Abstract
PURPOSE
This study aimed to identify the effects of the sintering conditions of dental zirconia on the grain size and translucency.
MATERIALS AND METHODS
Ten specimens of each of two commercial brands of zirconia (Lava and KaVo) were made and sintered under five different conditions. Microwave sintering (MS) and conventional sintering (CS) methods were used to fabricate zirconia specimens. The dwelling time was 20 minutes for MS and 20 minutes, 2, 10, and 40 hours for CS. The density and the grain size of the sintered zirconia blocks were measured. Total transmission measurements were taken using a spectrophotometer. Two-way analysis of variance model was used for the analysis and performed at a type-one error rate of 0.05.
RESULTS
There was no significant difference in density between brands and sintering conditions. The mean grain size increased according to sintering conditions as follows: MS-20 min, CS-20 min, CS-2 hr, CS-10 hr, and CS-40 hr for both brands. The mean grain size ranged from 347-1,512 nm for Lava and 373-1,481 nm for KaVo. The mean light transmittance values of Lava and KaVo were 28.39-34.48% and 28.09-30.50%, respectively.
All-ceramic restorations are preferred by both dentists and patients because of their good esthetic properties compared to metal restorations, namely an inherent translucency similar to that of natural teeth.1 However, all-ceramic restorations have limited indications and are difficult to be applied to a challenging prosthesis that requires physical strength because of their intrinsic weaknesses.2
In recent years, 3 mol% stabilized tetragonal zirconia polycrystalline (3Y-TZP) ceramics have gained favor as restorative materials because of their excellent mechanical properties3-5; they have high strength and toughness, good biocompatibility,6-8 and relatively good esthetic properties.3
Esthetically fabricated all-ceramic prostheses should have a color and translucency comparable to those of natural teeth.9,10 Of these two factors, the translucency of the core is the most important determinant of the esthetic properties of all-ceramic restorations.1 However, the zirconia core is less translucent than other dental all-ceramic materials such as glass-infiltrated ceramic, which is known for its good esthetic properties.11-13 Therefore, by increasing the translucency of the zirconia core, the esthetic characteristics of dental restoration can be improved.
Fundamentally, translucency is highly dependent on light scattering.14 Pores inside materials greatly influence light scattering; low porosity is required to improve the translucency of ceramics.15,16 In addition, the amount of light absorbed, reflected, and transmitted depends on the amount of crystal and chemical components inside the core materials, the size of the particles compared with the wavelength of incident light.17
Translucency of zirconia is related to the amount and type of additives,18 the sintering temperature,19 the atmospheric conditions during the sintering process,20 and the heating methods.21 In particular, the final temperature of the sintering process and the heating method used are direct determinants of the density, porosity, and grain size of zirconia.22
Several studies have reported that small mean grain size is associated with enhanced translucency, mechanical properties and a delay in low temperature degradation (LTD) caused by inhibition of the transformation from the tetragonal phase to the monoclinic phase.18,21,23 Microwave sintering (MS) is more efficient than conventional sintering (CS) at reducing the size of zirconia grains.22 MS has advantages of improved productivity and energy saving due to volumetric heating and rapid sintering over CS, and yields zirconia grains with a uniform microstructure.24,25 For ceramics, improved densification and inhibition of the grain growth may reduce the sintering time by enhancing the mechanical properties and enabling sintering at lower temperatures.18,26 However, the translucency of dental zirconia in relation to MS has not been extensively studied.
The aim of this study was to investigate the pattern of changes in the density, grain size, and translucency of dental zirconia according to various sintering conditions (time and method), as well as the effects of the sintering conditions on the translucency of dental zirconia. Our hypothesis in this study was that a change in the sintering conditions would change the translucency of the zirconia core.
Commercially available Lava frame zirconia (3M ESPE, St. Paul, MN, USA) and KaVo Everest ZS-blanks (KaVo Dental GmbH, Biberach, Germany) dental zirconia blocks were used in this study. The sintering temperatures for MS and CS were set according to the instructions of the manufacturers. The heating rate was 50℃/min for MS and near 10℃/min for CS. The dwelling time was 20 minutes for MS and 20 min 2, 10, or 40 hr for CS (four conditions). The detailed sintering conditions are shown in Table 1. After sintering, 10 specimens [10 mm (width) × 10 mm (length) × 1 mm (thickness)] were made for each of the two types of zirconia (Lava and KaVo) and for each of the five sintering conditions using a diamond saw (ISOMET, Buehler Ltd., Lake Bluff, IL, USA). The blocks were cut in a container filled with water using low speed diamond saw. The thickness of the core was adjusted to 1 mm (± 0.05 mm) using electronic Vernier calipers (Mitutoyo, Kanagawa, Japan). The core was then polished using 1,200-grit wet sandpaper (Buehler Ltd., Lake Bluff, IL, USA).
The density of the sintered specimens was measured using the Archimedes method in water, following the ISO 18754 standard.27
To measure the translucency of the dental zirconia cores, light transmittance was measured using a spectrophotometer. (SpectraMagic CM-3500d, KONICA MINOLTA, Osaka, Japan). Measurements were performed at wavelengths ranging from 400 to 700 nm at 10 nm intervals. Measurements were repeated three times per specimen.
Transmittance (T) was determined using the following equation:
where L specimen is the specimen luminance and L source is the source luminance.
To measure the size and microstructure of the grain, the blocks were polished using a 1 µm diamond paste. All specimens were thermally etched at 1,400℃ for 10 min. Afterwards, all specimens were cleaned ultrasonically in acetone for 10 min and then dried for 24 hr. The specimens were then investigated via scanning electron microscopy (JSM-6360, JEOL Techniques, Tokyo, Japan). The grain size was calculated using the average linear intercept method of ASTM Standard E112.28 For each specimen, 25 line segments were taken into account.29 This process was performed last to prevent interference from the effects of the polishing that was done to obtain SEM images to measure light transmittance.
Descriptive statistics were expressed as mean and standard deviation (SD). To analysis relationship with 2 factors, brands and sintering methods, the 2-way analysis of variance (ANOVA) model was used to compare the density, grain size, and light transmittance values (%) of Y-TZP according to different sintering conditions and zirconia brands. As assumption of normality tested by the Shapiro-Wilk's test was satisfied in the distribution of density and grain size, the (parametric) 2-way ANOVA was applied. For density all 2 factors were insignificant. For grain size, the interaction term was insignificant in the full factorial model, therefore the main-effect model was used. However the assumption of normality was not satisfied in that of light transmittance and nonparametric version of 2-way ANOVA using rank transformed value was applied. The interaction term was significant with full factorial model of light transmittance. As assumption of equal variance was not satisfied in grain size and transmittance, the Tamhane's T2 method was applied for the post-hoc comparison. All statistical tests were performed at a type-one error rate of 0.05. The statistical software package SPSS version 12.0 (SPSS V12.0K, SPSS Inc., Chicago, IL, USA) was used.
The density of the Lava zirconia core ranged from 6.05 to 6.07, and that of the KaVo zirconia core from 6.05 to 6.06. The density of Lava was highest (6.07) at MS-20 minutes and the lowest at CS-40 hours, whereas the density of KaVo was highest at both MS-20 minutes and CS-2 hours. Under all conditions, the density of each specimen was close to the full density. The density of Lava did not differ significantly from that of KaVo. Furthermore, there was no significant difference in density according to sintering conditions.
The changes in the mean light transmittance according to the brands and sintering conditions are summarized in Table 2. The mean light transmittance values of Lava and KaVo were 28.39-34.48% and 28.09-30.50%, respectively. Both Lava and KaVo showed the highest mean light transmittance values of 34.48% and 30.50%, respectively, at MS-20 minutes, and the lowest mean light transmittance values of 28.39% and 28.09%, respectively, at CS-40 hours. Statistical analyses revealed that sintering conditions interacted with brand.
The mean grain size ranged from 347-1,512 nm for Lava and 373-1, 481 nm for KaVo according to the sintering conditions. Both Lava and KaVo had the smallest mean grain sizes of 347 and 373 nm, respectively, at MS-20 minutes, and the largest mean grain sizes of 1,512 and 1,481 nm, respectively, at CS-40 hours (Table 3). The longer the sintering time, the larger the resulting grain. This finding was confirmed by SEM images (Fig. 1).
Because we found that the grain size (P<.001) and translucency (P<.001) of dental zirconia changed according to changes in sintering conditions, we adopted our null hypothesis.
The translucency of dental ceramics can be studied in three ways: through direct transmission, which can be assessed by measuring the light that reaches a detector; total transmission, which can be assessed by measuring both the light that reaches the detector and that which passes the ceramic and is scattered; and indirect measurements via spectral reflectance.14 In this study, we measured translucency by assessing the total transmission.
Light scattering has been widely studied owing to its important relation to the esthetics of all-ceramic restorations affected by color and translucency.14,30 Light scattering relies on the grain size and wavelength of incident light. If the grain and wavelength of incident light are in a similar range, the amount of light scattering increases with grain size and lowers the light transmittance. If grain size is much larger than the wavelength of incident light, the amount of light scattering becomes inversely proportional to the grain size, and independent of the wavelength of incident light.18,31,32
In our study, light transmittance decreased with an increase in grain size not only for MS and CS, which have grain sizes in the range of visible light, but also for the larger-grained CS10 and 40. Our results are consistent with Hayashi et al.33 in which an increase in grain size led to higher light transmittance when experimenting with a wavelength of 300-1,700 nm and 0.82-1.43 µm particles. Also, O et al.34 reported that the measurement of light transmittance with a grain size of 0.8-2 µm using a 1,000 nm wavelength, which is consistent with our results.
Thus far, factors having a negative effect on translucency are known to be grain boundaries, pores, second-phase of component, and light scattering from rough surfaces.33 However, recent studies reported that polycrystalline materials have more light scattering in micropores than at grain boundaries despite their birefringence property.33,35,36 Even so, when grain size falls below micron units, the effect of light scattering from pores within materials is significantly reduced.18,20,33,35
The theoretical full density of the TZP material was 6.10 g/cm3, and ISO 13356 states that it is appropriate for the density of zirconia to exceed 6.00 g/cm3.37 In this study, the density of all the specimens ranged from 6.06 to 6.07 g/cm3. There were no statistically significant differences among specimens. Hayashi et al.33 reported no relationship between pores and light scattering for specimens having a submicron-sized grain and high density (> 99%), which is consistent with our results. The slight difference of final sintering temperatures caused by different manufacturer's instructions (1,450 and 1,500℃) did not affect the densities of the zirconia specimens.
Tekeli and Erdogan38 reported that a high sintering temperature and a long dwelling time increased the grain size and thus, the number of micropores, resulting in a material with reduced mechanical properties. In our study, CS resulted in a difference in grain sizes based on the dwelling time (P<.001). In particular, the products of both manufacturers showed minute grain boundary cracks at CS-40 hours (Fig. 1E). It has been reported that although a grain boundary crack is a type of defect that decreases light transmittance, the effect is very weak.33,35,36 Therefore, this suggests that an excessive dwell time decreases the light transmittance mainly by inducing grain growth.
In contrast, Hjerppe et al.39 reported that a short sintering time for dental zirconia decreased the grain size, though this finding was not statistically significant, and had no influence on the mechanical properties. In our study, however, there was a significant difference in the heating rates of MS and CS, which indicates a great difference in overall entire sintering time and mean grain size (Table 3).16
We found that a short sintering time yielded a small grain with high light transmittance while, in contrast, large grains formed by long sintering times had low light transmittance (Table 1 and 2). We found that the MS method, which can be completed in a short time, yielded zirconia with a small grain size and high light transmittance. These results should improve the low light transmittance of dental zirconia compared to other glass-infiltrated ceramics.11,12 In addition, considering the clinical significance of zirconia, the physical properties and marginal fitness of the coping should be studied in relation to the sintering method used, grain size, and light transmittance.
Within the limitations of this study, we concluded that sintering condition (time) affected the grain size of dental zirconia: the shorter the sintering time, the smaller the grain size and this decrease of grain size resulted in an increase of the light transmittance values of the final dental zirconia.
Figures and Tables
Fig. 1
SEM images (Original magnification ×10,000) of the polished and thermally etched surface of Lava specimens sintered for various times using different approaches: A: MS-20 minutes, B: CS-20 minutes, C: CS-2 hours, D: CS-10 hours, and E: CS-40 hours. The black arrows indicate grain boundary cracks.
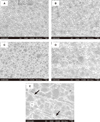
References
1. Kelly JR, Nishimura I, Campbell SD. Ceramics in dentistry: historical roots and current perspectives. J Prosthet Dent. 1996; 75:18–32.
2. Fischer H, Weber M, Marx R. Lifetime prediction of all-ceramic bridges by computational methods. J Dent Res. 2003; 82:238–242.
3. Conrad HJ, Seong WJ, Pesun IJ. Current ceramic materials and systems with clinical recommendations: a systematic review. J Prosthet Dent. 2007; 98:389–404.
4. Tinschert J, Natt G, Mautsch W, Augthun M, Spiekermann H. Fracture resistance of lithium disilicate-, alumina-, and zirconia-based three-unit fixed partial dentures: a laboratory study. Int J Prosthodont. 2001; 14:231–238.
5. Chen YM, Smales RJ, Yip KH, Sung WJ. Translucency and biaxial flexural strength of four ceramic core materials. Dent Mater. 2008; 24:1506–1511.
6. Akagawa Y, Hosokawa R, Sato Y, Kamayama K. Comparison between freestanding and tooth-connected partially stabilized zirconia implants after two years' function in monkeys: a clinical and histologic study. J Prosthet Dent. 1998; 80:551–558.
7. Ichikawa Y, Akagawa Y, Nikai H, Tsuru H. Tissue compatibility and stability of a new zirconia ceramic in vivo. J Prosthet Dent. 1992; 68:322–326.
8. Scarano A, Di Carlo F, Quaranta M, Piattelli A. Bone response to zirconia ceramic implants: an experimental study in rabbits. J Oral Implantol. 2003; 29:8–12.
9. Rosenstiel SF, Land MF, Fujimoto J. Contemporary Fixed Prosthodontics. 4th ed. St. Louis: Mosby;2006. p. 262. p. 643.
10. Yu B, Ahn JS, Lee YK. Measurement of translucency of tooth enamel and dentin. Acta Odontol Scand. 2009; 67:57–64.
11. Heffernan MJ, Aquilino SA, Diaz-Arnold AM, Haselton DR, Stanford CM, Vargas MA. Relative translucency of six all-ceramic systems. Part I: core materials. J Prosthet Dent. 2002; 88:4–9.
12. Heffernan MJ, Aquilino SA, Diaz-Arnold AM, Haselton DR, Stanford CM, Vargas MA. Relative translucency of six all-ceramic systems. Part II: core and veneer materials. J Prosthet Dent. 2002; 88:10–15.
13. Vichi A, Louca C, Corciolani G, Ferrari M. Color related to ceramic and zirconia restorations: a review. Dent Mater. 2011; 27:97–108.
14. Brodbelt RH, O'Brien WJ, Fan PL. Translucency of dental porcelains. J Dent Res. 1980; 59:70–75.
15. Peelen JGJ, Metselaar R. Light-scattering by pores in polycrystalline materials: transmission properties of alumina. J Appl Phys. 1974; 45:216–220.
16. Zhang HB, Kim BN, Morita K, Yoshida H, Lim JH, Hiraga K. Optimization of high-pressure sintering of transparent zirconia with nano-sized grains. J Alloys Compd. 2010; 508:196–199.
17. Clarke FJ. McLean JW, editor. Measurement of color of human teeth. Proceedings of the First International Symposium on Ceramics. 1983. Chicago: Quintessence;p. 441–490.
18. Casolco SR, Xu J, Garay JE. Transparent/translucent polycrystalline nanostructured yttria stabilized zirconia with varying colors. Scr Mater. 2008; 58:516–519.
19. Jiang L, Liao Y, Wan Q, Li W. Effects of sintering temperature and particle size on the translucency of zirconium dioxide dental ceramic. J Mater Sci Mater Med. 2011; 22:2429–2435.
20. Anselmi-Tamburini U, Woolman JN, Munir ZA. Transparent nanometric cubic and tetragonal zirconia obtained by high-pressure pulsed electric current sintering. Adv Funct Mater. 2007; 17:3267–3273.
21. Yang D, Raj R, Conrad H. Enhanced sintering rate of Zirconia (3Y-TZP) through the effect of a weak dc electric field on grain growth. J Am Ceram Soc. 2010; 93:2935–2937.
22. Janney MA, Calhoun CL, Kimrey HD. Microwave sintering of solid oxide fuel cell materials: I, Zirconia-8 mol% Yttria. J Am Ceram Soc. 1992; 75:341–346.
23. Li JF, Watanabe R. Phase Transformation in Y2O3-Partially-Stabilized ZrO2 Polycrystals of Various Grain Sizes during Low-Temperature Aging in Water. J Am Ceram Soc. 1998; 81:2687–2691.
24. Ebadzadeh T, Valefi M. Microwave-assisted sintering of zircon. J Alloys Compd. 2008; 448:246–249.
25. Cheng J, Agrawal D, Zhang Y, Roy R. Microwave sintering of transparent alumina. Mater Lett. 2002; 56:587–592.
26. Luo J, Adak S, Stevens R. Microstructure evolution and grain growth in the sintering of 3Y-TZP ceramics. J Mater Sci. 1998; 33:5301–5309.
27. ISO 18754 - Fine ceramics-advanced ceramics, advanced technical ceramics-Determination of density and apparent porosity. Geneva; Switzerland: ISO;2008.
28. ASTM. Standard test method for determining average grain size E112-96. Part 301. 2003. p. 243–266.
29. Mendelson MI. Average Grain Size in Polycrystalline Ceramics. J Am Ceram Soc. 1969; 52:443–446.
30. Cook WD, McAree DC. Optical properties of esthetic restorative materials and natural dentition. J Biomed Mater Res. 1985; 19:469–488.
31. Lee YK. Influence of scattering/absorption characteristics on the color of resin composites. Dent Mater. 2007; 23:124–131.
32. O'Brien WJ, Johnston WM, Fanian F. Double-layer color effects in porcelain systems. J Dent Res. 1985; 64:940–943.
33. Hayashi K, Kobayashi O, Toyoda S, Morinaga K. Transmission optical properties of polycrystalline alumina with submicron grains. Mater Trans JIM. 1991; 32:1024–1029.
34. O YT, Koo JB, Hong KJ, Park JS, Shin DC. Effect of grain size on transmittance and mechanical strength of sintered alumina. Mat Sci Eng A. 2004; 374:191–195.
35. Apetz R, van Bruggen MPB. Transparent alumina: A light scattering model. J Am Ceram Soc. 2003; 86:480–486.
36. Alaniz JE, Perez-Gutierrez FG, Aguilar G, Garay JE. Optical properties of transparent nanocrystalline yttria stabilized zirconia. Opt Mater. 2009; 32:62–68.
37. ISO 13356 - Implants for surgery-Ceramic materials based on yttria-stabilized tetragonal zirconia (Y-TZP). Geneva; Switzerland: ISO;2008.
38. Tekeli S, Erdogan M. A quantitative assessment of cavities in 3 mol% yttria-stabilized tetragonal zirconia specimens containing various grain size. Ceram Int. 2002; 28:785–789.
39. Hjerppe J, Vallittu PK, Fröberg K, Lassila LV. Effect of sintering time on biaxial strength of zirconium dioxide. Dent Mater. 2009; 25:166–171.