Abstract
PURPOSE
The purpose of this study was to analyze the effects of two different implant surface treatments on initial bone connection by comparing the Removal Torque Values (RTQs) at 7 and 10 days after chemically modified, sandblasted, large-grit and acid-etched (modSLA), and Laser-etched (LE) Ti implant placements.
MATERIALS AND METHODS
Twenty modSLA and 20 LE implants were installed on the left and right tibias of 20 adult rabbits. RTQs were measured after 7 and 10 days in 10 rabbits each. Scanning electron microscope (SEM) photographs of the two implants were observed by using Quanta FEG 650 from the FEI company (Hillsboro, OR, USA). Analyses of surface elements and components were conducted using energy dispersive spectroscopy (EDS, Horiba, Kyoto, Japan).
RESULTS
The mean RTQs were 12.29 ± 0.830 and 12.19 ± 0.713 Ncm after 7 days (P=.928) and 16.47 ± 1.324 and 16.17 ± 1.165 Ncm after 10 days (P=.867) for LE and modSLA, respectively, indicating no significant inter-group differences. Pore sizes in the LE were 40 µm and consisted of numerous small pores, whereas pore sizes in the modSLA were 5 µm. In the EDS analysis, Ti, O, and C were the only three elements found in the LE surfaces. Na, Ca, Cl, and K were also observed in modSLA, in addition to Ti, O, and C.
Dental implants have been successfully used in recent decades as an alternative treatment method for missing teeth to attain dental function.
Brånemark et al. suggested that implants should be given a healing period of 3 – 6 months without load after placement. During this period, prevention of micro-agitation of the implant, promotion of osseointegration, and prevention of infiltration of soft tissues were suggested to increase the clinical success rate, which became a principle of implant treatment for a long time.12
However, many efforts have been made recently to reduce the conventional treatment period and the discomfort of patients during the healing period of implant placement. In addition, many implant surface treatments have been studied to accelerate osseointegration between bone and implant.3456
The surface structure of the implant is one of the main factors that affect osseointegration, by which the implant directly connects with the bone.78 Various methods of implant surface treatment have been developed from simply imparting roughness to a machined surface or changing the surface microstructure to providing a chemically more active surface.3910
According to recent studies, SLA surface implants represent osseointegration at an earlier state based on the various sizes of implants, implant surface roughness, and excellent chemical properties.111213
According to Ferguson et al., based on the excellent surface characteristics and hydrophilic properties, SLA surface implants are effective in early cellular reactions at the early stage of osseointegration.14
SLA surface implants exhibit two types of surface properties known as macro and micro at the same time. The macro structure formed through the sandblasting process can secure the mechanical bonding force, and the micro structure formed by acid etching has an advantage of promoting cell activity.15
However, SLA surface implants based on sandblasted, large-grit, and acid-etched surfaces go through a cleaning process such as acid etching to remove particles remaining on the surface during the blasting process. However, previous reports pointed out that some particles may remain after acid etching and interfere with osseointegration.16
On the other hand, the laser-etching (laser treatment) surface treatment method is a noncontact method. This method has a high purity surface that does not contain impurities and has sufficient surface roughness to enhance the bone-binding force. TiN formed on the surface promotes bone formation by augmenting biocompatibility.1718
In addition, laser-treated implants increase corrosion resistance and hardness, thereby increasing osseointegration. Moreover, these implants show 2.5 times higher RTQ value than machined surface implant.18 According to Lee, no significant difference in RTQ was found between the SLActive and laser-etched (LE) implants after 4 weeks of implant placement.16
The purpose of this study was to analyze the effects of two different implant surface treatments on initial bone connection by comparing the RTQs at 7 and 10 days after chemically modified SLA (modSLA) and LE implant placements.
The implants were machined-surfaced titanium screws (grade 4) with a diameter of 3.75 mm and a length of 4 mm. LE implants (CSM Implant Co., Ltd., Daegu, Korea) were used as an experimental group; and modified SLA implants (CSM Implant Co., Ltd., Daegu, Korea), as a control group.
The surface of the experimental group was irradiated with Nd:YAG laser (Jenoptic Laser Optik system GmbH, Jena, Germany) by moving the glass fiber to the linear motion (Wavelength: 15 kHz, rated output: 10 W, pulse width: 2 µsec). The distance from the glass fiber to the most accurate focus was determined, and the laser was irradiated uniformly (focus size: diameter of 400 µm).
Implant surfaces were irradiated without contact, and all surfaces were treated uniformly and then acid-treated with HCl/H2SO4. The surface of the control implants was sandblasted and acid-treated at 80° with HCl. After washing with distilled water, they were stored in saline on a clean bench.
Scanning electron microscope (SEM) photographs of the two implants were observed at magnifications of ×1,000, ×2,500, ×5,000, ×10,000 by using Quanta FEG 650 from the FEI company (Hillsboro, OR, USA). Analyses of surface elements and components were conducted using energy dispersive spectroscopy (EDS, Horiba, Kyoto, Japan).
The roughness of the surfaces of the experimental and control groups were measured using a confocal laser scanning microscopy (Carl Zeiss, LSM 700, Oberkochen, Germany). Surface roughness was measured at three points in screw thread and three points in screw root, and the centerline average roughness (RSa) and square root mean roughness (RSq) were measured.
Twenty New Zealand rabbits were used in this experiment. The mean weight of the rabbits in the experiment group was 3.5 kg. The experiment was approved by the Animal Care and Use Committee of Kyung-Pook National University (KNU 2017-0045).
Anesthesia was given to the rabbits via intramuscular injection of 2.0 mL per kg of tiletamine/zolazepam (Zoletil50, Virbac Korea, Seoul, Korea) and 5 mg/kg xylazine (Rompun, Bayer Korea, Seoul, Korea). In addition, local anesthesia was performed with subcutaneous injection of 2% lidocaine before the operation.
Before the surgery, hair on the tibia was removed and the skin was cleaned with iodine and 75% ethanol solution. The tibia was then exposed. After bone exposure, each implant was placed in a tibia by using the existing implant placement method, in accordance with the manufacturer's instruction. In the experimental group, the LE implant was placed in the tip of the left tibia, and the control modSLA implant was placed in the tip of the right tibia and sutured with a 4-0 absorbable suture.
After the operation, the rabbits were isolated for 24 hours to provide a sufficient recovery environment. In addition, 0.3 mL of enrofloxacin (Baytril, Bayer Korea, Seoul, Korea) and 0.3 mL of methanpyrone (Novin-50, Bayer Korea, Seoul, Korea) and butaphosphan (Catosal, Bayer Korea, Seoul, Korea) were injected intramuscularly for 3 days to help the rabbits recover and prevent infection.
The insertion torque was measured when the implant was placed. 7 days after the first operation, 10 of the 20 rabbits were killed and the RTQ was measured. 10 days after the first operation, the remaining 10 rabbits were killed and the RTQ was measured.
The measuring instrument used was a digital MGT-12 torque meter (Mark-10 Corporation, Copiague, NY, USA). The insertion torque and RTQ were measured in Ncm units. A specially designed connector was used to connect the torque meter to the implant.
The program used for statistical analysis was IBM SPSS Statistics ver. 20.0 (IBM Co., Armonk, NY, USA), and the measured data were compared using a T test. All data are presented as mean ± standard deviation, with a significance level of P ≤ .05.
In the experimental and control groups, the surfaces of the implants were observed at ×1,000, ×2,500, ×5,000, ×10,000 magnification under a field-emission scanning electron microscope before surgery. Porous structures were observed throughout the surface of both types of implants. In the case of the LE implant, a large porous structure of 40 µm and a small porous structure of approximately 1 µm were observed in the structure. A porous structure of approximately 5 µm was observed for the modSLA implant (Fig. 1).
In the EDS analysis, Ti, O, and C were the only three elements found in the laser-treated implant surfaces. Na, Ca, Cl, and K were also observed in modSLA, in addition to Ti, O, and C (Fig. 2, Table 1).
The mean centerline roughness (RSa) of the experimental group (LE implants) was 11.16 ± 1.947 µm, and the mean square roughness (RSq) was 13.95 ± 2.254 µm. The mean centerline roughness (RSa) of the control group (modSLA implants) was 1.28 ± 0.646 µm, and the mean square roughness (RSq) was observed to be 1.65 ± 0.846 µm (Fig. 3, Table 2).
The mean insertion torques of the LE and modSLA implants were 10.95 ± 0.654 Ncm and 11.11 ± 2.911 Ncm, respectively. No significant differences were found between the two groups (P = .741 > .05). After 7 days of implantation, the mean insertion torque of the LE implant was 12.29 ± 0.830 Ncm, and the mean RTQ of the modSLA implant was 12.19 ± 0.713 Ncm. No significant difference was found between the two groups (P = .928 > .05).
10 days after implantation, the mean RTQs of the LE and modSLA implants were 16.47 ± 1.324 Ncm and 16.17 ± 1.165 Ncm, respectively. No significant difference was found between the two groups (P = .867 > .05; Table 3).
In this study, the degree of osseointegration of implants was measured through a removal torque test, which is one of the basic methods to diagnose the degree of osseointegration of implants. It has been proposed and used by Roberts et al. It is a method to measure the critical torque value at which bone-implant contact is broken.19 In addition, Johansson et al. suggested that removal torque is closely related to the contact area between the implant and bone, and the amount of bone embedded in the implant threads, and that a large removal torque implies relatively more osteosynthesis.20212223
According to Sennerby et al., no significant differences in removal torque values were found at 6 weeks, 3 months, and 6 months for screw-shaped implants tested in rabbit tibia.24 According to Albreksson et al., the tibia of the rabbit had complete healing after 6 weeks of implant placement; thereafter, no change was observed in removal torque. In humans, approximately 3 – 4 months were required for complete healing.25
Therefore, the implant bone growth process of the osseointegrated implants took 2 or 3 times more time than that of the rabbits. In other words, a week of bone growth in rabbits is about 2 to 3 weeks in humans, and 10 days of bone growth in rabbits is about 3 to 4 weeks in humans.
According to Lee, SLActive and LE implants placed in the rabbit tibia showed that the SEM findings of the bone attached to the implant surface at the time of removal 3 weeks after implantation showed a complete new bone shape.16
In this experiment, we measured and analyzed the degree of relative osseointegration according to the method of implant surface treatment in the early loading period by measuring the removal torque value 7 and 10 days after placing modSLA and LE implants in rabbit tibia. As a result, the mean removal torque value was 12.29 ± 0.830 Ncm for the LE implant and 12.19 ± 0.713 Ncm for the modSLA implant at 7 days after implantation. No significant differences were found between the two groups.
The mean removal torque values were 16.47 ± 1.324 Ncm and 16.17 ± 1.165 Ncm in the LE and control groups, respectively, even after 10 days of implant removal. No significant differences were found between the two groups. In other words, no significant clinical difference was found when comparing the osseointegration of the two implants 7 and 10 days after implantation in the rabbit tibia, that is, during the period corresponding to early loading in humans.
In comparison with the modSLA implants, which are currently the most popular and well-known surface treatments, the LE surface treatment method has a similar effect on the initial osseointegration and is a clinically effective surface treatment method.
The implants have a period of reduced overall stability until the biological stability increases as the mechanical stability decreases after implantation.26 In this experiment, the removal torque values of both types of implants increased after 7 and 10 days, in comparison with the insertion torque values. This implies that both types of implants have gone beyond the stability dip period, where concern emerges about the possible decrease in the initial stability of bone formation after 7 days of implant placement. Moreover, this indicates that early bone adhesion progresses rapidly.
One of the methods for increasing the biocompatibility of implants is to increase surface roughness. The surface roughness of an implant affects the fusion of bone to the surface of the implant and cell differentiation.2728 The surface roughness of LE implants has been reported to have a higher surface roughness value than the control implants.17293031
In this experiment, the mean centerline roughness (RSa) in the experimental group (LE implants) was 11.16 ± 1.947 µm, and the mean square roughness (RSq) was 13.95 ± 2.254 µm. The mean centerline roughness (RSa) in the control group (modSLA implants) was 1.28 ± 0.646 µm, and the mean square roughness (RSq) was observed to be 1.65 ± 0.846 µm. In other words, the surface roughness of the LE implant was significantly higher than that of the modSLA implant.
These results may lead to an increase in the surface area of the implant surface, which may have a considerable influence on the initial bone connection of the implant. In view of these excellent surface characteristics, the LE implants are considered to have a high surface treatment potential in the future.
In the case of the LE implant, a large porous structure of 40 µm and a small porous structure of approximately 1 µm were observed in the structure. A porous structure of approximately 5 µm was observed for the modSLA implant.
In particular, the surface area of the LE implants was significantly increased by the porous structure formed by the laser etching surface treatment process and by the microporous structures forming the porous structure.
This porous structure plays a major role in osseointegration by contributing to the increase in the surface area and mechanical bonding of bone and implant.
The EDS surface analysis revealed that only three elements of Ti, O, and C were observed on the surface of the LE implant, whereas Na, Ca, Cl, and K were observed in addition to Ti, O, and C on the surface of the modSLA implant. This means that an oxide layer was formed on both the experimental and control implant surfaces.
In addition, the elements observed on the modSLA surface, such as Na, Ca, Cl, and K, facilitate the osseointegration of the implant and bone by improving the wettability of the implant surface. Based on this increased hydrophilicity, modSLA implant surfaces can absorb blood and proteins more quickly, which is a very positive factor in initial osseointegration. Moreover, the modSLA implant in saline solution has the advantage of maintaining surface hydrophilicity, preventing the surface of the implant from being contaminated from the −C and −CH groups in the air, and preventing the reduction of surface energy.32
We observed no significant differences in biomechanical bond strength to bone between the LE and modified SLA implants during the early stage of osseointegration.
On the basis of the above-mentioned experimental results, the LE implant can be considered an excellent surface treated implant in addition to the modSLA implant, which is currently considered to be the most stable implant, and can be applied to the early loading of the prosthesis clinically.
References
1. Albrektsson T, Brånemark PI, Hansson HA, Lindström J. Osseointegrated titanium implants. Requirements for ensuring a long-lasting, direct bone-to-implant anchorage in man. Acta Orthop Scand. 1981; 52:155–170. PMID: 7246093.
2. Brånemark PI, Adell R, Breine U, Hansson BO, Lindström J, Ohlsson A. Intra-osseous anchorage of dental prostheses. I. Experimental studies. Scand J Plast Reconstr Surg. 1969; 3:81–100. PMID: 4924041.
3. Smeets R, Stadlinger B, Schwarz F, Beck-Broichsitter B, Jung O, Precht C, Kloss F, Gröbe A, Heiland M, Ebker T. Impact of dental implant surface modifications on osseointegration. Biomed Res Int. 2016; 2016:6285620. PMID: 27478833.


4. Chen CJ, Ding SJ, Chen CC. Effects of surface conditions of titanium dental implants on bacterial adhesion. Photomed Laser Surg. 2016; 34:379–388. PMID: 27454339.


5. Shah FA, Johansson ML, Omar O, Simonsson H, Palmquist A, Thomsen P. Laser-modified surface enhances osseointegration and biomechanical anchorage of commercially pure titanium implants for bone-anchored hearing systems. PLoS One. 2016; 11:e0157504. PMID: 27299883.


6. Park SH, Park KS, Cho SA. Comparison of removal torques of SLActive implant and blasted, laser-treated titanium implant in rabbit tibia bone healed with concentrated growth factor application. J Adv Prosthodont. 2016; 8:110–115. PMID: 27141254.


7. Junker R, Dimakis A, Thoneick M, Jansen JA. Effects of implant surface coatings and composition on bone integration: a systematic review. Clin Oral Implants Res. 2009; 20:185–206. PMID: 19663965.


8. Wong M, Eulenberger J, Schenk R, Hunziker E. Effect of surface topology on the osseointegration of implant materials in trabecular bone. J Biomed Mater Res. 1995; 29:1567–1575. PMID: 8600147.


9. von Wilmowsky C, Moest T, Nkenke E, Stelzle F, Schlegel KA. Implants in bone: part I. A current overview about tissue response, surface modifications and future perspectives. Oral Maxillofac Surg. 2014; 18:243–257. PMID: 23435578.


10. Dohan Ehrenfest DM, Coelho PG, Kang BS, Sul YT, Albrektsson T. Classification of osseointegrated implant surfaces: materials, chemistry and topography. Trends Biotechnol. 2010; 28:198–206. PMID: 20116873.


11. Zinelis S, Silikas N, Thomas A, Syres K, Eliades G. Surface characterization of SLActive dental implants. Eur J Esthet Dent. 2012; 7:72–92. PMID: 22319766.
12. Buser D, Broggini N, Wieland M, Schenk RK, Denzer AJ, Cochran DL, Hoffmann B, Lussi A, Steinemann SG. Enhanced bone apposition to a chemically modified SLA titanium surface. J Dent Res. 2004; 83:529–533. PMID: 15218041.


13. Cochran DL, Buser D, ten Bruggenkate CM, Weingart D, Taylor TM, Bernard JP, Peters F, Simpson JP. The use of reduced healing times on ITI implants with a sandblasted and acid-etched (SLA) surface: early results from clinical trials on ITI SLA implants. Clin Oral Implants Res. 2002; 13:144–153. PMID: 11952734.
14. Ferguson SJ, Broggini N, Wieland M, de Wild M, Rupp F, Geis-Gerstorfer J, Cochran DL, Buser D. Biomechanical evaluation of the interfacial strength of a chemically modified sandblasted and acid-etched titanium surface. J Biomed Mater Res A. 2006; 78:291–297. PMID: 16637025.


15. Marin C, Bonfante EA, Granato R, Suzuki M, Granjeiro JM, Coelho PG. The effect of alterations on resorbable blasting media processed implant surfaces on early bone healing: a study in rabbits. Implant Dent. 2011; 20:167–177. PMID: 21448026.


16. Lee JT, Cho SA. Biomechanical evaluation of laser-etched Ti implant surfaces vs. chemically modified SLA Ti implant surfaces: Removal torque and resonance frequency analysis in rabbit tibias. J Mech Behav Biomed Mater. 2016; 61:299–307. PMID: 27093590.


17. Gaggl A, Schultes G, Müller WD, Kärcher H. Scanning electron microscopical analysis of laser-treated titanium implant surfaces-a comparative study. Biomaterials. 2000; 21:1067–1073. PMID: 10768759.


18. Cho SA, Jung SK. A removal torque of the laser-treated titanium implants in rabbit tibia. Biomaterials. 2003; 24:4859–4863. PMID: 14530083.


19. Roberts WE, Smith RK, Zilberman Y, Mozsary PG, Smith RS. Osseous adaptation to continuous loading of rigid endosseous implants. Am J Orthod. 1984; 86:95–111. PMID: 6589962.


20. Johansson C, Albrektsson T. Integration of screw implants in the rabbit: a 1-year follow-up of removal torque of titanium implants. Int J Oral Maxillofac Implants. 1987; 2:69–75. PMID: 3481352.
21. Johansson CB, Albrektsson T. A removal torque and histomorphometric study of commercially pure niobium and titanium implants in rabbit bone. Clin Oral Implants Res. 1991; 2:24–29. PMID: 1807419.


22. Johansson CB, Sennerby L, Albrektsson T. A removal torque and histomorphometric study of bone tissue reactions to commercially pure titanium and Vitallium implants. Int J Oral Maxillofac Implants. 1991; 6:437–441. PMID: 1820312.
23. Ivanoff CJ, Sennerby L, Lekholm U. Influence of mono- and bicortical anchorage on the integration of titanium implants. A study in the rabbit tibia. Int J Oral Maxillofac Surg. 1996; 25:229–235. PMID: 8872230.
24. Sennerby L, Thomsen P, Ericson LE. A morphometric and biomechanic comparison of titanium implants inserted in rabbit cortical and cancellous bone. Int J Oral Maxillofac Implants. 1992; 7:62–71. PMID: 1398826.
25. Albrektsson T, Brånemark PI, Eriksson A, Lindström J. The preformed autologous bone graft. An experimental study in the rabbit. Scand J Plast Reconstr Surg. 1978; 12:215–223. PMID: 368971.


26. Raghavendra S, Wood MC, Taylor TD. Early wound healing around endosseous implants: a review of the literature. Int J Oral Maxillofac Implants. 2005; 20:425–431. PMID: 15973954.
27. Brett PM, Harle J, Salih V, Mihoc R, Olsen I, Jones FH, Tonetti M. Roughness response genes in osteoblasts. Bone. 2004; 35:124–133. PMID: 15207748.


28. Bagno A, Di Bello C. Surface treatments and roughness properties of Ti-based biomaterials. J Mater Sci Mater Med. 2004; 15:935–949. PMID: 15448401.


29. Kang SH, Cho SA. Comparison of removal torques for lasertreated titanium implants with anodized implants. J Craniofac Surg. 2011; 22:1491–1495. PMID: 21778844.


30. Faeda RS, Tavares HS, Sartori R, Guastaldi AC, Marcantonio E Jr. Biological performance of chemical hydroxyapatite coating associated with implant surface modification by laser beam: biomechanical study in rabbit tibias. J Oral Maxillofac Surg. 2009; 67:1706–1715. PMID: 19615586.


31. Rong M, Zhou L, Gou Z, Zhu A, Zhou D. The early osseointegration of the laser-treated and acid-etched dental implants surface: an experimental study in rabbits. J Mater Sci Mater Med. 2009; 20:1721–1728. PMID: 19291368.


32. Donos N, Hamlet S, Lang NP, Salvi GE, Huynh-Ba G, Bosshardt DD, Ivanovski S. Gene expression profile of osseointegration of a hydrophilic compared with a hydrophobic microrough implant surface. Clin Oral Implants Res. 2011; 22:365–372. PMID: 21561478.


Fig. 1
Scanning electron microscopy image of LE and mod SLA implant surfaces. (A) ×1,000, (B) ×1,000, (C) ×2,500, (D) ×2,500, (E) ×5,000, (F) ×5,000, (G) ×10,000, (H) ×10,000.
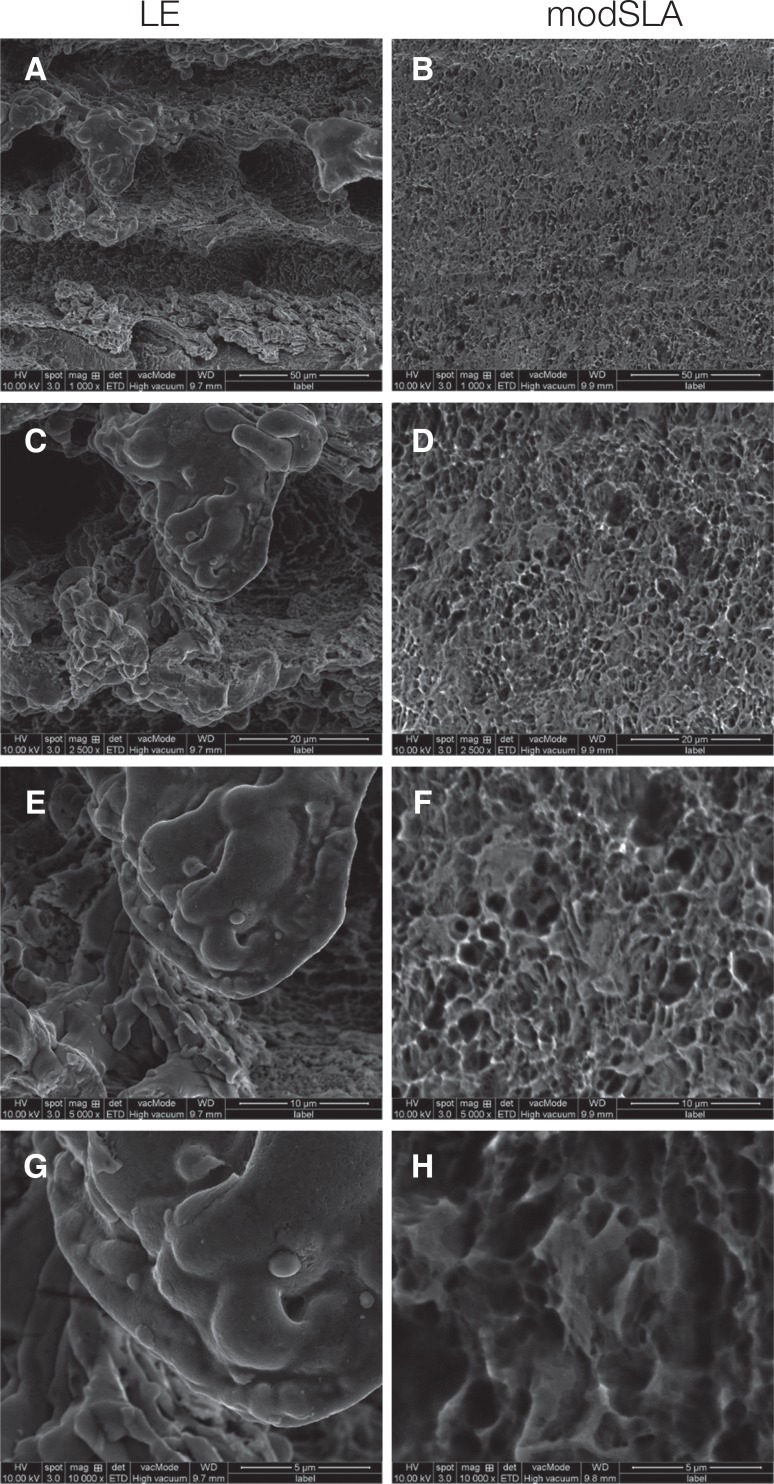
Fig. 2
(A) LE implant EDS spectrum. Ti, O, and C were the only three elements found in the LE implant surfaces. (B) modSLA implant EDS spectrum. Na, Ca, Cl, and K were also observed in modSLA, in addition to Ti, O, and C.
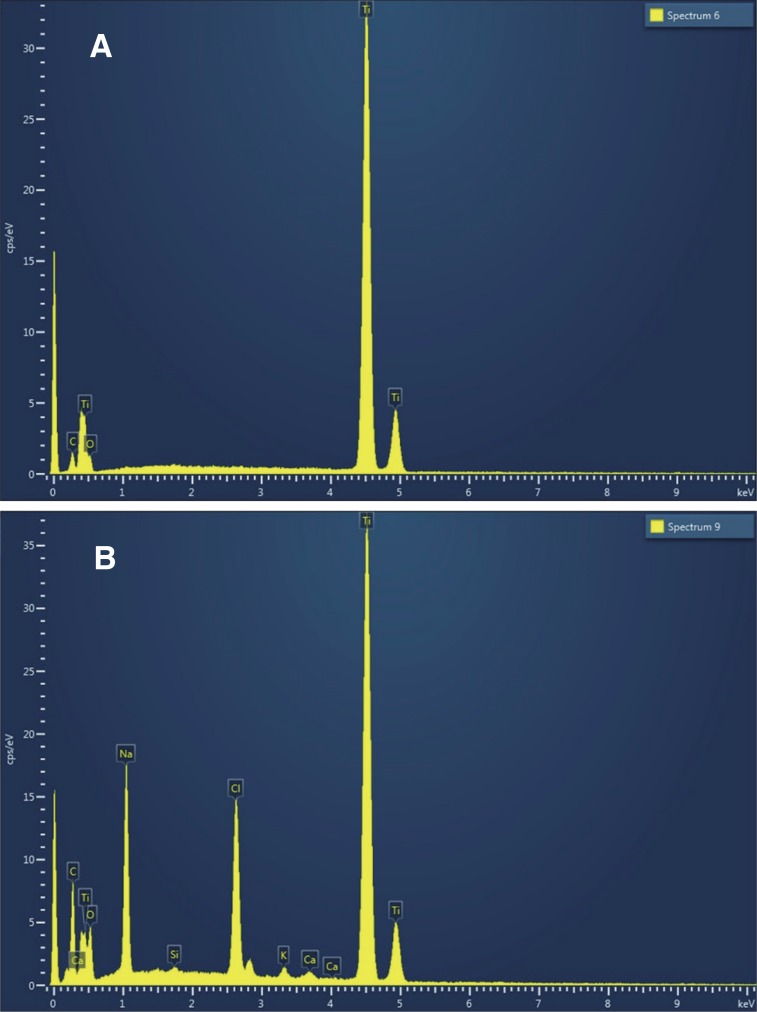
Fig. 3
Confocal laser scanning microscopy image: (A) LE implant surface; (B) modSLA implant surface.

Table 1
Surface element analysis of the LE and modSLA implants
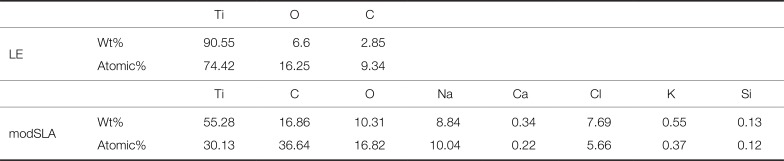
Ti | O | C | ||
---|---|---|---|---|
LE | Wt% | 90.55 | 6.6 | 2.85 |
Atomic% | 74.42 | 16.25 | 9.34 |
Ti | C | O | Na | Ca | Cl | K | Si | ||
---|---|---|---|---|---|---|---|---|---|
modSLA | Wt% | 55.28 | 16.86 | 10.31 | 8.84 | 0.34 | 7.69 | 0.55 | 0.13 |
Atomic% | 30.13 | 36.64 | 16.82 | 10.04 | 0.22 | 5.66 | 0.37 | 0.12 |
Table 2
Surface roughness of LE and modSLA implant
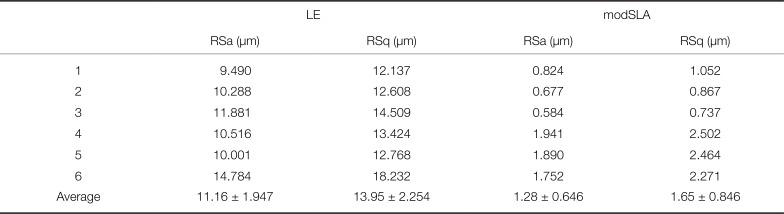
Table 3
Insertion torque and Removal torque of LE and modSLA implant
