Abstract
PURPOSE
The aim of this in vitro study was to investigate the fracture resistance under chewing simulation of implant-supported posterior restorations (crowns cemented to hybrid-abutments) made of different all-ceramic materials.
MATERIALS AND METHODS
Monolithic zirconia (MZr) and monolithic lithium disilicate (MLD) crowns for mandibular first molar were fabricated using computer-aided design/computer-aided manufacturing technology and then cemented to zirconia hybrid-abutments (Ti-based). Each group was divided into two subgroups (n=10): (A) control group, crowns were subjected to single load to fracture; (B) test group, crowns underwent chewing simulation using multiple loads for 1.2 million cycles at 1.2 Hz with simultaneous thermocycling between 5℃ and 55℃. Data was statistically analyzed with one-way ANOVA and a Post-Hoc test.
RESULTS
All tested crowns survived chewing simulation resulting in 100% survival rate. However, wear facets were observed on all the crowns at the occlusal contact point. Fracture load of monolithic lithium disilicate crowns was statistically significantly lower than that of monolithic zirconia crowns. Also, fracture load was significantly reduced in both of the all-ceramic materials after exposure to chewing simulation and thermocycling. Crowns of all test groups exhibited cohesive fracture within the monolithic crown structure only, and no abutment fractures or screw loosening were observed.
CONCLUSION
When supported by implants, monolithic zirconia restorations cemented to hybrid abutments withstand masticatory forces. Also, fatigue loading accompanied by simultaneous thermocycling significantly reduces the strength of both of the all-ceramic materials. Moreover, further research is needed to define potentials, limits, and long-term serviceability of the materials and hybrid abutments.
With the availability of computer-aided design/computer-aided manufacturing (CAD/CAM) technique and the introduction of the high strength all-ceramic materials, the tendency towards replacing the metal-ceramic restorations with the highly aesthetic all-ceramic materials is increasing.12 While the use of some all-ceramic materials has been narrowed down mainly due to inaccurate fitting3, the use of zirconia and lithium disilicate ceramics has increased in recent years. Restorations made from these materials have demonstrated successful short- and medium-term survival rates under clinical performance.456
Titanium has been the popular option for abutments in the posterior region because of its favourable mechanical properties.78 However, there is also a trend to substitute titanium with all-ceramic materials, i.e. Al2O3 and ZrO2, to provide patients with a more aesthetically pleasing restoration.910 In both in vitro and in vivo studies, zirconia has been investigated as an abutment material and has shown both strength and biocompatibility needed for an abutment.111213 In addition, zirconia abutments can be customized and fabricated using the CAD/CAM technology, adding simplicity, efficiency and reducing cost and time.1415
The hybrid-abutment approach is a combination of strength and aesthetics. However, it is still relatively new in implant dentistry. The all-ceramic hybrid-abutment crown system is made of three components: a) an all-ceramic crown, b) an all-ceramic abutment, and c) a Ti-base abutment. Clinical studies by Lin et al.16 and Hornbrook17 and Traini et al.18 suggested the use of hybrid abutments as an adequate approach to enhance aesthetics in the anterior region for single all-ceramic crowns. The combination of monolithic lithium disilicate crowns with a Ti-base (hybrid crowns) was also considered a reliable and cost effective approach in the posterior region.19
Few in vitro studies have looked at the use of hybrid abutments and their influence on the fracture resistance of all-ceramic crown.2021222324 Stimmelmayr et al.24 reported that titanium abutments and hybrid abutments exhibited comparable mechanical properties. On the other hand, other studies showed that crown structure,22 all-ceramic crown material22 and the abutment material,20 and manufacturer and design23 all have proven to have an influence on the fracture resistance of all-ceramic crowns.
Current literature provides only limited information on the fracture resistance of crowns supported by hybrid-abutments. Therefore, the aim of this study was to investigate fatigue resistance and post-fatigue fracture load of all-ceramic crowns in hybrid-abutment system. This study adopted a clinically relevant mechanical testing to investigate the fracture resistance of implant-supported posterior restorations (crowns cemented to hybrid-abutment) under chewing simulation. The null hypothesis was that there would be no difference in fracture load between the zirconia and lithium disilicate crowns when cemented to a zirconia hybrid-abutment and supported by dental implants. We also hypothesized that fatigue, manifested by chewing simulation and thermal cycling, would have no significant effect on the all-ceramic materials fracture load.
Forty Ankylos implants (Ankylos C/X, DENTSPLY-Friadent GmbH, Mannheim, Germany) with a diameter of 5.5 mm, and Internal Ankylos compatible titanium base (Ti-Base) abutments (Dess, Dental Smart Solutions, Montcada, Spain) of 1.00 mm hex screw, 4 mm height, and 0° angulations were used for this study. The forty implants were divided into two groups (n = 20) according to the monolithic all-ceramic crown material to be tested, namely monolithic zirconia (MZr) and monolithic lithium disilicate (MLD).
CAD/CAM technology was used for the fabrication of both the zirconia abutments and the monolithic all-ceramic crowns. Using a dental laboratory scanner (3Shape, Copenhagen, Denmark), AnkylosScanBase was used to obtain the Ti-Base geometry needed to design Zr abutments (Zenostar, Ivoclar Vivadent, Lichtenstein, Germany) with 1.0 mm depth shoulder. With the split file technology, a monolithic crown was also designed according to the manufacturer's recommendations for thickness of full contour crowns (1.0 mm circular, 1.5 mm occlusal). The CAD file was transferred to a 5-axis milling machine (ZENOTEC select, Wieland Dental, Lindenstraße, Germany) to mill 40 Zr abutments and 20 MZr crowns using pre-sintered Zr discs (Zenostar Zr, Wieland Dental, Germany). A wet milling machine (ZENOTEC select hybrid, Wieland Dental, Lindenstraße, Germany) was used to mill the 20 MLD crowns (IPS e.max-CAD for Zenotec, Wieland Dental, Germany). Zr structures (abutments & MZr) were sintered in a Programat S1 furnace (Ivoclar Vivadent, Schaan, Liechtenstein) (Fig. 1), while MLD crowns were crystallized in a Programat EP 3010 furnace (Ivoclar Vivadent, Schaan, Liechtenstein). Both the sintering and the crystallization conditions are listed in Table 1, Table 2. Upon completion of sintering and crystallization, abutments and monolithic crowns were checked for fitting followed by glazing of the crowns according to the manufacturer's instructions.
To prepare the crowns for chewing simulation, specimen holders were fabricated to ensure fitting to the chewing simulators' sample cup and to standardize the positioning of the implants across all the test groups (Fig. 2). To position implants and create a negative replica of the sample cup, implants were screwed into a heavy putty (Coltene Whaledent, Altstätten, Switzerland) to the desired perpendicular position and to the horizontal plane up to the first thread in order to simulate clinical procedures. Afterwards, the negative replica of Ti-Base abutment screwed to implant were positioned in a cup, and silicone duplicate material (Exaktosil N21, Bredent) was poured to create the positive replica of the sample cup and was left to set in a pressure pot to avoid porosity. Finally, acrylic resin (Palapress vario, Heraeus Kulzer, Wehrheim, Germany) was poured in the mold and upon setting was checked in the original chewing simulation sample cup for fitting. The base was made using acrylic resin; it has a modulus of elasticity of approximately 12 GPa, which is similar to that of human bone (18 GPa).25
To assemble all components, Ti-Base abutments were screw tightened on the implants with a torque wrench driver (Dentsply-Friadent GmbH, Mannheim, Germany) to 20 N/cm. The abutment access hole was filled with a temporary restorative material (Fermit N; Ivoclar Vivadent AG, Schaan, Liechtenstein). All Zr abutments were then cemented to the Ti-Base abutment using a self-curing dental luting composite (Multilink Hybrid Abutment, Ivoclar Vivadent, Schaan, Liechtenstein) according to the manufacturer's instructions. 24 hours later, both MZr and MLD crowns were cemented to the Zr abutments using Multilink Automix (Ivoclar Vivadent, Schaan, Liechtenstein) according to the manufacturer's instructions. All specimens were stored at 37℃ in distilled water for a minimum of 7 days prior to testing to ensure overall hydration of both the cement and the embedding material.26
Each group was divided into two subgroups (n = 10): a) in control group, crowns were subjected to single load to fracture (SLF) using a universal testing machine (Model LRX; Lloyds Instrument, West Sussex, UK) and b) crowns underwent fatigue by means of chewing simulation (CS) (CS-4.8; SD Mechatronik GmbH, Feldkirchen-Westerham, Germany) for 1.2 million cycles prior to the SLF test.
Crowns underwent CS for 1,200,000 cycles with a 6-mm diameter stainless steel spherical indenter, according to the following protocol; 50 N for 250,000 cycles, followed by 100 N for 500,000 cycles, and finally 50 N for another 450,000 cycles with a loading frequency of 1.2 Hz. This loading protocol simulates 5 clinical years; according to previous studies, 250,000 cycles were used to simulate one year of clinical service.25 To simulate natural masticatory function, articulating paper was used to position indenters 0.5 mm lingual to the disto-buccal cusp tip and sliding 0.3 mm lingual27 to the central fossa with a mouth opening of 6 mm. During testing, each crown was subjected to simultaneous thermal cycling between 5℃ and 55℃ in distilled water resulting in 5118 thermal cycles with 60 s dwell time for each cycle and 15 s pause time to empty the chambers. Each crown was checked for any cracks, chipping or fractures at the end of each loading stage using an endodontic optical microscope at 12×.
A jig with a sample cup similar to that of the chewing simulator was specifically designed for the SLF testing (Fig. 3A) to ensure stability of the samples during testing. All crowns of the control group, as well as those surviving CS, were then subjected to SLF test in the universal testing machine (Lloyds Instrument Model LRX, Fareham, England). Load was vertically applied at the triangular ridges of both lingual cusps and disto-buccal cusps using 6-mm diameter spherical stainless steel indenter at a crosshead speed of 1 mm/min28 until failure (Fig. 3B). The fracture load of SLF test for all the groups were recorded in N.
One crown from each group was randomly selected to be examined using Scanning Electron Microscopy (SEM) (Joel, JCM-5000 NeoScope, Tokyo, Japan) to evaluate fractured surfaces. Crowns were sputter coated with gold (Leica EM SCD050, Wetzlar, Germany) to a thickness of approximately 15 nm prior to imaging.
Data was analyzed using the SPSS statistical software (version 22.0, IBM, Chicago, IL, USA). All-ceramic materials and testing regimes, as well as the loads at fracture for each group were registered and descriptive statistics (mean and standard deviation (SD)) was performed. To evaluate statistical significance among the groups, a one-way analysis of variance (ANOVA) was conducted, followed by LSD Post-Hoc test (two-tailed). All the statistical analysis was performed with significance level set at 5%.
This study showed that all crowns (MZr and MLD) survived the CS testing, resulting in 100% survival rate. However, wear facets were observed in all crowns at the occlusal contact point (Fig. 4). Not being the focus of this study, wear facets did not undergo further investigations.
Fracture load mean and SD of all groups are presented in Fig. 5. Generally, the mean fracture loads of MLD crowns were lower than those of the MZr group. In each ceramic group, the un-fatigued crowns had higher fracture load mean than that of the fatigued crowns; the mean fracture load of the MZr control group was 3929.5 ± 491.4 compared to 3131.5 ± 714.3 of the fatigued group. MLD control group and fatigued MLD recorded mean fracture loads of 2077.4 ± 99.6 and 1646.2 ± 211.7, respectively.
One-way ANOVA analysis showed significant differences between MZr and MLD groups and within the subgroups. LSD Post-Hoc tests showed a significant difference in fracture load between MZr control and MLD control (P ≤ .05). Within each ceramic group, significant differences were shown between the control group and the fatigued group (P ≤ .05 in both MZr and MLD groups).
Both MZr and MLD crowns exhibited cohesive fracture mode within the monolithic crown structure only. Both materials showed different fracture pattern with different numbers of fracture fragments (Fig. 6). None of the zirconia abutments were fractured upon completion of SLF testing of both groups.
SEM imaging showed the presence of hackles in both MZr and MLD fractured surfaces, which indicates the orientation of crack as shown in Fig. 7.
This study focused on implant-supported restorations using the hybrid abutment concept.16171829 Results of this study showed that implant-supported monolithic crowns made of zirconia had significantly higher fracture resistance compared to the monolithic crowns made of lithium disilicate material. The results also suggested that fatigue application caused significant reduction in the fracture resistance of both of the all-ceramic groups. These findings rejected the null hypothesis that there would be no significant difference in fracture load and fatigue resistance between monolithic zirconia and lithium disilicate implant-supported crowns used in combination with zirconia abutment cemented to Ti-Base.
To the authors' knowledge, this work is the first to report on this combination of materials, designs, and testing protocol in an in vitro context. Therefore, comparing the findings of the current study to the findings of published work should be made with caution.
Prior to releasing a material for clinical use, in vitro tests are necessary to prove materials' performance and applicability. Such tests can be performed in a short period of time with a standardized test parameters,30 and its results are more clinically relevant when the tests closely simulate the clinical conditions.31 Our results confirmed that chewing simulation over 5 years had an impact on the fracture strength of different all-ceramic crown systems when supported by implants. Mechanical stress and wet environment, “hydrothermal stress” in particular, can accelerate the aging of zirconia structures. Aging of zirconia, also termed as “low temperature degradation” (LTD),32 is a phenomenon where crystals slowly transform from the stable tetragonal phase to the less stable monoclinic phase in the absence of any mechanical load.3334 Various factors cause aging, such as grain size35, residual stress, as well as stabilizer type and content.33 Surface defects, processing and finishing techniques, as well as vapor and temperature also play a key role in aging of any zirconia structure.3436
Due to the material properties and geometry, monolithic crowns milled from zirconia perform well when used in the molar region and supported by implants.37 de Kok et al. reported that where only SLF was used, the highest load to fracture was observed for monolithic zirconia crowns, followed by lithium disilicate crowns, when cemented to a prefabricated titanium abutment.38 When monolithic crowns made of zirconia and lithium disilicate were compared, zirconia was superior to lithium disilicate in terms of fracture strength.3739 The present study showed that monolithic zirconia restorations had significantly higher fracture resistance compared to monolithic lithium disilicate crowns. Kelly reported that strong highly crystalline ceramics has more opaque appearance and less translucency compared to aesthetic ceramics.40 It is also known that ceramic materials strength declines when exposed to mechanical loading; this usually cause subcritical crack propagation initiated by humid environment of the artificial mouth imitating the oral environment.41
Using zirconia as an abutment material for implant-supported restorations has proven to be superior to other all-ceramic materials; an in vitro study42 on the fracture resistance of all-ceramic restorations on implants revealed that crowns supported with ZrO2 abutments withstood higher load to fracture than those supported with Al2O3 abutment. Clinically, a 4-year result of a prospective clinical study reported that abutments made of zirconia can provide enough stability to support single-tooth restorations in anterior and premolar regions when supported by implants show good response to both the soft and hard tissues.11 Similarly, a systematic review by Sailer et al.43 have reported a high cumulative success rate of zirconia implant abutments after 11-year follow up, in both the anterior and the posterior regions.
A recent study in 2016 on implant-supported monolithic crowns reported that lithium disilicate crowns had generally a higher fracture resistance value after thermocycling mechanical loading (TCML) compared to polished zirconia reinforced lithium silicate crowns.44 However, the difference in fracture force values was not statistically significant. Straumann implant-abutment dummies were used in this study rather than zirconia abutments.44
Two in vitro studies on hybrid-abutments were recently published.2123 Ceramic crown and lithium disilicate abutment on Ti sleeve were tested in the anterior region and were concluded to be clinically reliable; however, success was limited by the abutment screw.21 Similarly, zirconia abutments were tested against zirconia hybrid abutments,23 but load was directed on the abutments and no crowns were involved in the testing. Hence, results of both studies cannot be compared to the results of the current study.
With regard to the failure mode observed in this study, crowns made of both zirconia and lithium disilicate failed predominantly by bulk fracture involving the whole thickness of the crown. This mode of fracture is the most common mechanical failure in LD restorations reported in previous in vivo4546 and in vitro studies.4748 In clinical context, radial cracks from the cementation surface propagate toward the occlusal surface and cause bulk fracture of dental crowns.3149 However, bulk fracture in laboratory simulation is mostly the result of a Hertzian cone crack extension which extends from the surface underneath the loading indenter and propagates to the whole crown thickness.47 A previous in vitro study48 demonstrated that radial cracks at the cementation surface beneath the contact point did not occur until the cyclic loading force was increased to 1400 N. Therefore, the loading forces used during the cyclic loading in this study were not enough to generate radial cracks.
Although hybrid-abutment was suggested by Lin et al.16 and Hornbrook17 as an adequate approach in restoring teeth supported by implants, there is currently little scientific information and clinical data on the applicability of hybrid-abutment concept in specific and implant-supported restorations50 in general. Hence, the current study provides practitioners with evidence for choosing the designs and materials to protect the benefit of their patients and potentially provide manufacturers with feedback regarding processing and design issues.
While enamel would be considered the ideal material to be used as an antagonist for in vitro testing of restorative materials, the use of a spherical stainless steel indenter instead of natural tooth as an antagonist during the cyclic loading might be considered a limitation in this study. However, natural teeth vary in morphology and require a precise machining process for manufacturing which makes it less convenient and accurate.51 Also, using sphere antagonist in the in vitro cyclic loading testing was considered a good and adequate approach to understand the clinical flaw mechanism, although it is hard to predict.52
Within the limitation of the current study, it can be concluded that implant-supported monolithic zirconia restorations cemented to hybrid abutments are unlikely to fracture and should have satisfactory clinical performance withstanding molar masticatory forces. In addition, although the different aging process occurs in both zirconia and lithium disilicate crown materials, fatigue loading with simultaneous thermocycling caused aging in both of the tested materials and reduced their strength significantly. Finally, clinical trials are important to provide the final word in the applicability of the hybrid abutment concept, and current data is not sufficient to suggest a safe clinical serviceability. Therefore, further research is needed to define potential, limits, and long-term serviceability of such combination of materials and hybrid abutment system.
ACKNOWLEDGEMENTS
The authors would like to thank Ivoclar Vivadent for providing study materials. Also, grateful for Mrs. Helen Monaghan and Mrs. Kylie Mortimer from the School of Dentistry and Oral Health at Griffith University for facilitating prosthodontic laboratory resources during the preparation of study samples. Also, would like to thank Mr. Ian Underhill from School of Engineering at Griffith University for his help in the mechanical testing.
References
1. Kapos T, Evans C. CAD/CAM technology for implant abutments, crowns, and superstructures. Int J Oral Maxillofac Implants. 2014; 29:117–136. PMID: 24660194.


2. Santos MJ, Costa MD, Rubo JH, Pegoraro LF, Santos GC Jr. Current all-ceramic systems in dentistry: a review. Compend Contin Educ Dent. 2015; 36:31–37. quiz 38, 40. PMID: 25822404.
3. Pallis K, Griggs JA, Woody RD, Guillen GE, Miller AW. Fracture resistance of three all-ceramic restorative systems for posterior applications. J Prosthet Dent. 2004; 91:561–569. PMID: 15211299.


4. Batson ER, Cooper LF, Duqum I, Mendonça G. Clinical outcomes of three different crown systems with CAD/CAM technology. J Prosthet Dent. 2014; 112:770–777. PMID: 24980739.


5. Larsson C, Wennerberg A. The clinical success of zirconia-based crowns: a systematic review. Int J Prosthodont. 2014; 27:33–43. PMID: 24392475.


6. Fasbinder DJ, Dennison JB, Heys D, Neiva G. A clinical evaluation of chairside lithium disilicate CAD/CAM crowns: a two-year report. J Am Dent Assoc. 2010; 141:10S–14S. PMID: 20516109.
7. Avivi-Arber L, Zarb GA. Clinical effectiveness of implant-supported single-tooth replacement: the Toronto Study. Int J Oral Maxillofac Implants. 1996; 11:311–321. PMID: 8752552.
8. Scheller H, Urgell JP, Kultje C, Klineberg I, Goldberg PV, Stevenson-Moore P, Alonso JM, Schaller M, Corria RM, Engquist B, Toreskog S, Kastenbaum F, Smith CR. A 5-year multicenter study on implant-supported single crown restorations. Int J Oral Maxillofac Implants. 1998; 13:212–218. PMID: 9581407.
9. Prestipino V, Ingber A. Esthetic high-strength implant abutments. Part I. J Esthet Dent. 1993; 5:29–36. PMID: 8507510.
10. Prestipino V, Ingber A. Esthetic high-strength implant abutments. Part II. J Esthet Dent. 1993; 5:63–68. PMID: 8240848.


11. Glauser R, Sailer I, Wohlwend A, Studer S, Schibli M, Schärer P. Experimental zirconia abutments for implant-supported single-tooth restorations in esthetically demanding regions: 4-year results of a prospective clinical study. Int J Prosthodont. 2004; 17:285–290. PMID: 15237873.
12. Sailer I, Sailer T, Stawarczyk B, Jung RE, Hämmerle CH. In vitro study of the influence of the type of connection on the fracture load of zirconia abutments with internal and external implant-abutment connections. Int J Oral Maxillofac Implants. 2009; 24:850–858. PMID: 19865625.
13. Nascimento C, Pita MS, Fernandes FH, Pedrazzi V, de Albuquerque Junior RF, Ribeiro RF. Bacterial adhesion on the titanium and zirconia abutment surfaces. Clin Oral Implants Res. 2014; 25:337–343. PMID: 23316996.


14. Gomes AL, Montero J. Zirconia implant abutments: a review. Med Oral Patol Oral Cir Bucal. 2011; 16:e50–e55. PMID: 20526253.


15. Kohal RJ, Att W, Bächle M, Butz F. Ceramic abutments and ceramic oral implants. An update. Periodontol 2000. 2008; 47:224–243. PMID: 18412584.


16. Lin WS, Harris BT, Zandinejad A, Martin WC, Morton D. Use of prefabricated titanium abutments and customized anatomic lithium disilicate structures for cement-retained implant restorations in the esthetic zone. J Prosthet Dent. 2014; 111:181–185. PMID: 24360007.


17. Hornbrook D. Case report using the “H” abutment: achieving esthetics, strength, and predictability for the anterior implant. Compend Contin Educ Dent. 2015; 36:192194–198. 200–201. PMID: 25822745.
18. Traini T, Pettinicchio M, Murmura G, Varvara G, Di Lullo N, Sinjari B, Caputi S. Esthetic outcome of an immediately placed maxillary anterior single-tooth implant restored with a custom-made zirconia-ceramic abutment and crown: a staged treatment. Quintessence Int. 2011; 42:103–108. PMID: 21359243.
19. Selz CF, Vuck A, Guess PC. Full-mouth rehabilitation with monolithic CAD/CAM-fabricated hybrid and all-ceramic materials: A case report and 3-year follow up. Quintessence Int. 2016; 47:115–121. PMID: 26417616.
20. Rosentritt M, Hagemann A, Hahnel S, Behr M, Preis V. In vitro performance of zirconia and titanium implant/abutment systems for anterior application. J Dent. 2014; 42:1019–1026. PMID: 24699071.


21. Silva NR, Teixeira HS, Silveira LM, Bonfante EA, Coelho PG, Thompson VP. Reliability and Failure Modes of a Hybrid Ceramic Abutment Prototype. J Prosthodont. Epub 2016 Feb 24.


22. Honda J, Komine F, Kamio S, Taguchi K, Blatz MB, Matsumura H. Fracture resistance of implant-supported screw-retained zirconia-based molar restorations. Clin Oral Implants Res. 2017; 28:1119–1126. PMID: 27412687.


23. Kelly JR, Rungruanganunt P. Fatigue Behavior of Computer-Aided Design/Computer-Assisted Manufacture Ceramic Abutments as a Function of Design and Ceramics Processing. Int J Oral Maxillofac Implants. 2016; 31:601–609. PMID: 27183069.


24. Stimmelmayr M, Heiss P, Erdelt K, Schweiger J, Beuer F. Fracture resistance of different implant abutments supporting all-ceramic single crowns after aging. Int J Comput Dent. 2017; 20:53–64. PMID: 28294205.
25. Att W, Kurun S, Gerds T, Strub JR. Fracture resistance of single-tooth implant-supported all-ceramic restorations: an in vitro study. J Prosthet Dent. 2006; 95:111–116. PMID: 16473084.


26. Coelho PG, Silva NR, Bonfante EA, Guess PC, Rekow ED, Thompson VP. Fatigue testing of two porcelain-zirconia all-ceramic crown systems. Dent Mater. 2009; 25:1122–1127. PMID: 19395078.


27. Gibbs CH, Lundeen HC, Mahan PE, Fujimoto J. Chewing movements in relation to border movements at the first molar. J Prosthet Dent. 1981; 46:308–322. PMID: 6943340.


28. Strub JR, Gerds T. Fracture strength and failure mode of five different single-tooth implant-abutment combinations. Int J Prosthodont. 2003; 16:167–171. PMID: 12737249.
29. Ouzer A. The evolution and fabrication of implant-supported full-arch hybrid prostheses. From conventional casted metal to an all-ceramic zirconia. N Y State Dent J. 2015; 81:44–49.
30. Kern M, Strub JR, Lü XY. Wear of composite resin veneering materials in a dual-axis chewing simulator. J Oral Rehabil. 1999; 26:372–378. PMID: 10373083.


31. Kelly JR. Clinically relevant approach to failure testing of all-ceramic restorations. J Prosthet Dent. 1999; 81:652–661. PMID: 10347352.


32. Kobayashi K, Kuwajima H, Masaki T. Phase change and mechanical properties of ZrO2-Y2O3 solid electrolyte after ageing. Solid State Ionics. 1981; 3:489–495.
33. Beuer F, Schweiger J, Eichberger M, Kappert HF, Gernet W, Edelhoff D. High-strength CAD/CAM-fabricated veneering material sintered to zirconia copings-a new fabrication mode for all-ceramic restorations. Dent Mater. 2009; 25:121–128. PMID: 18620748.
34. Lughi V, Sergo V. Low temperature degradation -aging- of zirconia: A critical review of the relevant aspects in dentistry. Dent Mater. 2010; 26:807–820. PMID: 20537701.


35. Chai J, Chong KH. Probability of failure of machined zirconia dental ceramic core materials. Int J Prosthodont. 2009; 22:340–341. PMID: 19639068.
36. Denry I, Kelly JR. State of the art of zirconia for dental applications. Dent Mater. 2008; 24:299–307. PMID: 17659331.


37. Zhang Y, Mai Z, Barani A, Bush M, Lawn B. Fracture-resistant monolithic dental crowns. Dent Mater. 2016; 32:442–449. PMID: 26792623.


38. de Kok P, Kleverlaan CJ, de Jager N, Kuijs R, Feilzer AJ. Mechanical performance of implant-supported posterior crowns. J Prosthet Dent. 2015; 114:59–66. PMID: 25819357.


39. Zesewitz TF, Knauber AW, Nothdurft FP. Fracture resistance of a selection of full-contour all-ceramic crowns: an in vitro study. Int J Prosthodont. 2014; 27:264–266. PMID: 24905268.


40. Kelly JR. Dental ceramics: current thinking and trends. Dent Clin North Am. 2004; 48:513–530.
41. Ritter JE. Predicting lifetimes of materials and material structures. Dent Mater. 1995; 11:142–146. PMID: 8621036.


42. Yildirim M, Fischer H, Marx R, Edelhoff D. In vivo fracture resistance of implant-supported all-ceramic restorations. J Prosthet Dent. 2003; 90:325–331. PMID: 14564286.


43. Sailer I, Philipp A, Zembic A, Pjetursson BE, Hämmerle CH, Zwahlen M. A systematic review of the performance of ceramic and metal implant abutments supporting fixed implant reconstructions. Clin Oral Implants Res. 2009; 20:4–31. PMID: 19663946.


44. Rosentritt M, Hahnel S, Engelhardt F, Behr M, Preis V. In vitro performance and fracture resistance of CAD/CAM-fabricated implant supported molar crowns. Clin Oral Investig. 2017; 21:1213–1219.


45. Taskonak B, Sertgöz A. Two-year clinical evaluation of lithia-disilicate-based all-ceramic crowns and fixed partial dentures. Dent Mater. 2006; 22:1008–1013. PMID: 16375961.


46. Gehrt M, Wolfart S, Rafai N, Reich S, Edelhoff D. Clinical results of lithium-disilicate crowns after up to 9 years of service. Clin Oral Investig. 2013; 17:275–284.


47. Zhao K, Wei YR, Pan Y, Zhang XP, Swain MV, Guess PC. Influence of veneer and cyclic loading on failure behavior of lithium disilicate glass-ceramic molar crowns. Dent Mater. 2014; 30:164–171. PMID: 24331550.


48. Silva NR, Bonfante EA, Martins LM, Valverde GB, Thompson VP, Ferencz JL, Coelho PG. Reliability of reduced-thickness and thinly veneered lithium disilicate crowns. J Dent Res. 2012; 91:305–310. PMID: 22205635.


49. Kelly JR, Rungruanganunt P, Hunter B, Vailati F. Development of a clinically validated bulk failure test for ceramic crowns. J Prosthet Dent. 2010; 104:228–238. PMID: 20875527.


50. Patzelt SB, Spies BC, Kohal RJ. CAD/CAM-fabricated implant-supported restorations: a systematic review. Clin Oral Implants Res. 2015; 26:77–85.


51. Heintze SD, Zellweger G, Cavalleri A, Ferracane J. Influence of the antagonist material on the wear of different composites using two different wear simulation methods. Dent Mater. 2006; 22:166–175. PMID: 16087228.


52. Zhang Y, Sailer I, Lawn BR. Fatigue of dental ceramics. J Dent. 2013; 41:1135–1147. PMID: 24135295.


Fig. 2
Fabrication of sample holder (A) Implant positioning and the CS sample cup duplication to create a negative replica of the sample cup, (B & C) Creating the positive replica of the sample cup, (D) The positive sample cup replica with implant and Ti-Base abutment inverted, (E) Acrylic resin poured in the mold and checked in the original chewing simulator sample cup for fitting.
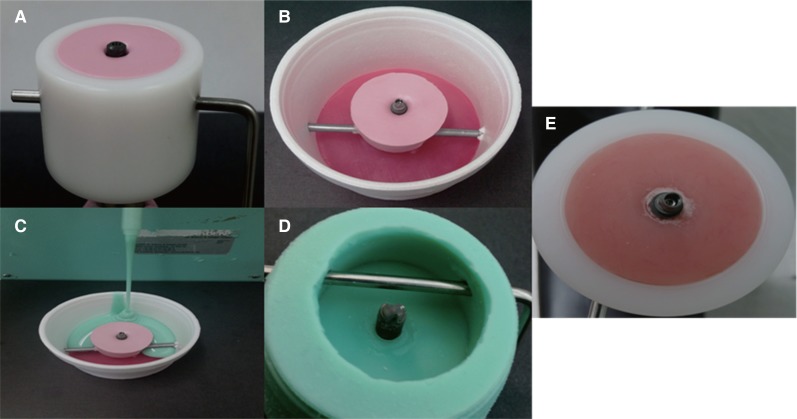
Fig. 4
Wear facets visible on the disto-buccal cusp of tested crowns after CS (arrows) using an endodontic microscope at 12×; (A) MZr, (B) MLD.
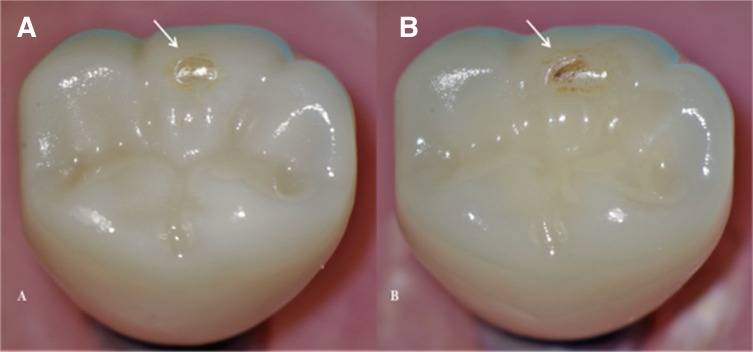
Fig. 6
Fracture pattern for the two tested groups after SLF (A) MZr; fracture along the mesiodistal plane and the lingual developmental groove, (B) MLD; fracture along the mesiodistal plane.
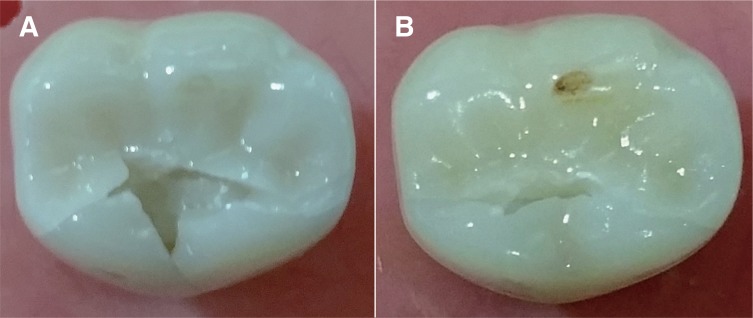
Fig. 7
Representative SEM images after fracture resistance testing showing hackles in both (A) MZr and (B) MLD.
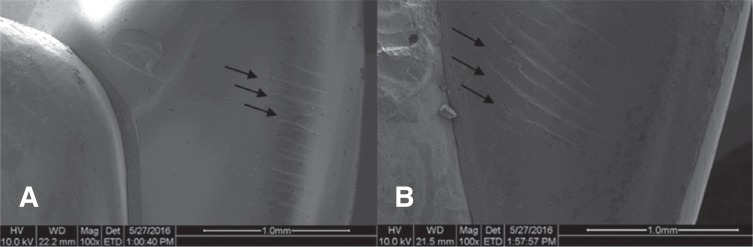
Table 1
Sintering conditions for zirconia structures in Programat S1 furnace
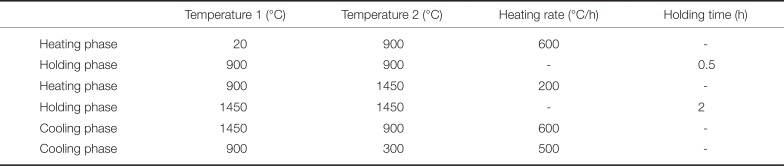