Abstract
PURPOSE
This in vitro study intended to investigate the mechanical and thermal characteristics of Valplast, and of polymethyl methacrylate denture base resin in which different esthetic fibers (E-glass, nylon 6 or nylon 6.6) were added.
MATERIALS AND METHODS
Five groups were formed: control (PMMA), PMMA-E glass, PMMA-nylon 6, PMMA-nylon 6.6 and Valplast resin. For the transverse strength test the specimens were prepared in accordance with ANSI/ADA specification No.12, and for the impact test ASTM D-256 standard were used. With the intent to evaluate the properties of transverse strength, the three-point bending (n=7) test instrument (Lloyd NK5, Lloyd Instruments Ltd, Fareham Hampshire, UK) was used at 5 mm/min. A Dynatup 9250 HV (Instron, UK) device was employed for the impact strength (n=7). All of the resin samples were tested by using thermo-mechanical analysis (Shimadzu TMA 50, Shimadzu, Japan). The data were analyzed by Kruskal-Wallis and Tukey tests for pairwise comparisons of the groups at the 0.05 level of significance.
RESULTS
In all mechanical tests, the highest values were observed in Valplast group (transverse strength: 117.22 ± 37.80 MPa, maximum deflection: 27.55 ± 1.48 mm, impact strength: 0.76 ± 0.03 kN). Upon examining the thermo-mechanical analysis data, it was seen that the E value of the control sample was 8.08 MPa, higher than that of the all other samples.
Polymethy methacrylate (PMMA) resin, widely used in denture base, has lots of advantageous properties: it is easy to apply and to repair, its low cost, acceptability by most of the patients, stability in the oral cavity, and aesthetical properties. Its mechanical feature, however, is far from the ideal because it has weak flexural and impact strength and low fatigue resistance. These often lead to denture failure during chewing or when it is dropped.1-4 There has been ongoing effort to enhance the strength and fatigue resistance of PMMA. These efforts can be listed as: (1) reinforcement of denture materials with the addition of filling materials,1,5,6 (2) altering the chemistry of PMMA resin materials and (3) manufacturing alternative denture base materials.5,7
Fiber-reinforced resin seemed to be the best option. With choices ranging from carbon, aramid, rayon, E-glass and nylon fibers as resin strengtheners, favourable result on impact and transverse strength properties of PMMA resin have been reported and discussed in detail.5,6,8-11 There are a number of the studies focusing on the effect of glass fibers on the mechanical qualities of PMMA resin.3,5,6,10 Reinforcement of the resin has been achieved in clinical environment with good strength results, and glass fibers were regarded to be esthetically fit for this purpose.4-6,11-13 Nevertheless, there is progressing search for alternative resin materials with better mechanical strength than PMMA.7,14 Alternative polymer systems to PMMA, such as polyamide, epoxy, polystyrene, or vinyl acrylic resins, have also been tried. However, the desired denture base material has not been developed yet.4,15
Polyamides, known as 'nylon' are thermoplastic polymers produced by condensation between a diamine and a dibasic acid.7 In a number of studies polyamide was used as a denture base polymer in 1950s.16 It was reported that nylon was not found to be doing well for denture construction owing to discoloration tendency and high water absorption. Hence, nylon denture was not suitable for common use. It was especially used in exceptional cases like repeated denture fracture and for patients with tissue allergies against acrylic denture base or denture fractures.14
Denture base resins could be subjected to varying degrees of temperatures in the mouth during foods intake, cleaning, or particularly during smoothing and polishing processes at the manufacturing stage. Therefore, it is significant to know the mechanical properties and the stability of the resin material composition against heat.17 For the denture base resin materials, widely performed techniques have been differential scanning calorimetry (DSC), dynamic mechanical thermal analysis (DMTA), and thermal mechanical analysis (TMA).18 Among these methods, TMA has been known to be the most important method because it yields reproducible data for the vitreous transition temperature, coefficient of thermal elongation, and modulus of elasticity.18-20
In the present study, a mechanical and thermal properties comparison were made between the polyamide based denture base material (Valplast International Corp, USA), as an alternative to poly methyl methacrylate resin, and this PMMA resin system was modified by adding aesthetic fibers including E-glass, Nylon 6, or Nylon 6.6 fibers.
Acrylic resin (Meliodent; Bayer Dental, Newbury, Berkshire, UK), three different fibers [E-glass (KCR4, Nylon 6 (N6), Nylon 6.6 (N6.6), Table 1] and polyamide-based resin system (Valplast, International Corp, USA) were used. The transverse and impact strength tests were performed as mechanical tests. Five groups, (α=0.05, β=0.10, 1- β=0.90) each consisting of 7 specimens (P=.91947), were formed for each test. The groups formed were as follows: PMMA, three PMMA groups including KCR4, N6 or N6.6, and Valplast.
Transverse strength was determined by three-point bending test method. The specimens for this test were prepared in 65 × 10 × 2.5 mm dimensions in accordance with ANSI/ADA specification No.12. In preparing the test specimens, stainless steel mould was used. The standard wax specimens were obtained by pouring the melted pink plate waxes (Modelling Wax, De Trey S.A, Bios Colombes, France) into the isolated mold. The prepared wax specimens were taken into the mold and these wax specimens were removed by melting them and then the molds were made ready for acrylic molding.
Fiber material was prepared as follows: each of the KCR4, N6, and N6.6 fibers were trimmed in 4 mm length and weighed on the assay balance (Precisia ×B 220, Precisia Instruments Ltd. Switzerland). The fiber specimens were added to the polymer powder randomly in a ratio of 3% by weight.
Acrylic resin specimens were prepared with or without fiber at a powder/liquid ratio of 2.34 g/mL in accordance with the manufacturer's instructions. After 1 minute mixing and following a dough time of 6 min, the acrylic resin was molded into the moulds which had been prepared in advance. After the molding procedure was executed, 5 minutes pressure was applied onto the molds under a hydraulic press (Rucker PHI, Birmingham, UK). The molds were then transferred into a 70℃ water-bath for 1 h, and after that, the polymerization action was performed immediately by keeping them in the boiling water for 30 minutes. Heated cartridges were used for the polymerization of Valplast specimens. The cartridges were heated under vacuum in a heating furnace by incubation for 11 minutes at 287℃, and the specimens were injected into the cartridges by using an injector (Valplast, International Corp, USA). When polymerization is completed, the flasks were left to cool at ambient temperature prior to being opened. Deflasked specimens were polished by hand with a 600 grit water-proof silicon carbide paper under tap water.
Transverse strength was determined by the three-point flexure test in a computer-aided universal test device (Lloyd NK5, Lloyd Instruments Ltd., Fareham Hampshire, UK). The specimens were placed on supports, located by 50 mm intervals, and a 5 mm/min cracking speed was applied. This amount of speed is enough to crack the specimens. The degree of bending before the cracking occurred was recorded (Nexygen, Lloyd Instruments Ltd., Fareham, Hampshire, UK).
Transverse strength values were calculated in N/mm2 (MPa) by using the following formula:
where Ts = Transverse Strength (N/mm2); F = Applied Load (N); l = Distance between supports (mm); b = Specimen width (mm); d = specimen thickness (mm).
The specimens for the impact strength test were obtained in accordance with ASTM D256 standards, and prepared in metal moulds in 55 × 10 × 10 mm dimensions. The specimens had a V-shaped notch in the middle, with 2 mm width and 2.54 mm depth. The specimens were also siliconized (Imprex putty, Arma, Turkey). Polymerization procedures were kept identical, as described previously. When polymerization is completed, the flasks were left to cool at ambient temperature prior to being opened. Deflasked specimens were manually polished as described above. Impact strength was determined in a Charpy type impact test device (Dynatup 9250HV, Instron, UK). A drop weight of 7.3 kg (which was equivalent to 12 J energy) was applied to the specimens on the unnotched side.
TMA test samples were prepared at 20 × 5 × 1 mm dimensions as recommended by the manufacturer of the instrument (Shimadzu TMA 50, Shimadzu, Japan). Curves demonstrating changes in thermal-mechanical properties with temperature were obtained by using the values produced by the TMA instrument. The stress-strain curves were obtained under nitrogen atmosphere at a heating rate of 10℃min-1 and at a loading rate of 5 gmin-1. LogE, Strain-temperature curves were obtained through reading every one of the temperature points, and modulus of elasticity (E) values for each sample on the recorded graphs. These curves were used in determining the thermomechanical parameters, modulus of elasticity (E), coefficient of thermal elongation (α) and vitreous transition (Tg) values.
After the data collection, mean value and standard deviation were assessed with a SPSS statistical software program (14.0 version, SPSS Inc., Chicogo, IL, USA). Data were then analyzed with Kruskal-Wallis and Tukey tests for pairwise comparisons of the groups at the 0.05 level of significance.
After application of the transverse test to all the specimen groups, the fractured surfaces were examined under SEM. In the specimens whose acrylic part was broken and where the fiber showed continuity, the exposed parts of the fiber were cut, and 2 mm sections were obtained by using a water-cooled sectioning device (Buehler Isomed, Low Speed Saw, USA). The section specimens were then made conductive under the vacuum of 4 × 10-2 mbar in a Polaron SC7620 Sputter Coater device by coating with Au-Pd for 15 seconds (3 Angstrom coating per second, in total 45 Angstrom). The interface of fiber-resin was visualized by surface scanning electron microscope (LEO 440 Scanning Electron Microscope, UK).
The results obtained from the mechanical tests were presented in Table 2. When the values of transverse strength, maximum deflection and impact strength were compared, the differences between the groups were found to be significant. In all the tests we carried out, the highest values were observed in the Valplast group. But, in the groups with fibre addition, though higher transvers and impact strength values were observed in the group with glass fibre addition, the value of maximum deflection was found to be the highest in the group with nylon 6.6 fibre addition. But, there are no statistical differences.
TMA analysis data are given in Table 3. Valplast material group had the highest mechanical resistance values (Fig. 1). Modulus of elasticity (E) values was obtained from the curvatures of the original linear sections of the stress-strain curves. Likewise, the coefficient of thermal elongation values (α) were acquired from the original linear sections of the temperature-strain curves (Fig. 2). And the vitreous transition temperature (Tg) values were obtained using the instant drop regions of the temperature-logE curves (Fig. 3). single climax observed in the thermo-mechanical curves, and thermo-mechanical parameters (Table 3) of the samples could be taken as an indication that the used fibers were compatible with the PMMA resin.
Structural images of the specimens were obtained by using a surface scanning electron microscope. The assessments in fiber-added specimens were made in terms of fiber-resin connection and fiber distribution (Fig. 4, 5, 6 and 7). It was evident on the images that both the control group (Fig. 4A) and the Valplast group displayed a smoother and more compact structure than other specimen groups (Fig. 4B). In the KCR4 fiber specimens group, it was observed that the glass fibers formed bunch-shaped structures inside the resin matrix together with adhesion of acrylic resin. Some pores could also be seen that might have resulted from disintegrations from the resin matrix (Fig. 5). In the fiber specimens to which N6.6 and N6 were added, no interfacial adhesion with the resin was observed, and some cavities appeared to have formed on the interface with the resin matrix. This observation could indicate that some flexions might have occurred on these fibers before fracturing (Fig. 6 and 7).
For the determination of mechanical properties of denture base resins, the transverse strength, impact strength tests and thermo-mechanical tests are often applied.1,3,5,8,21 These tests have been accepted as the most similar forces to the ones which are naturally applied to the oral denture.21 It has been reported that the mechanical strength in the PMMA composite structure, strengthened with fibers, involved many factors such as the type, distribution, quantity and matrix connection of the fiber.4,22,23 Information available in the literature on the impact of the quantity of fiber, added into the polymer matrix, on the composite structure has been somewhat controversial.2,22,24 In a study, for example, it has been reported that adding glass fiber to the heat-polymerized acrylic resin, in a ratio of 58% by weight, increased the transverse strength by 146% in comparison with that of group reinforced with glass fiber,22 whereas in another study it has been indicated that the addition of 6 mm polyethylene fiber beyond 3%, did not cause any improvement in the impact strength and that fiber concentration above 4% resulted in dry and fragile dough.24
The length of fiber used in the reinforcement might also be important. For example, it has been reported in a study that the addition of short E-glass fiber to the acrylic resin increased the transverse strength of the composite resin.1 In another study it has been shown transverse strength of insignificant difference by adding 2, 4 and 6 mm long fibers.8 Guided by these previous studies, in the present study, the fibers used were prepared in 4 mm lengths and added randomly into the PMMA powder at 3% concentration.
The transverse strength of acrylic resin, formed by adding E-glass, aramid and nylon fibers in 5 mm length and in the ratio of 2% by weight, has been shown to have increased in comparison with the control group.25 In another study it has been reported that the addition of E-glass, rayon, polyester, Nylon 6 and 6.6 fibers into PMMA in the ratio of 3% and in different lengths, increased the transverse strength, but only the nylon 6.6 fiber used in 6 mm length improved the transverse strength.26
After testing the strength of conventional and the polyamide denture base materials, it has been reported that the polyamide based denture base material had the most flexibility and no fracture occurred during the loading process.27 Results of the transverse test performed in the present study indicated Valplast had the highest transverse strength (117.22 ± 37.80 MPa), and no fracture was observed in these specimens. Non-breakability has been reported7,28 but some differences in the data that could result from different test conditions were evidenced. Polyamides are polymers containing amide bands C(O)-NH in their main chain. These show a strong tendency to crystallize, which is additionally strengthened by the formation of hydrogen bonds between the oxygen and nitrogen atoms of two various amide groups. These bonds give the polyamide hardness and stiffness.29
The fiber composition of E-glass includes 55% SiO2, 22% CaO, 15% Al2O3, 6% B2O3, 0,5% MgO, < 1.0% Fe + Na + K (manufacturer's infor mation). It has been expressed that the E-glass fiber had a high transverse strength as it contains high alumina and low alkali and borosilicate. Additionally, as the modulus of elasticity of glass fibers is fairly high, most of the stresses could be received by them without causing any deformation.25,26 In the present study, it was shown that although there was no statistical difference between the fiber-added groups, the highest value (108.54 ± 10.33 MPa) was observed in the KCR4-added group. One of the reasons for this finding might be that glass fiber naturally has high transverse strength and its adhesion (Fig. 5) to PMMA also increases the bending strength of the overall structure.
The lowest transverse strength value (83.36 ± 4.25 MPa) was observed in the N6.6 fiber-added groups. As can be seen in the SEM images (Fig. 7), the N6.6 fibers did not appear to have adhered to the resin matrix, this might result from some residual oil left in the fiber material during its manufacture process. Although N6.6 fiber did not increase transverse strength, it yielded the highest maximum deflexion value (4.10 ± 0.37 mm) among fiber added groups. Our findings appeared to be in good agreement with those of Doğan et al.26
Many researchers have used the impact strength tests in order to evaluate the denture base materialsor to determine the effects of environmental conditions and continuous, trimmed fibers. This test has also been used for the comparison of different denture base resins. The impact strength is not the real property of a material because the dimension of the specimen is dependent on the factors such as the existence and dept of the notches, the loading configuration and the speed of impact. The frenulum areas damaging the integrity of acrylic dentures are the ones where the stress concentration is high. In order to stimulate this, the specimens of impact test are prepared in notches.30 In our study, we also prepared the specimens of impact test according to the Charpy test method.
In the present study, it was observed that the values of maximum impact strength were the highest in the Valplast group (0.76 ± 0.03 kN), and it was much higher than those of the other groups. This could be attributed to the chemical structure properties of Valplast, enabling it to better absorb forces, which is different from those of PMMA. Although the value of impact strength of KCR4-added specimens was not statistically significant, it was higher than the other fiber-added groups. Furthermore, when compared to the control group, the values of impact strength of all fiber-added groups appeared to be statistically significant. In literatures, there have been similar findings.5,27,31
It is expressed that the distribution of fibers inside the matrix might affect the mechanical strength.5 In the SEM images, it can be seen that the distribution of nylon-based fibers inside the resin matrix is more regular when compared with KCR4 group. Obviously there must be a difference between the bending modulus values of bunched- and single fiber.5
Upon examining the thermo-mechanical analysis data, it could be seen that the E value of the control sample 8.08 MPa, higher than that of the all other samples. On the other hand, the control samples produced the lowest α value of only 2.58℃-1. Control group also yielded the lowest stress and strain values, 2.4 MPa and 239, respectively. It could also be seen that Valplast resin specimens yielded the lowest E value, 5.06. This was followed by that of glass fiber added specimens. On the other hand, Valplast resin maintained its structure at 3.2 MPa stress and yielded a strain value of 550. These findings indicate that the Valplast material possessed both harder and flexible properties together when compared to the other groups. High mechanical strength of the Valplast specimens could also mean that they would have much lower fatigue values at the maximum loading conditions. In this study, the vitreous transition temperature values increased upon addition of fiber to PMMA, and the vitreous transition temperature was also found to be satisfactory in all sample groups from a clinical perspective. Here, it would also be worth mentioning that Valplast resin yielded the highest vitreous transition temperature, 130℃.
In this study, the modulus of elasticity of Valplast was found to be lower than those of other sample groups. Here, Valplast probably provided the polyamide based structure with more flexibility. Therefore, it might be possible to suggest that the added fibers reduced the modulus of elasticity values of PMMA, thus improving its elastic properties and that the fibers used were compatible with the structure of the acrylic resin. In a study on how the addition of fiber affects the elastic modules of PMMA, it is reported that the modules of composite structures may vary according to the characteristics of the fibres.32 This finding conforms with the outcomes of our study.
The coefficient of thermal elongation values of the samples prepared in this study were obtained by using the original linear sections of the temperature-strain curves. Alpha values of all of the examined sample groups were found to be higher than those of the control group. Amongst all the fiber-added sample groups, nylon 6 fiber containing specimens yielded the highest α values, and these were followed by the nylon 6.6 fiber containing samples. Glass added specimens on the other hand produced the lowest α values. These results together could indicate that differences observed in the coefficient of thermal elongation values resulted mainly from the differences in the structural propertied of the fibers used. One study found in the literature has reported that different materials could yield similar thermal elongation values but materials and techniques in this study differ significantly from ours.33 To sum up, the Valplast sample group yielded the lowest E value and highest strain value under maximum loading conditions.
Limitations of the present in vitro study must be mentioned. Although Valplast displayed better mechanical properties, it requires relevant clinical investigations.
We can induce the following conclusions by taking the results obtained from the present study:
Valplast denture base resin produced higher transverse strength values than those of the control and the fiber-added groups. This also displayed the highest energy absorption capacity.
Thermo-mechanical test results showed that Valplast and nylon 6.6 added specimens displayed better vitreous transition temperatures. Specimen groups produced higher coefficient of thermal elongation values than control PMMA samples. The modulus of elasticity values of all sample groups were lower than that of the control group, therefore added fibers enhanced the structural elasticity of PMMA.
While some adhesion was observed between the KCR4 fiber and the matrix in terms of the interface connection of fiber/resin matrix, no adhesion seemed to have occurred on the interface between other fibers and the matrix.
Figures and Tables
Fig. 4
A: PMMA specimen without fiber (original magnifications ×100), B: Valplast specimen (original magnifications ×100).
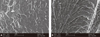
References
1. Marei MK. Reinforcement of denture base resin with glass filler. J Prosthodont. 1999; 8:18–26.
2. Stipho HD. Effect of glass fiber reinforcernent on some mechanical properties of autopolymerizing polymethyl methacrylate. J Prosthet Dent. 1998; 79:580–584.
3. Jagger D, Harrison A, Jagger R, Milward P. The effect of the addition of poly (methyl methacrylate) fibres on some properties of high strength heat-cured acrylic resin denture base material. J Oral Rehabil. 2003; 30:231–235.
4. Jagger DC, Harrison A, Jandt KD. The reinforcement of dentures. J Oral Rehabil. 1999; 26:185–194.
5. Dogan OM, Bolayır G, Keskin S, Doğan A, Bek B, Boztuğ A. The effect of esthetic fibers on impact resistance of a conventional heat-cured denture base resin. Dent Mater J. 2007; 26:232–239.
6. Nakamura M, Takahashi H, Hayakawa I. Reinforcement of denture base resin with short-rod glass fiber. Dent Mater J. 2007; 26:733–738.
7. Uçar Y, Akova T, Aysan I. Mechanical properties of polyamide versus different PMMA denture base materials. J Prosthodont. 2012; 21:173–176.
8. Chen SY, Liang WM, Yen PS. Reinforcement of acrylic denture base resin by incorporation of various fibers. J Biomed Mater Res. 2001; 58:203–208.
9. Schreiber CK. Polymethyl metacrylate reinforced with carbon fibers. Br Dent J. 1971; 130:29–30.
10. Gutteridge DL. The effect ofincluding ultra-high-modulus polyethylene fibre on the impact strength of acrylic resin. Br Dent J. 1988; 164:177–180.
11. Kanie T, Fujii K, Arikawa H, Inoue K. Flexural properties and impact strength of denture base polymer reinforced with woven glass fibers. Dent Mater. 2000; 16:150–158.
12. Solnit GS. The effect of methyl methacrylate reinforcement with silane-treated and untreated glass fibers. J Prosthet Dent. 1991; 66:310–314.
13. Vallittu PK. Curing of a silane coupling agent and its effect on the transverse strength of autopolymerizing polymethylmethacrylate-glass fibre composite. J Oral Rehabil. 1997; 24:124–130.
14. Yunus N, Rashid AA, Azmi LL, Abu-Hassan MI. Some flexural properties of a nylon denture base polymer. J Oral Rehabil. 2005; 32:65–71.
15. Jagger DC, Jagger RG, Allen SM, Harrison A. An investigation into the transverse and impact strength of "high strength" denture base acrylic resins. J Oral Rehabil. 2002; 29:263–267.
16. Phoenix RD, Mansueto MA, Ackerman NA, Jones RE. Evaluation of mechanical and thermal properties of commonly used denture base resins. J Prosthodont. 2004; 13:17–27.
17. Jerolimov V, Jagger RG, Milward PJ. Effect of the curing cycle on acrylic denture base glass transition temperatures. J Dent. 1991; 19:245–248.
18. Huggett R, Brooks SC, Campbell AM, Satguranathan R, Bell GA. Evaluation of analytical techniques for measurement of denture-base acrylic resin glass-transition temperature. Dent Mater. 1990; 6:17–19.
19. Laird JL, Liolios G. Thermal analysis techniques for the rubber laboratory. Am Lab. 1990; 46–50.
20. Boztug A, Coşkun A, Bolayır G, Zengin HB, Özdemir AK. Preparation of compatible blends of poly(methyl metacrylate) used in dentistry with a reactive terpolymer containing maleic anhydride and their thermomechanical characterization. J Appl Polym Sci. 2006; 100:363–367.
21. McCabe JF, Walls AWG. Applied dental materials. 9th ed. Copenhagen: Blackwell Munksgaard;2008.
22. Vallittu PK, Lassila VP, Lappalainen R. Transverse strength and fatigue of denture acrylic-glass fiber composite. Dent Mater. 1994; 10:116–121.
23. Karacaer O, Polat TN, Tezvergil A, Lassila LV, Vallittu PK. The effect of length and concentration of glass fibers on the mechanical properties of an injection- and a compression-molded denture base polymer. J Prosthet Dent. 2003; 90:385–393.
24. Gutteridge DL. Reinforcement of poly (methyl methacrylate) with ultra-high-modulus polyethylene fiber. J Dent. 1992; 20:50–54.
25. John J, Gangadhar SA, Shah I. Flexural strength of heat-polymerized polymethyl methacrylate denture resin reinforced with glass, aramid, or nylon fibers. J Prosthet Dent. 2001; 86:424–427.
26. Doğan OM, Bolayir G, Keskin S, Doğan A, Bek B. The evaluation of some flexural properties of a denture base resin reinforced with various aesthetic fibers. J Mater Sci Mater Med. 2008; 19:2343–2349.
27. Stafford GD, Huggett R, MacGregor AR, Graham J. The use of nylon as a denture-base material. J Dent. 1986; 14:18–22.
28. Takabayashi Y. Characteristics of denture thermoplastic resins for non-metal clasp dentures. Dent Mater J. 2010; 29:353–361.
29. Gruszka I, Lewandowski S, Benko E, Perzyna M. Structure and mechanical properties of polyamid fibres. Fibers Text Eastern Eur. 2005; 13:133–136.
30. Zappini G, Kammann A, Wachter W. Comparison of fracture tests of denture base materials. J Prosthet Dent. 2003; 90:578–585.
31. Chen SY, Liang WM. Effects of fillers on fiber reinforced acrylic denture base resins. Mid Taiwan J Med. 2004; 9:203–210.
32. Clarke RL. Dynamic mechanical thermal analysis of dental polymers I Heat-cured poly(methyl methacrylate)-based materials. Biomaterials. 1989; 10:494–498.
33. Tezvergil A, Lassila LV, Vallittu PK. The effect of fiber orientation on the thermal expansion coefficients of fiber-reinforced composites. Dent Mater. 2003; 19:471–477.