Abstract
PURPOSE
The aim of this study was to evaluate the influence of resin cement thickness on the microtensile bond strength between zirconium-oxide ceramic and resin cement.
MATERIALS AND METHODS
Thirty-two freshly extracted molars were transversely sectioned at the deep dentin level and bonded to air-abraded zirconium oxide ceramic disks. The specimens were divided into 8 groups based on the experimental conditions (cement type: Rely X UniCem or Panavia F 2.0, cement thickness: 40 or 160 µm, storage: thermocycled or not). They were cut into microbeams and stored in 37℃ distilled water for 24 h. Microbeams of non-thermocycled specimens were submitted to a microtensile test, whereas those of thermocycled groups were thermally cycled for 18,000 times immediately before the microtensile test. Three-way ANOVA and Sheffe's post hoc tests were used for statistical analysis (α=95%).
RESULTS
All failures occurred at the resin-zirconia interface. Thermocycled groups showed lower microtensile bond strength than non-thermocycled groups (P<.001). Differences in cement thickness did not influence the resin-zirconia microtensile bond strength given the same resin cement or storage conditions (P>.05). The number of adhesive failures increased after thermocycling in all experimental conditions. No cohesive failure was observed in any experimental group.
Densely-sintered high-purity zirconium-oxide ceramic has been widely used in the field of restorative dentistry because of its favorable aesthetic characteristics and excellent mechanical properties.1-4 With the advance of computer-aided design/manufacturing (CAD/CAM) technologies, dental zirconia is currently applied to frameworks for fixed dental prostheses, implant abutments as well as single all-ceramic restorations.1-3 A major weakness of dental zirconia is its inferior ability to adhere to resin cement.5 As zirconia has a polycrystalline structure and limited vitreous phase, neither hydrofluoric acid etching nor silanization can achieve durable zirconia-resin bonding.5-7
Various methods have been used to establish durable adhesion between dental zirconia and resin cement. Air abrasion has been used to increase the roughness and wettability of zirconia surfaces. Increased bond strength was reported when zirconia surfaces were air-abraded with Al2O3.8-10 Initial defects created by air abrasion were reported as a risk factor for compromising the mechanical strength of dental zirconia.11 However, if resin cement was used to fill the defects, the strength of zirconia was maintained.12 Primers and cements, which consist of adhesive monomers, have been used to achieve chemical bonds between resin and zirconia. Methacrylate phosphoric ester13 and 10-methacryloxyethyl-dehydrogenphosphate (10-MDP)11,12 are known as adhesive functional monomers. Wolfart et al.14 reported favorable tensile bond strength when they applied Panavia F 2.0, which contains 10-MDP, to an air-abraded zirconia surface. These authors emphasized the role of the adhesive functional monomer. Self-adhesive cement has been used with the advantages of simplified luting procedures without additional tissue conditioning. Yang et al.15 reported that Rely X UniCem (3M ESPE, St. Paul, USA) showed high bond strength to an air-abraded zirconia surface without priming. Rely X UniCem contains an adhesive phosphoric acid monomer that forms a chemical bond with the zirconia surface. In addition to air abrasion and functional monomers, attempts have also been made to enhance resin-zirconia bonding with tribochemical silica coating,16 heat-induced maturation, and selective infiltration-etching.17
Long-term water storage and thermocycling have been used to evaluate the durability of resin-zirconia bonding. Wegner et al.18 assessed the influence of different storage conditions on the bond strength of adhesive bonding systems with zirconia. They concluded that thermocycling has a much higher impact on the durability of resin-zirconia bonding. Lüthy et al. reported that Bis-GMA cement showed weak resistance to thermocycling conditions whereas resin cement containing adhesive functional monomer showed durable bonding capacity under both thermocycled and non-thermocycled conditions.18,19
Accurate adaptation is essential to successful all-ceramic dental restorations with good long-term prognoses. It has been reported that an internal gap size of 50 - 100 µm was suitable for the performance of resin cement.20,21 Reich et al.22 measured the internal gap sizes of 3-unit zirconia fixed partial dentures (FPDs) using a replica technique and concluded that the internal gaps of the zirconia frameworks, which were up to 105 µm, were greater than those of cast metal frameworks. Kohorst et al.23 investigated the marginal and internal gap sizes of 4-unit zirconia FDPs before and after veneering procedures. Although the mean internal gap size ranged from 71.1 to 115.1 µm, the maximum internal gap size ranged from 111.0 to 183.6 µm. Wettstein et al.24 and Sailer et al.25 also concluded that zirconia frameworks resulted in greater internal gaps than metal frameworks, and regarded this as a risk factor which could compromise long-term survival. Poor internal adaptation clearly produces greater cement thickness and could influence the durability of bond strength. Many experiments have been performed to measure the resin-zirconia bond strength under conditions differing in surface treatment, resin cement, and the use of primers among other factors. However, until now, there has been no comparison to evaluate the resin-zirconia bond strength under conditions differing in the thickness of resin cement.
The purpose of this study was to evaluate the microtensile bond strength between resin cement and air-abraded zirconia under different thicknesses of resin cement. Additionally, the effects of thermocycling and type of resin cement were also evaluated.
Thirty-two adult molars were stored in 10% formalin for a week. The coronal and root portion of each molar were ground flat using a model trimmer until intact dentin was exposed. The thickness of the molar specimens was approximately 5 mm after grinding. Sixteen cylinder-shaped (diameter: 16 mm, thickness: 15 mm) zirconium oxide blocks (ZS blank, Everest, Kavo, Lake Zurich, Ill, Germany) were sintered and transversely sectioned in half, producing 32 zirconium oxide discs 7 - 8 mm thick. The manufactured flat surfaces were chosen for the bonding surfaces. The bonding surface of each disc was incrementally ground and finished with 400-, 600-, 800-, 1200-grit silicone carbide paper under water irrigation, after which the specimens were ultrasonically cleaned in distilled water for 3 min. The specimens were air-abraded by an airborne particle abrasion unit (RONDOflex plus 360, Kavo) with 50 µm Al2O3 particles (Kavo) at 55 psi for 15 s from a perpendicular distance of 10 mm.
The zirconia discs and teeth were randomly divided into 8 groups according to the type of cement used, cement thickness, and storage conditions (Table 1).
A customized jig was fabricated to control the thickness of resin cement between the dentin and a zirconia disc. Each zirconia disc was positioned on the stabilizing mold of the customized jig. Tofflemire matrix bands (Rihana, USA) with a thickness of approximately 40 µm were utilized to provide a resin cement thickness of either 40 or 160 µm. For specimens with a cement thickness of 40 µm, a single Tofflemire matrix band was positioned at each periphery of the bonding surface and stabilized by fixation screws. A small amount of cyanoacrylate (Zapit, Dental ventures of America Inc., Coraona, CA, USA) was additionally used to stabilize the bands to the zirconia disc. For specimens with a cement thickness of 160 µm, 4 Tofflemire matrix bands were positioned at each periphery of the bonding surface, and fixation screws and cyanoacrylate were utilized to stabilize the bands. For specimens using Panavia F 2.0 (Kuraray Medical Inc, Japan) as the resin cement, primers A and B were mixed and applied on the cut dentin surface for 60 s. The resin cement was mixed according to the manufacturer's instructions and applied over the zirconia surface; subsequently, the dentin surface of each cut tooth was positioned on the resin cement. A constant pressure of 15 Ncm torque was applied for 5 min, and light irradiation (Satelec Mini LED, Satelec Bordeaux, France) was then performed for 40 s from each side of the specimen (Fig. 1). The bonded zirconia-resin-tooth specimens were stored in distilled water for 24 h at 37℃.
Bonded zirconia-resin-tooth specimens were mounted on a custom holding device designed to rotate the specimen at 90° angles on a sawing machine (Topmet Metsaw-LS, R&B Inc., Daejun, Korea). The specimens were sectioned using a low-speed precision diamond saw (4"Wafering Blade, Allied High Tech Products Inc., Rancho Domingues, CA, USA) under water irrigation. Approximately 10 to 12 microbeams of 1 mm × 1 mm × 10 mm were obtained from each specimen (Fig. 2). A digital image of each microbeam was taken to measure the cement thickness (Fig. 3) using the UTHSCA (University of Texas Health Science Center in San Antonio) Image Tool.
After measuring the cement thickness, the microbeams of non-thermocycled groups were submitted to a microtensile bond strength test. For the thermocycled groups, the microbeam specimens were submitted to thermocycling between 5 and 55℃ for 18,000 cycles (dwell time: 30 s, transfer time: 2 s), and then submitted to the microtensile bond strength test.
Each specimen was fixed with cyanoacrylate (Zapit Base, Dental ventures of America Inc.) on the microtensile testing jig. The specimens were positioned parallel to the long axis of the jig in order to minimize bending stresses. The jig was mounted on a universal testing machine (EZ-S, Shimadzu, Japan) as parallel as possible relative to the applied tensile load. Testing was performed at a crosshead speed of 1 mm/min.
After the microtensile test, all microbeams were analyzed using a light microscope (Nikon Cooperation, Japan) at 40× magnification to verify the failure modes. Failure modes were categorized as follows: adhesive failure between resin and zirconia, cohesive failure of cement, or mixed failure.
Table 2 presents a summary of the measured resin cement thickness for each group. The mean values ranged from 62.65 to 68.19 µm and from 164.08 to 172.68 µm in groups with cement thicknesses of 40 and 160 µm, respectively.
Table 3 presents the means and standard deviations of microtensile bond strength for each group. The specimens bonded with Panavia F 2.0 showed significantly greater microtensile bond strength than those bonded with Rely X UniCem (P<.001). Thermocycled groups showed significantly lower microtensile bond strength than non-thermocycled groups. Table 4 shows a summary of the 3-way ANOVA for microtensile bond strength conducted at each level of interacting factor.
Ever since zirconia was introduced as a dental restorative material, a great number of studies have evaluated the resin-zirconia bond strength using different zirconia surface treatments or resin cements with different compositions. One of the clinical concerns of zirconia restorations generated by CAD/CAM techniques is that the internal gap size is greater than that of conventional metal-ceramic restorations,22,24 which inevitably result in thicker layers of luting cement. The mechanical properties of the resin cement are degraded over the long term in an oral environment, which is often simulated experimentally by long-term water storage and thermocycling. During thermocycling, resin cement undergoes hydrolytic degradation, which contributes to the decrease in bond strength.25 Therefore, for zirconia restorations, which commonly have greater internal and marginal gap size, the thickness of resin cement could be an influential factor for the resin-zirconia bond. Greater degradation may occur for thicker cement layers.
Thus far, there is no report on the effect of resin cement thickness on resin-zirconia bonding. The current study is the first to evaluate the influence of resin cement thickness on the microtensile bond strength between resin cement and air-abraded zirconia. In terms of experimental design, two cement thicknesses were selected for the current study. The thickness of 40 µm represented a good-fitting zirconia restoration whereas that of 160 µm represented a poorly fit restoration. The value of 160 µm was chosen on the basis of a previous study in which the maximum internal gap sizes of zirconia FPDs were 183.6 and 158.0 µm at the premolars and molars, respectively. Given that the thickness of a single Tofflemire matrix band was 40 µm, a thickness of 160 µm could be reproduced by applying 4 bands over the zirconia disc. Considering the cement thickness as a single influencing factor, thick cement resulted in significantly greater bond strength rather than thin cement (P<.001). One reason for this may be that a thicker resin cement provides greater plasticity than a thinner resin cement, absorbing a greater microtensile force until debonding. Another explanation draws upon the concept of a configuration factor (C-factor).26 The C-factor was smaller in the 160 µm groups than in the 40 µm groups; therefore, thicker cement could cause less strain in the zirconia-resin-dentin complex and could lead possibly greater microtensile bond strength. However, when the experimental conditions were equated for resin cement and storage condition, resin cement thickness had no influence on microtensile bond strength. As noted above, thermocycling is commonly used to simulate the in vivo oral environment. In the present study, the microtensile bond strength significantly decreased after thermocycling in every experimental group (NTC > TC, P<.001). Two major mechanisms are considered as the reasons of this effect. One is the hydrolytic degradation at the bonded interface between the composite resin and the zirconia surface. The other is the degradation of the composite matrix and the filler particles within the composite resin itself.18 In this study, the number of adhesive failures increased in all thermocycled groups. In addition, no cohesive failure was observed in any experimental group. Therefore, it is inferred that the decrease in bond strength after thermocycling was not due to the degradation of the resin matrix itself but in large part to the hydrolytic degradation at the bonded interface. This explains why cement thickness had no influence on the microtensile bond strength between resin and air-abraded zirconia, as the bonded surface areas were not very different for the 40 and 160 µm groups (- 1 mm2) even though the cement thicknesses were different.
Cement selection is important for establishing effective bond strength to zirconia. Two types of dual-cured adhesive cement, Rely X UniCem and Panavia F 2.0, were used in this study. Rely X UniCem is a self-adhesive cement consisting of adhesive monomers with phosphoric acid groups. The phosphoric acid groups are thought to establish stable and durable bonding by achieving chemical bonds with metallic oxide on the zirconia surface.15 Panavia F 2.0 contains 10-MDP, an adhesive functional monomer, which forms chemical bonds between metallic oxide on the zirconia surface and the phosphate ester itself.12 Both cements are known to exhibit greater bond strengths with zirconia than conventional Bis-GMA cements.14 In contrast, Amaral and colleagues reported that a combination of 10-MDP-containing resin cement and air-abraded zirconia did not yield stable microtensile bond strength after thermocycling.16 Durable bond strength was only achieved by silanization of the zirconia surface following laboratory or chairside silica coating even though 10-MDP-containing resin cement was used. In the present study, Panavia F 2.0 resulted in significantly greater microtensile bond strength than Rely X UniCem regardless of whether or not the specimens were thermocycled (P<.05). Although Rely X UniCem resulted in about 50% mixed failures in the non-thermocycled group, the incidence decreased to 7% after thermocycling. The incidence of mixed failures for Panavia F 2.0 also decreased after thermocycling, although the rate (32 - 38%) was still greater than that of Rely X UniCem.
There are several experimental methods to evaluate the bond strength between resin and zirconia ceramic. These include: 1) building up resin cement directly on the zirconia surface using a glass cylinder 2) bonding a composite resin specimen to zirconia using resin cement, and 3) bonding dentin to zirconia using resin cement. Oyagüe et al.13 recommended using a resin cylinder rather than dentin to test the microtensile bond strength because the dentinal surface might have some unexpected microstructural variations which could lead to misleading results. In the present study, all specimens were debonded at the resin-zirconia interface, and not within dentin nor at the resindentin interface. This implies that the use of dentin can be an appropriate method to measure the resin-zirconia bond strength.
As mentioned previously, it is assumed that the decrease in bond strength after thermocycling was mainly due to hydrolytic degradation at the resin-zirconia interface. Therefore, future studies focusing on new surface-conditioning methods which could prevent hydrolytic degradation at the bonded interface should be designed to achieve durable resin-zirconia bonding.
Within the limitations of this study, the following conclusions may be drawn.
1. Difference in resin cement thickness had no significant effect on the microtensile bond strength between the resin cement and zirconia.
2. After 18,000 thermal cycles, the microtensile bond strength significantly decreased despite the application of adhesive monomer containing resin cement on the air-abraded zirconia surface.
3. The incidence of adhesive failure increased in all experimental groups after thermocycling.
Figures and Tables
Fig. 1
Schematic image of customized jig for zirconia-resin-tooth bonding. Two lateral fixation screws stabilized Tofflemire matrix bands. A vertical fixation screw immobilized the cut tooth specimen and maintained constant torque during polymerization of the resin cement.
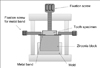
Fig. 2
Preparation of microbeams. A customized holding device was used to rotate the specimen 90 degrees, resulting in microbeams with a rectangular cut surface.
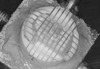
Fig. 3
Measurement of cement thickness. Microbeams with cement thicknesses of 40 (A) and 160 µm (B).
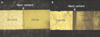
Fig. 4
SEM images of fractured non-thermocycled microbeams. The fractured surface reflects the mixed failure mode at the resin-zirconia interface (A: U40NTC; B: P160NTC).
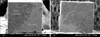
Fig. 5
SEM images of fractured microbeams which were thermally cycled 18,000 times. Both fractured surfaces represent adhesive failures at the resin-zirconia interface (A: U40TC, B: P40TC).
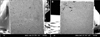
References
1. Ozcan M, Vallittu PK. Effect of surface conditioning methods on the bond strength of luting cement to ceramics. Dent Mater. 2003. 19:725–731.
2. Phark JH, Duarte S Jr, Blatz M, Sadan A. An in vitro evaluation of the long-term resin bond to a new densely sintered high-purity zirconium-oxide ceramic surface. J Prosthet Dent. 2009. 101:29–38.
3. Palacios RP, Johnson GH, Phillips KM, Raigrodski AJ. Retention of zirconium oxide ceramic crowns with three types of cement. J Prosthet Dent. 2006. 96:104–114.
4. Piconi C, Maccauro G. Zirconia as a ceramic biomaterial. Biomaterials. 1999. 20:1–25.
5. Kosmac T, Oblak C, Jevnikar P, Funduk N, Marion L. The effect of surface grinding and sandblasting on flexural strength and reliability of Y-TZP zirconia ceramic. Dent Mater. 1999. 15:426–433.
6. Janda R, Roulet JF, Wulf M, Tiller HJ. A new adhesive technology for all-ceramics. Dent Mater. 2003. 19:567–573.
7. Kern M, Wegner SM. Bonding to zirconia ceramic: adhesion methods and their durability. Dent Mater. 1998. 14:64–71.
8. Al-Dohan HM, Yaman P, Dennison JB, Razzoog ME, Lang BR. Shear strength of core-veneer interface in bi-layered ceramics. J Prosthet Dent. 2004. 91:349–355.
9. Oh WS, Shen C. Effect of surface topography on the bond strength of a composite to three different types of ceramic. J Prosthet Dent. 2003. 90:241–246.
10. Valandro LF, Della Bona A, Antonio Bottino M, Neisser MP. The effect of ceramic surface treatment on bonding to densely sintered alumina ceramic. J Prosthet Dent. 2005. 93:253–259.
11. Guazzato M, Albakry M, Quach L, Swain MV. Influence of surface and heat treatments on the flexural strength of a glass-infiltrated alumina/zirconia-reinforced dental ceramic. Dent Mater. 2005. 21:454–463.
12. Burke FJ, Fleming GJ, Nathanson D, Marquis PM. Are adhesive technologies needed to support ceramics? An assessment of the current evidence. J Adhes Dent. 2002. 4:7–22.
13. Oyagüe RC, Monticelli F, Toledano M, Osorio E, Ferrari M, Osorio R. Effect of water aging on microtensile bond strength of dual-cured resin cements to pre-treated sintered zirconium-oxide ceramics. Dent Mater. 2009. 25:392–399.
14. Wolfart M, Lehmann F, Wolfart S, Kern M. Durability of the resin bond strength to zirconia ceramic after using different surface conditioning methods. Dent Mater. 2007. 23:45–50.
15. Yang B, Barloi A, Kern M. Influence of air-abrasion on zirconia ceramic bonding using an adhesive composite resin. Dent Mater. 2010. 26:44–50.
16. Amaral R, Ozcan M, Valandro LF, Balducci I, Bottino MA. Effect of conditioning methods on the microtensile bond strength of phosphate monomer-based cement on zirconia ceramic in dry and aged conditions. J Biomed Mater Res B Appl Biomater. 2008. 85:1–9.
17. Aboushelib MN, Kleverlaan CJ, Feilzer AJ. Selective infiltration-etching technique for a strong and durable bond of resin cements to zirconia-based materials. J Prosthet Dent. 2007. 98:379–388.
18. Wegner SM, Gerdes W, Kern M. Effect of different artificial aging conditions on ceramic-composite bond strength. Int J Prosthodont. 2002. 15:267–272.
19. Lüthy H, Loeffel O, Hammerle CH. Effect of thermocycling on bond strength of luting cements to zirconia ceramic. Dent Mater. 2006. 22:195–200.
20. Leinfelder KF, Isenberg BP, Essig ME. A new method for generating ceramic restorations: a CAD-CAM system. J Am Dent Assoc. 1989. 118:703–707.
21. Molin MK, Karlsson SL, Kristiansen MS. Influence of film thickness on joint bend strength of a ceramic/resin composite joint. Dent Mater. 1996. 12:245–249.
22. Reich S, Wichmann M, Nkenke E, Proeschel P. Clinical fit of all-ceramic three-unit fixed partial dentures, generated with three different CAD/CAM systems. Eur J Oral Sci. 2005. 113:174–179.
23. Kohorst P, Brinkmann H, Dittmer MP, Borchers L, Stiesch M. Influence of the veneering process on the marginal fit of zirconia fixed dental prostheses. J Oral Rehabil. 2010. 37:283–291.
24. Wettstein F, Sailer I, Roos M, Hmmerle CH. Clinical study of the internal gaps of zirconia and metal frameworks for fixed partial dentures. Eur J Oral Sci. 2008. 116:272–279.
25. Sailer I, Fehér A, Filser F, Gauckler LJ, Lüthy H, Hämmerle CH. Five-year clinical results of zirconia frameworks for posterior fixed partial dentures. Int J Prosthodont. 2007. 20:383–388.
26. Feilzer AJ, De Gee AJ, Davidson CL. Setting stress in composite resin in relation to configuration of the restoration. J Dent Res. 1987. 66:1636–1639.