decreased tensile strength. Zuccari et al.,10 evaluated the improvement of the mechanical properties of PMMA, polyethyl methacrylate (PEMA) and poly isobutyl methacrylate (PIMA) resin matrices by reinforcing with oxides of Al, Mg, Zr and pulverized E-glass particles. They suggested that 2% admixtures by volume in PMMA resin matrix resulted in better mechanical properties.
PMMA absorbs relatively small amounts of water when placed in an aqueous environment due to the polarity of the resin molecules.11 By forcing the polymer chains apart, water absorbed into the material by a diffusion mechanism causes a slight expansion which may compensate the shrinkage that occurs during fabrication of heat-cured denture bases.12 The absorbed water in the polymer networks can cause physical changes such as plasticization and softening, leading to a decrease in transverse strength and fatigue limit.11-13 Water sorption also has detrimental effect on the color stability.14 The amount of water sorption should not exceed 32 µg/mm3 for heat-cured or chemical-cured materials according to the International Standards of Organization (ISO) specification No. 1567.15
Denture base acrylic resins are soluble in a variety of solvents, but the solubility in the fluids encountered in the oral cavity is not a favorable and desired property.11 The solubility of the acrylic resins represents the amount of water-soluble ingredients, unreacted monomers, plasticizers and initiators that leached out during 7 days the specimen was immersed in water.16 The solubility of specimen is measured by the observed loss of weight of the acrylic. As has been presented by the ISO specification No. 1567, the solubility value should not exceed 1.6 µg/mm3 for heat-cured and 8.0 µg/mm3 for chemical-cured materials.15
Since only the limited amount of data regarding the effect of metal oxides on heat-cured PMMA are available in the literature, the purpose of this study was to investigate the influence of different types and amounts of the metal oxides on some mechanical and physical properties of heatcured PMMA.
Impact strength, fracture toughness, water sorption and solubility tests of modified and unmodified heat-cured acrylic resin were carried out in this study. For each test, 50 acrylic resin specimens were fabricated and divided into 5 groups with 10 specimens each. One group was the control group and others were the test groups, which were formed according to the types and amounts of metal oxides incorporated into the acrylic resin (Table 1).
The oxide powders used were aluminium oxide (Al2O3) (Alcao, Deutschland GmBH, Germany), titanium oxide (TiO2) (Aldrich Chemie GmBH, Germany), and zirconium oxide (ZrO2) (Merck, Wasserfrei, Darmstadt, Germany). The oxide powders were heated at 800℃ for two hours in a muffle furnace for dehydration and then they were treated with a metal alloy primer (MP II, GC Corp. Tokyo, Japan) and allowed to dry for 5 minutes in a thermostatic oven at 62℃.10 The particle size of metal oxide powders was determined with a Master Sizer E (Ver.1.2b, Malvern Instruments Ltd. Malvern, UK). The average size of metal oxides Al2O3, TiO2, ZrO2 were 12.4, 9.6, and 8.6 µm, respectively. These values were much smaller compared to the particle size (121.2 µm) of heat-cured PMMA resin. For test groups, TiO2 and ZrO2 powders were admixed to the acrylic resin powders at 1% and 2% by volume, while Al2O3 powders were admixed to the acrylic resin powders only at 2% by volume prior to polymerization.
A pink heat-cured PMMA (Meliodent; Heraeus Kulzer Ltd, Berkshire, Germany) was used as denture base acrylic resin in the present study (Fig. 1). The acrylic resin was supplied as a powder and liquid. The specimens were fabricated according to the manufacturer's instructions. After the predetermined width and thickness of all specimens were confirmed using a digital caliper (Model SC-6; Mitutoyo Sul Americana Ltda, Suzano, SP, Brazil), the final finishing was performed using 600 grit silicone carbide sandpaper (Navyug Sales Corporation, Delhi, India).
For impact strength testing, 50 unnotched acrylic resin specimens (50.0 mm × 6.0 mm × 4.0 mm) were fabricated from a stainless steel mold. The dimensions of the specimens were selected as suggested in the former studies.17,18 Before testing, the specimens were allowed to remain in distilled water at 37℃ for a week. The low velocity impact strength tests were performed using a drop tower impact test machine (Dynatup 9250 HV, Instron Corp. Bucks, UK). Impact strength (I) was calculated as kJ/m2 with the following formula:
where E is the energy (which breaks the test specimen), W is the specimen width, and T is the specimen thickness.
For fracture toughness testing, 50 acrylic resin compact test specimens were fabricated from a stainless steel mold (Fig. 2) and tests were carried out as described in the study of Yilmaz and Korkmaz.19 After all specimens were stored in distilled water at 37℃ for 24 hours, using a razor blade, a precrack was formed at the end of the slots of samples (Fig. 3). Fracture toughness tests were performed in a universal testing machine (Zwick Z020, GmbH and Co, Ulm-Einsingen, Germany) with a cross-head speed of 5 mm/min, and peak load to fracture was recorded. The recorded data were used to determine the fracture toughness (KIc) in MPa·m1/2 according to the following formula:19
where pc is the maximum load (kN) prior to crack advance, b is specimen thickness (cm), w is the width of the specimen (cm) and
where a is crack length (crack starter notch plus fatigue crack, cm).
For water sorption and solubility testing, a stainless steel mold was used to prepare specimen disks in accordance to International Organization for Standardization (ISO) No.1567,15 with the exception of the dimensions of test specimens. A hundred acrylic resin specimens, 50 for water sorption and 50 for solubility test, were prepared with the nominal dimensions of 50 ± 1 mm in diameter and 2.5 ± 0.05 mm in thickness. The top and bottom surfaces of disk-shaped test specimens were flatten by grinding with 600 grit silicon carbide sandpaper and dried in dessicator containing thorougly dry anhydrous silica gel (freshly dries at 130℃) at 37 ± 2℃ for 24 hours. Specimens were stood in ambient temperature for 1 hour. The previously described drying cycle was repeated until the weight loss of each disk was not more than 0.2 mg in any 24 hour period. After the specimens reached constant mass (W1), they were immersed in distilled water at 37℃ ± 1 for 7 days. After that time, each specimen was removed from the water, wiped with a clean dry hand-towel until free from visible moisture, and waved in the air for 15 seconds. Then each specimen was weighed 1 minute after removal from the water with a precision of 0.2 mg. This mass was recorded as W2. After this weighing, the discs were reconditioned to constant mass in the dessicator described previously. This reconditioned mass was recorded as W3.15
The values for water sorption (Wsp) and solubility (Wsl) were obtained in g/cm3 for each of the specimens were calculated using the following formulas:
where W1 is the conditioned mass in g prior to immersion in water, W2 is the mass of the specimen in g after immersion in water; W3 is the reconditioned mass of the specimen in g after drying and V is the volume of the specimen cm3. After completing the calculation, the values were converted to µg/mm3.
Shapiro-Wilk test was used to examine whether the distribution of variables were normal or not. If the obtained P value is greater than .05, the distribution of the variable is normal.
ANOVA test was used for statistical analyses of impact strength and fracture toughness test results. Since the distribution of variable was normal, Tukey HSD statistical test was used to determine which groups had statistically significant differences. P<.05 was considered statistically significant.
Kruskal-Wallis test was used for statistical analyses of water sorption and solubility test results. Since the distribution of variable was not normal, Mann-Whitney U statistical test was utilized to determine which groups had statistically significant differences. P<.05 was considered statistically significant.
The statistical summary and statistically significant differences for IS, FT, WSP and WSL tests were shown in Table 2.
IS and FT values were significantly higher in Group 2, 3, 4 and 5 than in control group (P<.05). Group 5 demonstrated significantly higher IS (6.5536 ± 0.069 kJ/m2) and FT (1.6638 ± 0.003 MPa·m1/2) mean values compared to other groups (P<.05) and resulted in 40% and 30% increase in IS and FT respectively, compared to control group (IS = 4.6042 ± 0.066 kJ/m2, FT = 1.2743 ± 0.002 MPa·m1/2). However, Tukey HSD statistical test showed no significant difference between Group 2 and Group 4 in terms of IS (P>.05).
WSP and WSL values were significantly lower in Groups 2, 3, 4 and 5 than in control group (P<.05). Group 5 showed significantly lower mean WSP value (17.5 µg/mm3) and Group 2 and 5 yielded significantly lower WSL value (1.4 µg/mm3) compared to other groups (P<.05). Mann-Whitney U statistical test presented no significant differences between Group 2 and 5, Group 3 and 4 in terms of WSL and between Group 3 and 4 in terms of WSP (P>.05).
Acrylic resin is the most commonly used material for the construction of denture due to its esthetics, ease of manipulation and low cost. However, this material is not ideal because of its relatively low mechanical strength, which can cause the fracture of denture. The present study investigated the effects of some metal oxides, such as TiO2, Al2O3 and ZrO2 that are abundantly available, on impact strength, fracture toughness, water sorption and solubility of acrylic resin.
To date, polymers have been reinforced by adding materials such as metal oxides,9 metal strengtheners,17 carbongraphite fibers,20,21 aramid fiber,22 ultra-high molecular weight polyethylene fiber (UHMWPE),23 and glass fibers.11,24 The size, shape and distribution of filler paricles in the polymer matrix and strong adhesion at the interface play major role on the mechanical properties of particulatefilled polymer composites.25 The size of metal oxide particles should be small for proper processing.26 The particle sizes of metal oxides (7-13 µm) used in this study are much smaller than that of powder resin particles (121.2 µm). Therefore, the metal oxide particles will fill the interstitial of polymer particles to give a heterogenous mixture and will not force the displacement of the segments of polymer chain.26 Furthermore, the percentage of metal oxides should be kept low to ensure that they will be embedded in resin. The advantage of fillers used in the current study is the lower density, thus the light weight of acrylic resin is maintained. All groups in this study contained only one type of metal oxide, except Group 2. Mixing two metal oxides (ZrO2 and TiO2) having different particle sizes can lead to have more particles per unit volume, which may provide additional improvement of mechanical and physical properties of acrylic denture bases by inhibiting the spaces between oxide particles.
The inorganic reinforcing particulate fillers usually display high surface energy because of the hydrophilic ionic nature. However, the hydrophobic polymer does not wet or interact interfacially with the filler due to the difference in surface energies. It is essential to modify the filler surface to improve surface wetting and adhesion between the filler and matrix.10 In the current study, prior to admixing with the polymer beads, the fillers were treated with a metal bonding agent (MP II) to create strong interphase between structural matrix and the resin matrix.
In the present study, the reinforcement of polymer with metal oxides caused significantly higher impact strength and fracture toughness values compared to control group. Since fracture toughness (KIC) and impact strength (I) are the most important properties of an acrylic resin denture base, it is desirable to produce a KIC and I values as high as possible.27 Group 1 had fracture toughness value of 1.27 MPa·m1/2 and impact strength value of 4.68 kJ/m2. Among test groups, Group 5 showed the highest impact strength (6.55 kJ/m2) and fracture toughness (1.66 MPa·m1/2) values, meaning 30% increase in fracture toughness and 40% increase in impact strength. This improvement might be related to the smallest particle dimension and proper distribution of ZrO2, which could lead to an increase in crack length during the process of fracture. Increasing crack length can result in the increase in energy absorption before fracture.28
Improving mechanical properties of acrylic denture bases by incorporating particulate fillers has been studied by few researchers. The results of the present study are consistent with those reported in the study of Zuccari et al.,10 in which Al2O3, MgO and ZrO2 powders were applied to reinforce the self-cured acrylic resins used to fabricate provisional fixed restorations. Among oxide particles, 2% ZrO2 exhibited the greatest improvements in modulus of elasticity, transverse strength, toughness and hardness. Schricker et al.,29 evaluated the effect of methacrylated hyperbranched polymers (MA-HB) prepared in different concentrations on the fracture toughness. They found that the fracture toughness values were 1.45 and 1.81 for control and 1% MA-HB added groups, respectively. On the other hand, Elshereksi et al.,30 investigated the influence of 10 wt% BaTiO3 filler incorporation on the fracture toughness of PMMA. They presented decreased KIC values for the test group compared to the control group. This was explained by slightly weak adhesion between BaTiO3 and polymer matrix and by anisotropic particle orientation throughout composite material. Also, they did not report any bonding agent used to treat BaTiO3 in order to improve bonding between filler and polymer matrix. Schulze et al.,31 concluded that an increase in filler fraction does not necessarily lead to an increase in strength. Because higher filler fractions create more defects that weaken the materials. Low filler fractions used in this study might have caused higher strength values obtained in the test groups. In order to be able to compare the results of similar studies, the loading conditions and specimen geometry, such as the dimensions of the sample and the presence and configuration of notches should be the same.3 Since no studies exist in the literature that utilized the same impact strength testing machine and relevant loading conditions, misleading conclusion may result when comparing the impact strength result direclty with other studies.
While improving the mechanical properties of a denture base acrylic resin, it is also of great importance not to compromise its physical properties such as water sorption and solubility. Acrylic resin dentures are notable for their tendency to absorb water, which causes corresponding dimensional change and the hydrolytic degredation of polymers.32,33 Solubility represents the mass of soluble materials from polymers. Initiators, activators, plasticizers and residual monomers are the soluble materials found in denture base acrylic resins.11 For denture base polymers, the value of water sorption should be less or equal to 32 µg/mm3 as recommended by ISO 1567.15 Previous studies reported values of 10-25 µg/mm3 for the water sorption of different types of acrylic resins.34,35 In this study, the highest mean water sorption value, 21.3 µg/mm3, were obtained for Group 1, which was very below the recommended value and in agreement with the results of other studies.34,35 When Group 1 and other groups were compared, it was observed that metal oxide incorporation lead to a decrease in water sorption values. Group 5 showed the lowest mean water sorption value of 17.5 µg/mm3, which was significantly lower than the other groups. As a result of solubility test, the mean values of test groups complied with the requirements of the ISO 156715 (not more than 1.6 µg/mm3 for heat-polymerizing acrylic resins). Group 2, 3, 4, and 5 had lower solubility values compared to Group 1. The lowest mean solubility values were determined for Group 2 and 5, which were significantly lower than other groups. The reasons why metal oxide added groups exhibited significantly lower water sorption and solubility values than control group can be explained in several ways. During the polymerization process of acrylic resins, porosity or microvoids can occur among polymer chains. A high level of porosity or microvoids has been shown to facilitate fluid transport into and out of polymer by serving as sites for molecules to be sequestered and leading to enhanced solvent uptake and elution.36 The metal oxides used in this study are insoluble in water 37 and could reduce the overall volume of the absorbing polymer.36 The second factor could be the metal bonding agent which was used to treat metal oxides. The metal primer, based on thiophosphoric acid methacrylate, had similar chemical nature with polymer matrix. Thus, the metal oxide-resin interface provided by metal bonding agent could lead to a reduction in the amount of water that reached to the inner layers of the polymer matrix. Vallittu38 compared two different silane compound agents in improving adhesion between fibres and acrylic denture base material. He reported that treating fibre with silane compound decreased the voids between polymer matrix and fibres, which led to less water sorption.
Within the limits of this study, the following conclusion can be drawn. Since Al2O3,TiO2 and ZrO2 fillers resulted in significant increase in impact strength and fracture toughness and significant decrease in water sorption and solubility, modification of heat-cured acrylic resins with certain amounts of metal oxides, especially with ZrO2, may be useful in preventing denture fractures and undesirable physical changes resulting from oral fluids clinically.
References
1. Darbar UR, Huggett R, Harrison A. Denture fracture-a survey. Br Dent J. 1994; 176:342–345. PMID: 8024869.


2. Jagger DC, Harrison A, Jandt KD. The reinforcement of dentures. J Oral Rehabil. 1999; 26:185–194. PMID: 10194725.


3. Meng TR Jr, Latta MA. Physical properties of four acrylic denture base resins. J Contemp Dent Pract. 2005; 6:93–100. PMID: 16299611.
4. Kanie T, Fujii K, Arikawa H, Inoue K. Flexural properties and impact strength of denture base polymer reinforced with woven glass fibers. Dent Mater. 2000; 16:150–158. PMID: 11203537.


5. Knott NJ. The durability of acrylic complete denture bases in practice. Quintessence Int. 1989; 20:341–343. PMID: 2756090.
6. Ruffino AR. Effect of steel strengtheners on fracture resistance of the acrylic resin complete denture base. J Prosthet Dent. 1985; 54:75–78. PMID: 3894643.


7. Teraoka F, Nakagawa M, Takahashi J. Adaptation of acrylic dentures reinforced with metal wire. J Oral Rehabil. 2001; 28:937–942. PMID: 11737565.


8. Goldberg AJ, Burstone CJ. The use of continuous fiber reinforcement in dentistry. Dent Mater. 1992; 8:197–202. PMID: 1387855.


9. Sehajpal SB, Sood VK. Effect of metal fillers on some physical properties of acrylic resin. J Prosthet Dent. 1989; 61:746–751. PMID: 2724170.


10. Zuccari AG, Oshida Y, Moore BK. Reinforcement of acrylic resins for provisional fixed restorations. Part I: Mechanical properties. Biomed Mater Eng. 1997; 7:327–343. PMID: 9457383.
11. Miettinen VM, Vallittu PK, Docent DT. Water sorption and solubility of glass fiber-reinforced denture polymethyl methacrylate resin. J Prosthet Dent. 1997; 77:531–534. PMID: 9151274.


12. Phoenix RD. Denture base resins: technical considerations and processing techniques. In : Anusavice KJ, editor. Phillip's Science of Dental Materials. 11th ed. St. Louis: Saunders WB Company;2003. p. 721–756.
13. Fujii K. Fatigue properties of acrylic denture base resins. Dent Mater J. 1989; 8:243–259. PMID: 2490598.


14. O'Brien WJ. Dental materials and their selection. 3rd ed. IL: Quinttessence Pub Co;2002. p. 1–11.
15. ISO 1567:1999. Dentistry-Denture base polymers. Geneva: International Organization for Standardization;1999.
16. Cucci AL, Vergani CE, Giampaolo ET, Afonso MC. Water sorption, solubility, and bond strength of two autopolymerizing acrylic resins and one heat-polymerizing acrylic resin. J Prosthet Dent. 1998; 80:434–438. PMID: 9791790.


17. Vallittu PK, Lassila VP. Reinforcement of acrylic resin denture base material with metal or fibre strengtheners. J Oral Rehabil. 1992; 19:225–230. PMID: 1500965.


18. Caycik S, Jagger RG. The effect of cross-linking chain length on mechanical properties of a dough-molded poly (methylmethacrylate) resin. Dent Mater. 1992; 8:153–157. PMID: 1521701.
19. Yilmaz C, Korkmaz T. The reinforcement effect of nano and microfillers on fracture toughness of two provisional resin materials. Mater Des. 2007; 28:2063–2070.


20. Manley TR, Bowman AJ, Cook M. Denture bases reinforced with carbon fibers. Br Dent J. 1979; 146:25–28. PMID: 282911.
21. DeBoer J, Vermilyea SG, Brady RE. The effect of carbon fiber orientation on the fatigue resistance and bending properties of two denture resins. J Prosthet Dent. 1984; 51:119–121. PMID: 6583382.


22. Mullarky RH. Aramid fiber reinforcement of acrylic appliances. J Clin Orthod. 1985; 19:655–658. PMID: 3864791.
23. Braden M, Davy KW, Parker S, Ladizesky NH, Ward IM. Denture base poly(methyl methacrylate) reinforced with ultra-thin modulus polyethylene fibers. Br Dent J. 1988; 164:109–113. PMID: 3279982.


24. Solnit GS. The effect of methyl methacrylate reinforcement with silane-treated and untreated glass fibers. J Prosthet Dent. 1991; 66:310–314. PMID: 1800725.


25. Unal H, Mimaroglu A. Influence of filler addition on the mechanical properties of Nylon-6 Polymer. J Reinf Plast Compos. 2004; 23:461–469.


26. Korkmaz T, Doğan A, Usanmaz A. Dynamic mechanical analysis of provisional resin materials reinforced by metal oxides. Biomed Mater Eng. 2005; 15:179–188. PMID: 15911998.
27. Alhareb AO, Ahmad ZA. Effect of Al2O3/ZrO2 reinforcement on the mechanical properties of PMMA denture base. J Reinf Plast Compos. 2011; 30:86–93.
28. Gupta N, Brar BS, Woldesenbet E. Effect of filler addition on the compressive and impact properties of glass fibre reinforced epoxy. Bull Mater Sci. 2001; 24:219–223.


29. Schricker SR, Hamza T, Jere D, Dotrong M, Wee A. Effect of metharcylated hyperbranched polymers on the fracture properties of denture base materials. J Macromol Sci Part A Pure Appl Chem. 2006; 43:205–212.
30. Elshereksi NW, Mohamed SH, Arifin A, Mohd Isak ZA. Effect of filler incorporation on the fracture toughness properties of denture base poly(methyl methacrylate). J Phys Sci. 2009; 20:1–12.
31. Schulze KA, Zaman AA, Söderholm KJ. Effect of filler fraction on strength, viscosity and porosity of experimental compomer materials. J Dent. 2003; 31:373–382. PMID: 12878020.


32. Cheng YY, Chow TW, Ladizesky NH. Denture base resin reinforced with high-performance polyethylene fiber: Water sorption and dimensional changes during immersion. J Macromol Sci Phys. 1993; 32:433–481.


33. Wong DM, Cheng LY, Chow TW, Clark RK. Effect of processing method on the dimensional accuracy and water sorption of acrylic resin dentures. J Prosthet Dent. 1999; 81:300–304. PMID: 10050118.


34. Barsby MJ. A denture base resin with low water absorption. J Dent. 1992; 20:240–244. PMID: 1430515.


35. Arima T, Murata H, Hamada T. The effects of cross-linking agents on the water sorption and solubility characteristics of denture base resin. J Oral Rehabil. 1996; 23:476–480. PMID: 8814563.


36. Ferracane JL. Hygroscopic and hydrolytic effects in dental polymer networks. Dent Mater. 2006; 22:211–222. PMID: 16087225.


37. Panyayong W, Oshida Y, Andres CJ, Barco TM, Brown DT, Hovijitra S. Reinforcement of acrylic resins for provisional fixed restorations. Part III: effects of addition of titania and zirconia mixtures on some mechanical and physical properties. Biomed Mater Eng. 2002; 12:353–366. PMID: 12652030.
38. Vallittu PK. Comparison of two different silane compounds used for improving adhesion between fibres and acrylic denture base material. J Oral Rehabil. 1993; 20:533–539. PMID: 10412475.


Fig. 1
Three types of acrylic resin specimens used in this study. (A) Test specimen for fracture toughness, (B) Test specimen for impact strength, (C) Test specimen for water sorption and solubility.
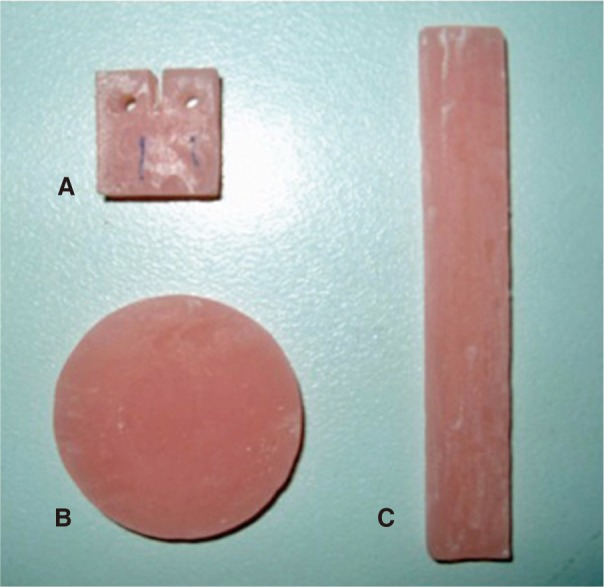
Fig. 2
Compact test specimen used for fracture toughness testing. (w = 12 mm, b = 4 mm, a = 2.4 mm) (Reprinted from Materials & Design, Yilmaz C, Korkmaz T, The reinforcement effect of nano and microfillers on fracture toughness of two provisional resin materials, 28(7): 2063-70, 2007, with permission from Elsevier).
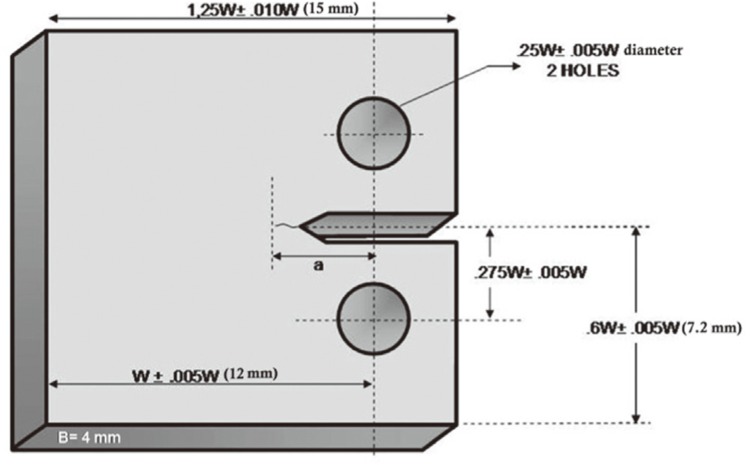
Fig. 3
Precrack formation of a specimen before fracture toughness testing. (Reprinted from Materials & Design, Yilmaz C, Korkmaz T, The reinforcement effect of nano and microfillers on fracture toughness of two provisional resin materials, 28(7), 2063-70, 2007, with permission from Elsevier).
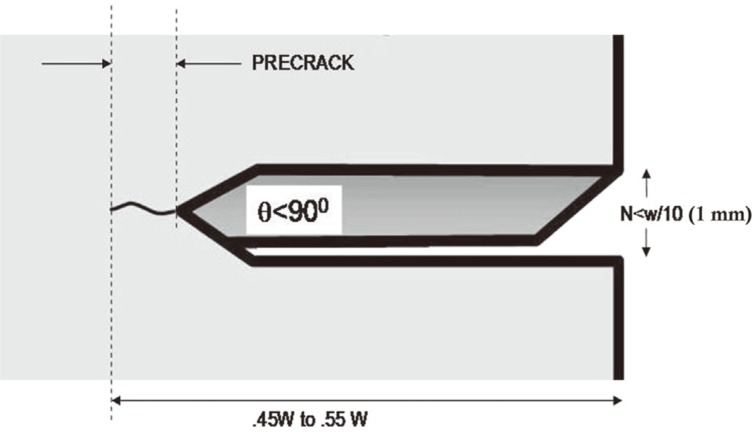