Abstract
PURPOSE
The aims of this pilot study were to introduce implant loading devices designed for animal study and to evaluate the validity of the load transmission ability of the loading devices.
MATERIALS AND METHODS
Implant loading devices were specially designed and fabricated with two implant abutments and cast metal bars, and orthodontic expansion screw. In six Beagles, all premolars were extracted and two implants were placed in each side of the mandibles. The loading device was inserted two weeks after the implant placement. According to the loading protocol, the load was applied to the implants with different time and method,simulating early, progressive, and delayed loading. The implants were clinically evaluated and the loading devices were removed and replaced to the master cast, followed by stress-strain analysis. Descriptive statistics of remained strain (µε) was evaluated after repeating three cycles of the loading device activation. Statistic analysis was performed using nonparametric, independent t-test with 5% significance level and Friedman's test was also used for verification.
In 1960, Brånemark introduced implantation, and it has been regarded as a standard treatment option in partially and fully edentulous patients reporting a success rate of over 95%.1 There are many influencing factors in the success and the failure of dental implant. The loading protocol is one of the most important factors. The loading methods are classified at the time when the first occlusal load is applied. At first, immediate loading is defined as a restoration placed in occlusion with the opposing dentition within 48 hours of implant placement. Secondly, early loading is defined as a restoration in contact with the opposing dentition and placed at least 48 hours after implant placement but no later than 3 months afterward. Delayed/conventional loading is the prosthesis attached in a second procedure that takes place sometime later than the conventional healing period of 3 to 6 months.2
In 1979, immediate loading protocol was first introduced by Ledermann; he reported successful healing in the mandibular anterior region after immediate loading of implants, placed and splinted together with a bar, supporting the overdenture.3 After this, there were many reports about long term success of immediate loading implants. Romanos4 reviewed articles about immediately loaded implants, and reported mean implant survival rate of 94.9%, for duration of 3.58 years on average, with total of 2,118 implants. Moreover, in poor bone quality such as the maxillary arch and the posterior mandible, implant survival rate of immediately loaded implants were reported to be over 90%.5,6
Immediate loading solves the functional and the esthetic problems of patient after implant surgery. It also presents positive effects on bone modeling and remodeling, giving mechanical stimuli to the alveolar bone that contacts to the implant.7 Stress and strain induced through the implants by occlusal force influence bone remodeling between the implant and the adjacent alveolar bone. Frost8 suggested that bone deformation would be repaired by bone remodeling unless it exceeds certain point of force to result in irreversible damage of the bone. This may lead to the assumption that proper occlusal force through the implant can make alveolar bone easy to resist to stress and strain.
In addition to immediate protocols, Binon9 reported that progressive functional loading performed by serial alterations of provisional restoration increase bone mass and bone density between the implant and the surrounding alveolar bone. This protocol was based on the idea that gradual loading or stimulation will allow bone to mature and grow denser, so as to improve the quality of bone.10 Appleton11 also reported that progressively loaded implants showed less crestal bone loss and more increased peri-implant bone density than conventionally loaded implants with 12 months investigation. In the early stage of healing, occlusal load was prevented in order to not apply overload to the immature crestal bone around the implants, and in later stages, progressively increased occlusal load was controlled at the level of stress transmitted to the crestal bone so that the load could be applied in accordance with the load-bearing capacity of the bone.12
Many studies have been performed to compare the effect of different loading protocols on the alveolar bone regeneration.13-16 However, one of their limitations was that they applied uncontrolled force in animals by directly applying occlusal contact with intraoral restoration. Therefore, it would be necessary to develop an implant loading device specially designed to use in the implant loading studies for the control of loading forces in the animal model. This pilot study was performed to investigate the validity of specially designed implant loading device. The aims of this study were to introduce the implant loading devices designed for animal study and to evaluate the validity on the load transmission ability of the loading devices.
The experiment was designed with 4 groups by the loading methods, shown in Table 1. With split mouth design in dogs, a total of 12 hemi-mandibles with 24 implants were allotted to the 4 groups using a random table. All the experimental groups were loaded by different time and method (Fig. 1). One unit load was defined as the amount of load applied to the implant when the expansion screw was rotated ¾ turn, which was 0.3 mm displacement of the loading device. Early loading (EL) group was applied 3-unit loads simultaneously at the time of the loading device placement. Periodic loading (PL) and periodic loading with rotation mobile implantation (Pm) group were applied three cycles of 1-unit load ; at 2 weeks, 4 weeks and 8 weeks after the loading device placement. Rotation mobile implant was placed according to the Ivanoff's study17; the implants were unstable to torque when using a wrench, but remained stable to lateral and axial loading. In this study, it was standardized that after the implant placement following the routine protocol, the implant was rotated two turns clockwise with the wrench, and then positioned back again until the fixture was placed in the crestal bone level. Finally, in delayed loading (DL) group, 3-unit loads were applied simultaneously at 8 weeks after the loading device placement. All the loading devices were removed at 12 weeks.
The Yonsei University Review Committee approved the experimental protocol for Animal Care and Use. Six young adult male Beagle dogs (15 - 25 kg) were used. The dogs were in good oral and general health and had intact maxillary and mandibular dentitions. The dogs were fed a soft diet throughout the experimental period in order to reduce chances of mechanical interferences with healing process during food intake.
All surgical procedures were performed under general anesthesia using atropine (0.05 mg/kg) subcutenously, xylazine (2 mg/kg) and ketamine hydrochloride (10 mg/kg) intravenously. The dogs were placed on a heating pad, intubated, administered 2% enflurane, and monitored with an electrocardiogram. After disinfecting the surgical sites, 2% lidocaine HCl with epinephrine 1:100,000 was administered by infiltration at the surgical sites. During minor experimental procedures, such as prophylaxis, load application through the loading device, the dogs were sedated with an intramuscular injection of 2% xylazine hydrochloride (20 mg/kg).
The dogs fasted 12 hours before the surgery to prevent vomiting. Enrofloxacin (5 mg/kg) was injected subcutaneously, and Ketorolac tromethamine (0.5 mg/kg) was injected intravenously. Under general anesthesia and infiltrative local anesthesia, second, third, and fourth premolars of both sides of the mandible were extracted. A full-thickness flap was raised in the region of four mandibular premolars; the teeth were sectioned bucco-lingually and extracted with forceps. The flaps were repositioned and sutured with 4-0 absorbable sutures. After surgery, amoxicillin/clavulanate (13.75 mg/kg) and clease (1 T/day) was fed for 5 days.
After three months of healing, sedation and anesthesia protocol used earlier was repeated for the implant surgery. After a horizontal, crestal incision was made from the distal region of the first premolar to the mesial region of the first molar, two 3.4 × 8.0 mm implants (FX 3408, Implantium, Dentium, Seoul, Korea), with sandblasted and acid-etched surfaces, were placed with accordance to the manufacturer's instructions, on each side of the mandible of each animal, for a total of 24 implants. The implants were placed 10 mm apart on the crestal bone level (Fig. 2A). The implants were placed rigidly according to the manufacturer's protocol with a torque controller except the rotation mobile implants. After implant placement, pick-up impression was taken on the fixture level with impression copings (DPU 4015HL, Dentium, Seoul, Korea) and self-cured acrylic resin (Pattern resin, GC, Tokyo, Japan) (Fig. 2B). Resin was added incrementally with brush-on technique to prevent polymerization shrinkage that may cause an inaccurate master model.
After the impression taking procedure, healing abutments (HAB 402035L, Dentium, Seoul, Korea) were connected into the implants; the flaps were repositioned, and then sutured. Postoperative medication was performed with same protocol as surgical procedures. After the loading device connection, clinical observations were performed weekly to ensure the loading devices were in good action, and prophylaxis was performed under the sedative procedure mentioned earlier.
Master model was made with impression copings connected to the fixture analogues (DAN 34, Dentium, Seoul, Korea) and the loading device was constructed on the master model as described. Two weeks after implant placement, 2-piece type dual abutments (DAB 4515HL, Dentium, Seoul, Korea) were connected into the fixture with 25 Ncm torque. The loading devices were attached to the abutments using self cured resin modified glass-ionomer cement (Rely-X, 3M ESPE, St. Paul, MN), and then abutment screw hole was filled with cotton pellet and temporary filling material (Caviton, GC Dental Corp., Tokyo, Japan).
Specially designed loading device is consisted of dual abutments, metal bars cemented with abutments, and orthodontic expansion screw attached with metal bars (Fig. 3A). It was designed to apply controlled force, simulating static occlusal force to the implants (Fig. 3B). Upper and lower metal bars was cast with lost wax technique by Ni-Cr alloy (T3, Ticonium Co., CMP Industries Inc., Albany, NY). Prefabricated wax mold was used to standardize the size and shape of the metal bars. Expansion screws (Sectional screw mini, 600-502-30, Dentarum, Scanorto, Denmark) were attached to the center of the upper and the lower arm using alpha laser welding machine (Siro Lasertec, Pforzheim, Germany)(Fig. 3A). Total of 12 loading devices were separately cast and fabricated from their own master cast. This loading device was connected with implant fixture with abutment screw. Actual forces simulating occlusal load were applied to the implant and the implant surrounding the bones by rotating the expansion screw (Fig. 3B).
After the experiment, loading devices were transferred to the master casts to verify the effectiveness of the loading device, and remaining strain was measured with three strain gauges (Kyowa, Chofu, Japan), amplifier (Kyowa, Chofu, Japan), and the data acquisition program (Kyowa, Chofu, Japan) (Fig. 4). One strain gauge was placed on the center of two implants and the other two implants were placed on the distal surface of both implants, within 1mm distance, and parallel to the line connecting the center of two implants. Strain gauges were bonded to the stone surface of master cast with a thin film of cyanoacrylate adhesive (Zapit, Dental Ventures of America, Corona, CA). Each strain gauge was separately wired, and the three strain gauges were connected to a multichannel bridge amplifier to form one leg of the bridge. All strain gauges were set to zero, and then the load was applied. After 1-unit load, the microstrain curve increased to peak strain, and then it decreased to show flat curve over time. Second and third 1-unit loads were applied 1 minute after previous loading. The remained strain value at the time of the flat curve was recorded in units of microstrain (µε). Increased remained strain was recorded when each 1-unit load was applied. Descriptive statistics of remained strain after each 1-unit load was evaluated with these data. For statistic analysis, nonparametric independent t-test was used with 5% significant level and Friedman's test was also used for verification. All calculations were performed using a specific statistical program (SPSS ver. 18.0, IBM, Somers, NY).
Table 2 shows the results of each implant, grouping, initial stability and the remarkable events during the experiment. All the experimental animals maintained good general health. After teeth extraction, healing occurred without complications in all the animals. During the implant placement, post-extraction sites showed to be healed clinically. Implant placement was also performed without complications. However, during the loading period, some of the implants showed signs of inflammation around the implants. Curettage was performed to those implants under short-term general anesthesia.
Four implants, placed in dog number 2, 4, and 5, showed loss of osseointegration during the placement of the loading device, before the start of 1st loading. There were no failed implants in EL group. DL and PL group each showed one implant failure while Pm group had two failures. During periodic prophylaxis and observation periods, welding failure was discovered between the metal bars and the expansion screws. The loading device was removed immediately, covering the implant with healing abutment, and the expansion screw was welded again. One loading device had a cement failure, so it was immediately cemented again. No other complications were observed.
Only the data of strain gauges attached near the implant showed changes during loading device activation. Figure 5 shows the representative stress-strain curve while loading device was activated. Strain curve shows the increased value in strain gauge number 1 after three times of 1-unit loading. Positive value of strain complies with the increase in the length of area that the strain gauge was attached by the load, thus this means the area around the implant received tensile stress. In contrast, curve of strain gauge number 3 showed positive value right after the first 1-unit load, and then it showed negative value after second and third 1-unit load. This means the area around the implant received compressive stress. Strain gauge number 2, which was attached between the two implants showed decreased strain value after the first 1-unit load. This, however, does not showremarkable changes in strain value when the loading was applied. As shown in Figure 4, strain gauge number 2 was placed relatively distant from the two implants in order to reduce the effect from the loading. descriptive statistics was evaluated with the data acquired by strain gauge number 1 and number 3, excluding the data acquired by strain gauge number 2.
Table 3 shows descriptive statistics of remained strain, which was obtained by taking the absolute value of the difference of the strain values between each 1-unit load. Mean remained strain of each 1-unit load when the total three 1-unit loads were applied separately through the loading devices. Out of 24 total strain gauges attached near the implants, data acquired by 5 strain gauges, showing irregular strain curve pattern were excluded. With data obtained by 19 strain gauges, remained strains showed similar mean difference after the 1st, 2nd, and 3rd 1-unit load were applied. However, the data showed high deviations. With the statistic test, the absolute values of remained strain were not different after three 1-unit loadings (P=.532). Changed strain value of each implant is shown in Table 4. Some of the data showed that the amount of remained strain after three 1-unit loading was similar, however, others showed different changes of strain value under disparate series of loading.
This study was performed prior to the future study about the relationship between the loading applied to the implant and the alveolar bone regeneration. It has been widely accepted that mechanical loading plays an important role in development, maintenance, and adaptation of the skeleton.18 As a result of cyclic mechanical load, sustained micro-fractures may occur around the implants. Newly formed bone can replace microfractured bony areas and avoid fatigue fracture of the bone under bone remodeling process. And this bone adaptation process is dependent on strain magnitude, duration, frequency, history, type and distribution.19
In this study, the loading device was designed to verify that early, progressive and delayed loading might affect bone remodeling in dogs. Previous studies were performed in animals to apply occlusal load directly to the implants through the fixed prostheses. However, the chewing patterns of these animals are different from those of humans, so the loads transferred to the implants were unpredictable. Also, it is difficult to regulate the amount of force directly applied to the implant because the occlusal force is different among individual animals used in those studies. Gotfredsen et al. designed the static loading devices with orthodontic expansion screws attached between the two metal crowns supported by implants.20 This design could not simulate the true occlusal loading, because the direction of applied load was perpendicular to the long axis of the implant. So, in this study, the loading device was designed to apply loads more parallel to the long axis of implant in order to simulate the clinical conditions.
The height of the loading device was standardized 10 mm for upper metal bar, and 8 mm for the lower metal bar, and the length of the loading device was determined to be 10 mm apart between the center of implants. This dimension allows loading device to not interfere with upper teeth but to provide sufficient space for the attachment of the expansion screw and sufficient rigidity of the metal bar. The loading device was designed with cement type prosthesis. It has been broadly used in regular practices because of the convenience and the economic benefits. In this study, cemented type loading device was used to simulate the clinical conditions. During the loading period, prophylaxis and clinical observations were performed to verify cement failure. There was no cement failure except one loading device, whichoccurred in the EL group. It was cemented immediately and the loading was applied normally.
One unit load was defined as the transferred load when the expansion screw rotated 3/4 turns clockwise. When the expansion screw rotates 1/4 turn, the linear amount of displacement was 0.1 mm. If the one unit load is applied, total amount of displacement would be 0.3 mm. Supposing that the load was transferred equally to both implants, each implant would undergo 150 µm of micro-movement. It has been suggested that micromovement of 28 µm or less have no adverse effect on osseointegration, whereas micro-movement of 150 µm or more may lead to fibrous tissue healing.21,22 Therefore, one unit load was designed to apply maximum load to the implant, not exceeding 150 µm displacement. However, in the experiment, the load could not be equally transferred to each implant. One implant endured 150 µm of micro-movement and the other was able to bear over 150 µm. This is one of the reasons implant failure rate was particularly higher in this study than in other studies.
The loading devices were remounted on the master casts, and remaining strains were measured with strain gauges. During the activation of loading devices, remaining strain was recorded. These data revealed the aspect of loading through the loading devices. The loading devices were designed to apply axial direction of loads to the implants. But with these stress-strain analyses, it showed the directions of loads applied to the implants were different in the loading devices. In other words, it was predicted that the loading device would apply tensile force to one implant while compressive force to the other implant. In reality, compressive force and tensile force were combined to each implant after loading. Only one strain gauge was attached to the distal side of the implant so that the remained strain curves do not show proportional values after three loadings. This is one of the reasons that the data was highly deviated. If more strain gauges had been used around the implant, more accurate result would have appeared in this study.
Each 1-unit loading was applied one minute after previous loading. This was to eliminate the damping effect, which means that the strain value went up to the highest point and then decreased over time. In the pilot study on the stone model, there was no difference among the waiting time of 10, 5, and 1 minute, thus one-minute gap between each loading was performed in this study. Stress-strain analysis was performed on the master cast level. It was the most accurate method that the stress strain analysis can be performed on the alveolar bone of the study animals because force applied to alveolar bone was directly calculated using strain and elastic modulus of the alveolar bone. However, it is extremely difficult to attach strain gauges directly to the alveolar bone of the study animal, so it was performed on the master cast. Instead of calculating the force applied to the alveolar bone, the amount of remained strain was compared when the loading was applied to the implants. Pilot study was performed using polyurethane foam (Saw Bone, pacific Research Laboratories Inc., Vashon, WA) with acrylic resin (Ortho-Jet, Lang dental, Wheeling, IL) to verify the difference in the result between the alveolar bone and the stone cast. The result showed no difference of stress strain on the stone model compared to that of polyurethane foam.
To verify the effectiveness of the loading devices, absolute value of the remained strain after the loading was used. As both the tensile and the compressive force acted on the surface to which the strain gauge was attached, the amount of real compressive or tensile force was compared by taking the average of the absolute values of the remained strain. Though standard deviation was relatively high by each loading device, it showed no difference in the average absolute value of remained strain after each 1-unit load. It can be assumed from these stress-strain analyses that the loading devices transferred similar amount of loads to the implant effectively while not applying loads parallel to the long axis of the implant.
In this study, 4 implants failed; two in Pm group, one in PL and DL group. In this experiment, healing abutments were directly inserted after implant placement. Subsequent placement of the loading device may be affected by forces generated by tongue and other oral tissues in the implants with low initial stability. The other reason of high implant failure rate may be due to the differences in general health condition among the dogs. Few dogs were not in a good general health condition from the starting point of the experiment compared with other dogs. During teeth extraction, there were no difficulty in those dogs. In addition, during implant placement, the quality of the alveolar bone was very poor with thin cortical bone and loose trabecular bone.
There are some limitations of this loading device. Loading forces applied to the implants were static load, which is different from the dynamic load that takes place in natural oral cavity.23,24 Jemt et al. reported that static load generated by prosthesis misfit had not changed the marginal bone level.25 This means static load might not affect remodeling around the implant. To improve this problem, Duyck et al. designed a device to apply static and dynamic load.26 However, with this study on rabbit tibiae, no significantly lower bone to implant contact was found around the dynamically loaded implants in comparison to that of the statically loaded implants. Future study with adjusted loading device to apply static dynamic load is required.
Figures and Tables
Fig. 1
Loading protocols. EL: early loading, PL: periodic loading, Pm: periodic loading, rotation mobile, DL: delayed loading.
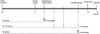
Fig. 2
Implant placement and impression taking. A: The implants were placed 10 mm apart, remaining 2 mm of buccal and lingual cortex, B: Impression was taken with impression copings and pattern resin.
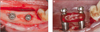
Fig. 3
Diagram of the loading device. A: A schematic illustration of the loading device, B: The direction of force applied to the implant and the surrounding alveolar bone.
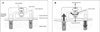
Fig. 4
Measurement of efficiency of the loading device with strain gauge. Three strain gauges were attached on the distal side of both implants and on the center of both implants.
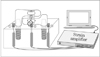
References
1. Testori T, Wiseman L, Woolfe S, Porter SS. A prospective multicenter clinical study of the Osseotite implant: four-year interim report. Int J Oral Maxillofac Implants. 2001. 16:193–200.
2. Cochran DL, Morton D, Weber HP. Consensus statements and recommended clinical procedures regarding loading protocols for endosseous dental implants. Int J Oral Maxillofac Implants. 2004. 19:109–113.
3. Ledermann P. Bar-prosthetic management of the edentulous mandible by means of plasma-coated implantation with titanium screws. Dtsch Zahnarztl Z. 1979. 34:907–911.
4. Romanos G, Froum S, Hery C, Cho SC, Tarnow D. Survival rate of immediately vs delayed loaded implants: analysis of the current literature. J Oral Implantol. 2010. 36:315–324.
5. Rocci A, Martignoni M, Burgos PM, Gottlow J, Sennerby L. Histology of retrieved immediately and early loaded oxidized implants: light microscopic observations after 5 to 9 months of loading in the posterior mandible. Clin Implant Dent Relat Res. 2003. 5:88–98.
6. Romanos GE, Nentwig GH. Immediate versus delayed functional loading of implants in the posterior mandible: a 2-year prospective clinical study of 12 consecutive cases. Int J Periodontics Restorative Dent. 2006. 26:459–469.
7. Hoshaw SJ, Brunski JB, Cochran GVB. Mechanical loading of Branemark implants affects interfacial bone modeling and remodeling. Int J Oral Maxillofac Implants. 1994. 9:345–360.
8. Frost HM. Wolff's Law and bone's structural adaptations to mechanical usage: an overview for clinicians. Angle Orthod. 1994. 64:175–188.
9. Binon PP, Sullivan DY. Provisional fixed restorations technique for osseointegrated implants. J Calif Dent Assoc. 1990. 18:23–30.
10. Misch CE. Contemporary Implant Dentistry. 1999. 2nd ed. St Louis: Mosby;595–608.
11. Appleton RS, Nummikoski PV, Pigno MA, Cronin RJ, Chung KH. A radiographic assessment of progressive loading on bone around single osseointegrated implants in the posterior maxilla. Clin Oral Implants Res. 2005. 16:161–167.
12. Roberts WE. Bone tissue interface. J Dent Educ. 1988. 52:804–809.
13. Jayme SJ, de Oliveira RR, Muglia VA, Novaes AB Jr, Ribeiro RF. The effects of different loading times on the bone response around dental implants: a histomorphometric study in dogs. Int J Oral Maxillofac Implants. 2010. 25:473–481.
14. Gostović AS, Todorović A, Lazić V, Todorović A, Milinković I, Leković V. Immediate implant loading with fixed dental restorations-an animal model study. Vojnosanit Pregl. 2012. 69:181–189.
15. Rismanchian M, Attar BM, Razavi SM, Shamsabad AN, Rezaei M. Dental implants immediate loading versus the standard 2-staged protocol: an experimental study in dogs. J Oral Implantol. 2012. 38:3–10.
16. Rismanchian M, Bajoghli F, Gholamreza T, Razavi M. Clinical, histological and histomorphometrical evaluation of early loaded implants (an animal study). J Oral Implantol. 2012. 01. 03.
17. Ivanoff CJ, Sennerby L, Lekholm U. Influence of initial implant mobility on the integration of titanium implants. An experimental study in rabbits. Clin Oral Implants Res. 1996. 7:120–127.
18. Tanck E, Homminga J, van Lenthe GH, Huiskes R. Increase in bone volume fraction precedes architectural adaptation in growing bone. Bone. 2001. 28:650–654.
19. Turner CH. Three rules for bone adaptation to mechanical stimuli. Bone. 1998. 23:399–407.
20. Gotfredsen K, Berglundh T, Lindhe J. Bone reactions adjacent to titanium implants subjected to static load. A study in the dog (I). Clin Oral Implants Res. 2001. 12:1–8.
21. Brunski JB. Biomechanical factors affecting the bone-dental implant interface. Clin Mater. 1992. 10:153–201.
22. Szmukler-Moncler S, Piattelli A, Favero GA, Dubruille JH. Considerations preliminary to the application of early and immediate loading protocols in dental implantology. Clin Oral Implants Res. 2000. 11:12–25.
23. Mishra M, Ozawa S, Masuda T, Yoshioka F, Tanaka Y. Finite element study on the effect of abutment length and material on implant bone interface against dynamic loading. J Adv Prosthodont. 2011. 3:140–144.
24. Sohn BS, Heo SJ, Koak JY, Kim SK, Lee SY. Strain of implants depending on occlusion types in mandibular implant-supported fixed prostheses. J Adv Prosthodont. 2011. 3:1–9.
25. Jemt T, Book K. Prosthesis misfit and marginal bone loss in edentulous implant patients. Int J Oral Maxillofac Implants. 1996. 11:620–625.
26. Duyck J, Rønold HJ, Van Oosterwyck H, Naert I, Vander Sloten J, Ellingsen JE. The influence of static and dynamic loading on marginal bone reactions around osseointegrated implants: an animal experimental study. Clin Oral Implants Res. 2001. 12:207–218.