Abstract
PURPOSE
This study was to investigate the effects of recombinant human platelet-derived growth factor (rhPDGF-BB) and heparin to titanium surfaces for enhancement of osteoblastic functions and inhibition of inflammation activity.
MATERIALS AND METHODS
The anodized titanium discs, not coated with any material, were used as a control group. In heparinized- Ti group, dopamine was anchored to the surface of Ti substrates, and coated with heparin. In PDGF-Ti group, rhPDGF-BB was immobilized onto heparinized Ti surface. The surface morphologies were investigated by the scanning electron microscope in each group. The release kinetics of rhPDGF-BB were analyzed, and cytotoxicity tests for each group were conducted. The biocompatibilities were characterized by measuring cell proliferation, alkaline phosphatase activity, and calcium deposition using MG-63 cells. Statistical comparisons were carried out by one-way ANOVA tests. Differences were considered statistically significant at *P<.05 and **P<.001.
RESULTS
The combination of rhPDGF-BB and heparin stimulated alkaline phosphatase activity and OCN mRNA expression in osteoblastic cells (*P<.05 and **P<.001). MG-63 cells grown on PDGF-Ti had significantly higher amounts of calcium deposition than those grown on anodized Ti (**P<.001). Heparinized Ti was more anti-inflammatory compared to anodized Ti, when exposed to lipopolysaccharide using the transcript levels of TNF-α and IL-6 of proinflammatory cytokine (*P<.05 and **P<.001).
Many studies introduced to actively induce strong osseointegration of implant surfaces by immobilizing biochemical materials such as extracellular matrix (ECM) or growth factors on the oxidized surface through biochemical modification.1-3
Platelet-derived growth factor (PDGF) is a well-characterized tissue growth factor used in various animal and human studies.3-8 It was first used in dentistry, in the field of periodontology, and has been shown to be mitogenic and chemotactic for periodontal ligament cells, with the additional effect of promoting bone, ligament, and cement regeneration.9,10 PDGF is present in the bone matrix, secreted by platelets during early fracture repair, and is locally produced at the fracture sites.11-13 It is both chemotactic and mitogenic for osteoblasts and stimulates synthesis of osteoblast type I collagen, which is the primary extracellular component of the bone.11 The cell surface receptors for PDGF increase during fracture healing, further suggesting the role of these proteins in normal fracture healing.12,13 PDGF is important in the embryologic development of the skeleton, and localized injection of PDGF into the medullary cavity accelerates fracture healing in animals.14 Also, PDGF is successfully used to treat osteoporosis in animal models, resulting in improved trabecular bone density and strength in both the flat and long bones throughout the skeleton.15
Heparin, a highly sulfated glycosaminoglycan and linear natural polysaccharide, found to have binding affinities to various growth factors, such as vascular endothelial growth factor (VEGF), basic fibroblast growth factor (bFGF), and transforming growth factor-β (TGF-β).16 Moreover, biomaterial systems with heparin have advantages for the controlled release of these growth factors.17,18
With the popularization of dental implants, the incidence of peri-implantitis is now a growing problem causing local bone destruction and resulting in failure of the implants. For the above reasons, implant surfaces should have anti-inflammatory activity and should facilitate biomolecular adhesion to enhance the osteoblast function. Several studies suggested that the incorporation of BMP-2 and heparin on titanium (Ti) surfaces had a sustained release of the growth factor, well characterized anti-inflammatory activity, and enhancement of the osteoblastic function.18-22 However, there were no studies, regarding the effects of the combination of recombinant-human-platelet-derived growth factor-BB (rhPDGF-BB) and heparin on Ti surfaces.
The purpose of this study was to investigate the effects of rhPDGF-BB and heparin on titanium surfaces for the enhancement of the osteoblastic functions and inhibition of inflammatory activity.
Prior to the immobilization of rhPDGF-BB to a heparinized-Ti surface, dopamine (DOPA) was anchored onto the surfaces of the Ti substrates. The Ti substrates were briefly immersed in a 10-mL 10-mM Tris-HCl buffer solution (pH = 8.0) containing a 2 mg/mL DOPA concentration, and were maintained for 24 h under dark conditions. After anchoring, the substrates were washed with distilled water and were dried with N2 gas. Heparin was grafted onto amine-treated Ti discs by the 1-ethyl-3dimethylaminopropyl-carbodiimide-(EDC)-mediated reaction between the primary amine groups of the Ti surface and the carboxyl groups of heparin. In brief, 2 mg/mL heparin was dissolved in a 0.1 M 2-(N-morpholino) ethanesulfonic acid (MES) buffer (pH 5.6) containing EDC. The Ti discs were then immersed in the above solution for 24 h, at room temperature (RT). After the reaction, the Ti discs were washed with distilled water, then frozen at -80℃ for 24 h, and were lyophilized for one day. rhPDGF-BB at a 5 ng/mL concentration was immobilized onto a heparinized-Ti surface. In brief, the heparinized-Ti substrates were immersed in a 0.1 M MES buffer solution (pH 5.6). rhPDGF-BB then was added to the 0.1 M MES buffer solution. The reaction was allowed to proceed for 24 h at RT.
The surface morphologies of the anodized Ti and surface-modified Ti surfaces (heparinized-Ti and rhPDGF-BB-immobilized Ti) were investigated using a scanning electron microscope (SEM, S2300, Hitachi, Tokyo, Japan). The substrates were coated with gold using a sputter coater. The SEM was operated at 10 kV.
To evaluate the release kinetics of the rhPDGF-BB-immobilized heparinized-Ti surface, the substrates were soaked in a 15-mL conical tube (Falcon, Hessle, UK) containing 1 mL PBS (pH 7.4) at 100 rpm and 37℃, respectively. At the designed time intervals of 1, 2, and 8 h and 1, 3, 5, 7, 14, 21, and 28 days, the supernatant was collected and replaced with a fresh PBS solution. All the samples were stored at -20℃ until analysis. The absorbances of rhPDGF-BB were determined via an enzyme-linked immunosorbent assay (ELISA), according to the manufacturer's instructions, using a microplate reader (Bio-Rad, Hercules, CA, USA) at a wavelength of 495 nm, respectively.
The biocompatibility of the anodized Ti and the surface-modified Ti (heparinized-Ti and rhPDGF-BB-immobilized Ti) was characterized by measuring the cell proliferation, alkaline phosphatase activity, and calcium deposition of MG-63 cells (human osteosarcoma cell line, Korean Cell Bank Line, Seoul, South Korea). Cells were cultured in Φ-100 culture plates at 37℃, in a humidified atmosphere supplied with 5% CO2. Cells were maintained in Dulbecco's modified eagle's medium (DMEM) supplemented with 10% FBS, 50 µg/mL ascorbic acid, 10 nM dexamethasone, and 10 mM β-glycerolphosphate in the presence of 100 U/mL penicillin and 100 µg/mL streptomycin. Prior to cell seeding, the specimens were sterilized with 70% EtOH for 1 min and were rinsed twice with phosphate-buffered saline (PBS).
Cytotoxicity tests for anodized Ti and surface-modified Ti were conducted according to the ISO/EN 10993 Part 5 guidelines. To obtain extraction media, the DMEM medium was incubated with anodized Ti and surface-modified Ti (heparinized-Ti and rhPDGF-BB-immobilized Ti), respectively, for 24 h, at 37℃. MG 63 cells were seeded into 96-well plates at a concentration of 5×104 cells/well and were incubated for 24 h at 37℃, with DMEM supplemented with 10% FBS, and 1% 100 U/mL penicillin and 100 µg/mL streptomycin. After 24 h culture, the DMEM medium was removed from the 96-well plates, the cells were washed with PBS, and the extraction media were added. The cells were incubated for 24 and 48 h. At each time point, the extraction medium was aspirated and CCK-8 proliferation kit (Dojindo, Kumamoto, Japan) reagents were added to the cells. The cells were then incubated for 1 h at 37℃, and the optical density of the live cells was measured using a microplate reader at a wavelength of 450 nm. In addition, the viability of the cells on the surface of the anodized Ti and surface-modified Ti (heparinized-Ti and rhPDGF-BB-immobilized Ti) was assessed via live/dead staining. In brief, MG-63 cells were seeded at a density of 5×104 cells/mL on the anodized Ti and surface-modified Ti (heparinized-Ti and rhPDGF-BB-immobilized Ti), respectively. After 48 h incubation, the substrates were rinsed three times with PBS and incubated with live/dead staining (2 µM calcein AM and 4 µM ethidium homodimer-1) for 30 min at RT. The viable cells (green) and dead cells (red) were counted under a confocallaser scanning microscope (CLSM, EZ-C1, Nikon, Tokyo, Japan).
MG-63 cells were allotted into 1×105 cells per dose and inoculated on an anodized Ti surface and surface-modified Ti surfaces (heparinized-Ti and rhPDGF-BB-immobilized Ti) and then maintained for seven days. At the predesignated time intervals of the one-, three-, and seven-day incubation, the substrates were rinsed with PBS, and CCK-8 proliferation kit reagents were added to the specimens. After 1h incubation, the reagents were carefully transferred to 96-well plates. The optical density was measured, using a microplate reader, at a wave-length of 450 nm.
ALP activity was measured after 7, 14, and 21 days of culture. In brief, cells were seeded at a density of 1×105 cells/ml on an anodized Ti surface and surface-modified Ti surfaces (heparinized-Ti and rhPDGF-BB-immobilized Ti). The cells were washed with PBS and then with a 1X RIPA buffer [50 mM Tris-HCl, pH7.4, 150 mM NaCl, 0.25% deoxycholic acid, 1% NP-40, 1 mM EDTA, including protease and phosphatase inhibitors (1 mM PMSF, 1 mM sodium orthovanadate, 1 mM sodium fluoride, 1 µg/mL aprotinin, 1 µg/mL leupeptin, and 1 µg/mL pepstatin)], which was added to the cells. The cells in the RIPA buffer were sonicated using a Vibra Cell™ instrument (Sonics & Materials Inc., Danbury, CT, USA) for 1 minute at 110 Watts, on ice. After sonication, the cell lysates were centrifuged at 13,500 rpm and at 4℃ for 3 minute to remove the cell debris. The supernatants were incubated with p-nitrophenyl phosphate solution for 30 minute at 37℃. The reaction was stopped by adding 500 µl of 1 N NaOH. The ALP activity was determined by measuring the conversion of p-nitrophenyl phosphate to p-nitrophenol. The optical density was determined by using a microplate reader at a wavelength of 405 nm.
MG-63 cells were seeded at a density of 1×105 cells on anodized Ti and surface-modified Ti (heparinized-Ti and rhPDGF-BB-immobilized Ti). At 21 days, the cells were washed with PBS and were gently scraped off from the surfaces of the substrates. The cells were harvested via centrifugation at 13,500 rpm for 1 min, and lysis buffer (0.1% Triton X-100) was then added to the cells. The cells were sonicated on ice for 1 min to pulverize the cell membranes. The resulting supernatant was used for calcium deposition measurements using a QuantiChrom™ Calcium Assay Kit (DICA-500, BioAssay Systems, Davis, CA, USA), according to the manufacturer's instructions. The amount of calcium produced was estimated by measuring the absorbance at 612 nm using a microplate reader.
The mRNA expression levels of osteocalcin and osteopontin in MG-63 cells cultured on anodized Ti and surface-modified Ti (heparinized Ti and rhPDGF-BB-immobilized Ti) were assessed after 21-day incubation, via real-time PCR. In brief, 1×105 cells of MG-63 were seeded on the surface of the anodized Ti and on surface-modified Ti at 37℃ in a 5% CO2 incubator. After the 21-day incubation, the cDNA was synthesized using the Superscript First-Strand Synthesis System (Invitrogen, Carlsbad, CA, USA), according to the manufacturer's directions, using 1 µg total RNA with oligo (dT). The cDNA was amplified via PCR, using an RNA PCR kit (Bioneer Inc., Daejeon, South Korea), according to the manufacturer's instructions. The following oligonucleotide primers were used: osteocalcin (OSC) [(F) 5'-TGA GAG CCC TCA CAC TCC TC-3', (R) 5'-ACC TTT GCT GGA CTC TGC AC-3', osteopontin (OSP) [(F) 5'-GAG GGC TTG GTT GTC AGC-3', (R) 5'-CAA TTC TCA TGG TAG TGA GTT TTC C-3', GAPDH [(F) 5'-ACT TTG TCA AGC TCA TTT CC -3', (R) 5'-TGC AGC GAA CTT TAT TGA TG -3']. Real-time PCR reaction was performed with the above-mentioned specific primers. DyNAmo™ SYBR® Green qPCR Kit (Finnzymes, Espoo, Finland) and PCR amplification and detection were carried out on an ABI7300 Real-Time Thermal Cycler (Applied Biosystems, Foster, CA, USA). All the results were confirmed by repeating the experiment three times. The relative levels of OSC and OSP were normalized to GAPDH.
To assess the anti-inflammatory effects, MG-63 cells with a density of 1×105 cells were seeded on the surfaces of the anodized Ti and heparinized Ti. After 24 h incubation, the cultured cells were exposed to (100 ng/mL) lipopolysaccharide (LPS). The cells exposed by LPS were harvested for total RNA isolation. The isolated total RNA was extracted using the RNeasy Plus Mini Kit (Qiagen GmbH, Hilden, Germany), and 1 µg total RNA was reverse-transcribed into cDNA using AcccuPower RT PreMix (Bioneer, Daejeon, South Korea) according to the manufacturer's protocol. The following oligonucleotide primers were used: TNF-α[(F) 5'-GGC AGG TCT ACT TTG GAG TCA TTG C-3' (R) 5'-ACA TTC GAG GCT CCA GTG AAT TCG G-3'] IL-6; [(F) 5'-CTG GTG ACA ACC ACG GCC TTC CCT A-3' (R) 5'-ATG CTT AGG CAT AAC GCA CTA GGT T-3'] GAPDH; and [(F) 5'-ACT TTG TCA AGC TCA TTT CC -3', (R) 5'-TGC AGC GAA CTT TAT TGA TG -3']. Real-time PCR reaction was performed with the above-mentioned specific primers. DyNAmo™ SYBR® Green qPCR Kit (Finnzymes, Espoo, Finland) was used, and PCR amplification and detection were carried out on an ABI7300 real-time thermal cycler (Applied Biosystems, Foster, CA, USA). All the results were confirmed by repeating the experiment three times. The relative levels of OSC and OSP were normalized to GAPDH.
As shown in Fig. 1, the surface morphologies of the anodized Ti and surface-modified Ti were determined via scanning electron microscopy. The morphology of the anodized-Ti surface was similar to those of the surface-modified Ti surfaces.
The release kinetic of rhPDGF-BB immobilized on a heparinized-Ti surface was analyzed using enzyme-linked immunosorbent assay (Fig. 2). The amount of rhPDGF-BB released on the first day was approximately 32%. Over a release period of 28 days, 3.65 ± 0.40 ng rhPDGF-BB was released from the 5-ng rhPDGF-BB-immobilized heparinized-Ti surface.
The results of the cytotoxicity tests were confirmed by using MG-63 cells for anodized Ti, heparinized Ti, and rhPDGF-BB-immobilized Ti prior to osteoblastic cell proliferation and differentiation. There were no significant cytotoxic effects during the culture periods of up to 48 h (Fig. 3). Another cytotoxicity test was also performed for anodized Ti, heparinized Ti, and rhPDGF-BB-immobilized Ti, using live/dead assay. The live and dead cells were labeled with green and red fluorescence, respectively. Almost all the cells were alive after 48 h incubation on the surfaces of the anodized Ti, heparinized Ti, and rhPDGF-BB-immobilized Ti (Fig. 4).
The proliferation of the MG-63 cells cultured on anodized Ti and surface-modified Ti was assessed after one, three, and seven days of time. As shown in Fig. 5, the cultured MG-63 cells in all the groups increased throughout the incubation period for up to seven days. There were no significant differences in the proliferation of the cells grown on anodized Ti or surface-modified Ti after seven-day culture. In addition, the MG-63 cell proliferation on the heparinized-Ti and rhPDGF-BB-immobilized Ti surfaces was not significantly different from that on the anodized-Ti surfaces.
The ALP activity of the MG-63 cells was investigated after 7, 14, and 21 days on the surfaces of the anodized Ti and surface-modified Ti. As shown in Fig. 6, the MG-63 cells grown on the rhPDGF-BB-immobilized Ti had a significantly higher ALP activity than those cultured on the anodized Ti (*7 days: *P<.05; 14 days: **P<.001; and 21 days: *P<.05) at the time of analysis. In addition, there were significant differences in ALP activity between the MG-63 cells cultured on heparinized Ti and rhPDGF-BB-immobilized Ti after incubation for up to 21 days (7 days: *P<.05; 14 days: **P<.001; and 21 days: *P<.05).
The amount of calcium deposition by the MG-63 cells cultured on anodized Ti, heparinized Ti, and rhPDGF-BB-immobilized Ti was analyzed after 21 days of incubation. The results in Fig. 7 clearly show that the MG-63 cells grown on the rhPDGF-BB-immobilized Ti had significantly higher amounts of calcium deposition than those grown on the anodized Ti (**P<.001). Moreover, there were significant differences in calcium deposition between the MG-63 cells cultured on the rhPDGF-BB-immobilized Ti and those cultured on the heparinized Ti (**P<.001).
Osteogenic differentiation was also performed using the results of the real-time PCR for the mRNA expression of osteocalcin and osteopontin after 21-day incubation. As shown in Fig. 8(A), the osteopontin expression of the MG-63 cells cultured on the rhPDGF-BB-immobilized Ti was 2.5-fold higher than of those that were cultured on the anodized Ti. A significant difference in the osteopontin expression between the anodized Ti and the rhPDGF-BB-immobilized Ti was detected after 21 days (*P<.05). There were no significant differences, however, in osteopontin expression between the cells grown on the heparinized Ti and those grown on the rhPDGF-BB-immobilized Ti. The osteocalcin expression of the MG-63 cells grown on the rhPDGF-BB-immobilized Ti was 3.2-fold higher than of those grown on the anodized Ti (Fig. 8B). In addition, the osteocalcin expression was 2.0-fold higher in the rhPDGF-BB-immobilized Ti than in the heparinized Ti. There were significant differences in osteocalcin expression between the MG-63 cells grown on the anodized Ti and those grown on the rhPDGF-BB-immobilized Ti (**P<.001). Furthermore, the MG-63 cells that were cultured on the rhPDGF-BB-immobilized Ti had a significantly higher osteocalcin expression than those that were cultured on the heparinized Ti (**P<.001).
An anti-inflammatory effect of the heparinized Ti was confirmed compared to the anodized Tiafter LPS exposure, using the transcript levels of the TNF-α and IL-6 of proinflammatory cytokine. The mRNA levels of the TNF-α and IL-6 of the MG-63 cells cultured on an anodized-Ti surface after LPS exposure were 2.0- and 2.2-fold higher, respectively, when compared to those of the MG-63 cells only, without LPS exposure (Fig. 9). The mRNA levels of the TNF-α and IL-6 of the MG-63 cells cultured on a heparinized-Ti surface exposed to LPS were 57 and 65% lower than those that were cultured on the anodized Ti exposed to LPS, respectively.
As the implant surface is related to successful osseointegration and to shortened the required healing time, various methods of coating surface have been investigated.1,2 Therefore, the implant technologies focus on optimizing the surface characteristics, including the chemical components, electrical charges, fine surface structures, and porosity, to lead to in-vivo tissue reactions promoting osseointegration.23,24 Titanium (Ti) and its alloys have been widely used as implant materials. Titanium spontaneously forms an approximately-10-nm-thick oxidized layer (TiO2) on its surface during the preparation process.25 This TiO2, however, cannot be used as a passivity layer because it is generally thin and heterogeneous. Numerous methods have attempted to overcome the limitations of spontaneously formed TiO2 to improve its biocompatibility, by creating artificial anodized membranes through various methods, such as chemical, plasma, or anodic oxidation, through an electrochemical technique.25-27 Many studies have been conducted of late to actively induce strong osseointegration of implant surfaces by immobilizing biochemical materials such as extracellular matrix (ECM) or growth factors on the oxidized surface, through biochemical modification.2,3,23
In this study, the free amino groups of 3-aminopropyltriethoxysilane (ATPES) were first anchored onto the titanium surface to create high-positive-charge regions, and then heparin was covalently grafted to the titanium surface using a 1-ethyl-3-dimethylaminopropyl-carbodiimide-(EDC)-mediated coupling reaction between the primary amine groups of the Ti surface and the carboxyl groups of heparin. rhPDGF-BB was immobilized onto the heparinized-Ti surface.
ALP activity and calcium deposition are widely used as markers for the early and late differentiation of osteoblast cells, respectively.28,29 ALP activity was measured after a culture period of 7, 14, and 21 days. The MG-63 cells grown on the rhPDGF-BB-immobilized Ti surface had a significantly higher ALP activity than those that were cultured on anodized-Ti surfaces for different culture periods. In addition, there were significant differences in ALP activity between the MG-63 cells cultured on heparinized Ti and the rhPDGF-BB-immobilized Ti after incubation for up to 21 days. Thus, rhPDGF-BB stimulates osteoblastic differentiation. The amount of calcium deposition was analyzed by the MG-63 cells cultured on the anodized Ti, heparinized Ti, and rhPDGF-BB-immobilized Ti after 21-day incubation. The MG-63 cells grown on the rhPDGF-BB-immobilized Ti had significantly higher amounts of calcium deposition than those grown on the anodized Ti. Moreover, there were significant differences in calcium deposition between the MG-63 cells cultured on the rhPDGF-BB-immobilized Ti and those cultured on the heparinized Ti. These results indicate that rhPDGF-BB-immobilized Ti substrates can stimulate matrix formation and can enhance the osteoblastic function.
Heparin has well-characterized anti-inflammatory and anti-coagulant properties. The anti-inflammatory effects of heparinized Ti and anodized Ti were examined by measuring the transcript levels of the tumor necrosis factor-α(TNF-α) and interleukin 6 (IL-6) of proinflammatory cytokine. The expression of these cytokines was less detected in the MG-63 cells grown on the heparinized Ti than on the anodized Ti. Low-molecular-weight and unfractionated heparins induced a down-regulation of pro-inflammatory cytokines and NF-κB in LPS-stimulated human monocytes.30 These results suggest that heparin has an anti-inflammatory activity, consistent with the results of this study.
In this study, it was found that the modification of the Ti surfaces with heparin and PDGF had an anti-inflammatory effect on the MG-63 cells and resulted in the enhancement of the osteoblastic function. The immobilization of rhPDGF-BB on the heparinized-Ti surface caused the sustained release of rhPDGF-BB within a sufficient time and in sufficient amounts. It was demonstrated that the immobilization of rhPDGF-BB onto the heparinized-Ti surface sustained the release over an extended period. These results demonstrate that heparin is a suitable material for the sustained release of growth factors. An increase in osteoblastic proliferation was observed when the MG-63 cells were cultured on all the substrates. Additional research is needed, however, to assess the potential of the rhPDGF-BB- and heparin-immobilized Ti substrates for animal study.
Figures and Tables
Fig. 1
SEM of various Ti surfaces: (A) anodized Ti; (B) heparinized Ti; and (C) rhPDGF-BB-immobilized Ti.
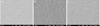
Fig. 3
Cytotoxicity tests of the anodized Ti, heparinized Ti, and rhPDGF-BB-immobilized Ti during culture periods of up to 48 h.
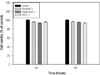
Fig. 4
Live/dead assay. (A) anodized Ti; (B) heparinized Ti; and (C) rhPDGF-BB-immobilized Ti after 48 h incubation.
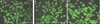
Fig. 5
Proliferation of the MG-63 cells cultured on anodized Ti, heparinized Ti, and rhPDGF-BB-immobilized Ti after one-, three-, and seven-day culture.
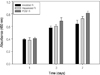
Fig. 6
ALP activity of the MG-63 cells cultured on anodized Ti, heparinized Ti, and rhPDGF-BB-immobilized Ti after 7-, 14-, and 21-day incubation (*P<.05 and **P<.001).
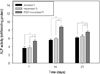
Fig. 7
Calcium deposition of the MG-63 cells grown on the anodized Ti, heparinized Ti, and rhPDGF-BB-immobilized Ti after 21-day incubation (**P<.001).
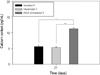
References
1. Rezania A, Healy KE. The effect of peptide surface density on mineralization of a matrix deposited by osteogenic cells. J Biomed Mater Res. 2000. 52:595–600.
2. Bab I, Chorev M. Osteogenic growth peptide: from concept to drug design. Biopolymers. 2002. 66:33–48.
3. Giannobile WV. Periodontal tissue engineering by growth factors. Bone. 1996. 19:23S–37S.
4. Park YJ, Ku Y, Chung CP, Lee SJ. Controlled release of platelet-derived growth factor from porous poly(L-lactide) membranes for guided tissue regeneration. J Control Release. 1998. 51:201–211.
5. Camelo M, Nevins ML, Schenk RK, Lynch SE, Nevins M. Periodontal regeneration in human Class II furcations using purified recombinant human platelet-derived growth factor-BB (rhPDGF-BB) with bone allograft. Int J Periodontics Restorative Dent. 2003. 23:213–225.
6. Nevins ML, Camelo M, Lynch SE, Schenk RK, Nevins M. Evaluation of periodontal regeneration following grafting intrabony defects with bio-oss collagen: a human histologic report. Int J Periodontics Restorative Dent. 2003. 23:9–17.
7. Nevins M, Camelo M, Nevins ML, Schenk RK, Lynch SE. Periodontal regeneration in humans using recombinant human platelet-derived growth factor-BB (rhPDGF-BB) and allogenic bone. J Periodontol. 2003. 74:1282–1292.
8. Nevins M, Giannobile WV, McGuire MK, Kao RT, Mellonig JT, Hinrichs JE, McAllister BS, Murphy KS, McClain PK, Nevins ML, Paquette DW, Han TJ, Reddy MS, Lavin PT, Genco RJ, Lynch SE. Platelet-derived growth factor stimulates bone fill and rate of attachment level gain: results of a large multicenter randomized controlled trial. J Periodontol. 2005. 76:2205–2215.
9. Lynch SE, de Castilla GR, Williams RC, Kiritsy CP, Howell TH, Reddy MS, Antoniades HN. The effects of short-term application of a combination of platelet-derived and insulin-like growth factors on periodontal wound healing. J Periodontol. 1991. 62:458–467.
10. Lynch SE, Williams RC, Polson AM, Howell TH, Reddy MS, Zappa UE, Antoniades HN. A combination of platelet-derived and insulin-like growth factors enhances periodontal regeneration. J Clin Periodontol. 1989. 16:545–548.
11. Bolander ME. Regulation of fracture repair by growth factors. Proc Soc Exp Biol Med. 1992. 200:165–170.
12. Andrew JG, Hoyland JA, Freemont AJ, Marsh DR. Platelet-derived growth factor expression in normally healing human fractures. Bone. 1995. 16:455–460.
13. Fujii H, Kitazawa R, Maeda S, Mizuno K, Kitazawa S. Expression of platelet-derived growth factor proteins and their receptor alpha and beta mRNAs during fracture healing in the normal mouse. Histochem Cell Biol. 1999. 112:131–138.
14. Nash TJ, Howlett CR, Martin C, Steele J, Johnson KA, Hicklin DJ. Effect of platelet-derived growth factor on tibial osteotomies in rabbits. Bone. 1994. 15:203–208.
15. Mitlak BH, Finkelman RD, Hill EL, Li J, Martin B, Smith T, D'Andrea M, Antoniades HN, Lynch SE. The effect of systemically administered PDGF-BB on the rodent skeleton. J Bone Miner Res. 1996. 11:238–247.
16. Sasisekharan R, Ernst S, Venkataraman G. On the regulation of fibroblast growth factor activity by heparin-like glycosaminoglycans. Angiogenesis. 1997. 1:45–54.
17. Perets A, Baruch Y, Weisbuch F, Shoshany G, Neufeld G, Cohen S. Enhancing the vascularization of three-dimensional porous alginate scaffolds by incorporating controlled release basic fibroblast growth factor microspheres. J Biomed Mater Res A. 2003. 65:489–497.
18. Ishibe T, Goto T, Kodama T, Miyazaki T, Kobayashi S, Takahashi T. Bone formation on apatite-coated titanium with incorporated BMP-2/heparin in vivo. Oral Surg Oral Med Oral Pathol Oral Radiol Endod. 2009. 108:867–875.
19. Young E. The anti-inflammatory effects of heparin and related compounds. Thromb Res. 2008. 122:743–752.
20. von Walter M, Herren C, Gensior TJ, Steffens GC, Hermanns-Sachweh B, Jahnen-Dechent W, Rüger M, Erli HJ. Biomimetic modification of the TiO2/glass composite Ecopore with heparinized collagen and the osteoinductive factor BMP-2. Acta Biomater. 2008. 4:997–1004.
21. Kodama T, Goto T, Miyazaki T, Takahashi T. Bone formation on apatite-coated titanium incorporated with bone morphogenetic protein and heparin. Int J Oral Maxillofac Implants. 2008. 23:1013–1019.
22. Kim SE, Song SH, Yun YP, Choi BJ, Kwon IK, Bae MS, Moon HJ, Kwon YD. The effect of immobilization of heparin and bone morphogenic protein-2 (BMP-2) to titanium surfaces on inflammation and osteoblast function. Biomaterials. 2011. 32:366–373.
23. Clark AE, Hench LL, Paschall HA. The influence of surface chemistry on implant interface histology: a theoretical basis for implant materials selection. J Biomed Mater Res. 1976. 10:161–174.
24. Puleo DA, Nanci A. Understanding and controlling the bone-implant interface. Biomaterials. 1999. 20:2311–2321.
25. De Giglio E, Sabbatini L, Colucci S, Zambonin G. Synthesis, analytical characterization, and osteoblast adhesion properties on RGD-grafted polypyrrole coatings on titanium substrates. J Biomater Sci Polym Ed. 2000. 11:1073–1083.
26. Tosatti S, De Paul SM, Askendal A, Vande Vondele S, Hubbell JA, Tengvall P, Textor M. Peptide functionalized poly(L-lysine)-g-poly(ethylene glycol) on titanium: resistance to protein adsorption in full heparinized human blood plasma. Biomaterials. 2003. 24:4949–4958.
27. Damsky CH. Extracellular matrix-integrin interactions in osteoblast function and tissue remodeling. Bone. 1999. 25:95–96.
28. Turksen K, Bhargava U, Moe HK, Aubin JE. Isolation of monoclonal antibodies recognizing rat bone-associated molecules in vitro and in vivo. J Histochem Cytochem. 1992. 40:1339–1352.
29. van den Beucken JJ, Walboomers XF, Boerman OC, Vos MR, Sommerdijk NA, Hayakawa T, Fukushima T, Okahata Y, Nolte RJ, Jansen JA. Functionalization of multilayered DNA-coatings with bone morphogenetic protein 2. J Control Release. 2006. 113:63–72.
30. Hochart H, Jenkins PV, Smith OP, White B. Low molecular weight and unfractionated heparins induce a downregulation of inflammation: decreased levels of proinflammatory cytokines and nuclear factor-kappaB in LPS-stimulated human monocytes. Br J Haematol. 2006. 133:62–67.