Abstract
PURPOSE
The purpose of this study was to analyze the effects of the splinted implant prosthesis in a reconstructed mandible using three-dimensional finite element analysis.
MATERIALS AND METHODS
Three-dimensional finite element models were generated from a patient's computed tomography data. The patient had undergone partial resection of the mandible that covered the area from the left canine to the right condyle. The mandible was reconstructed using a fibula bone graft and dental implants. The left mandibular premolars and molars remained intact. Three types of models were created. The implant-supported prosthesis was splinted and segmented into two or three pieces. Each of these models was further subcategorized into two situations to compare the stress distribution around normal teeth and implants. Oblique loading of 300 N was applied on both sides of the mandible unilaterally. The maximum von Mises stress and displacement of the models were analyzed.
RESULTS
The stress distribution of the natural mandible was more uniform than that of the reconstructed fibula. When the loading was applied to the implant prosthesis of reconstructed fibula, stress was concentrated at the cortical bone around the neck of the implants. The three-piece prosthesis model showed less uniform stress distribution compared to the others. Displacement of the components was positively correlated with the distance from areas of muscle attachment. The three-piece prosthesis model showed the greatest displacement.
Mandibular resection for the treatment of oral cancer, osteomyelitis, bisphosphonate related osteonecrosis of jaw bone, and infection may result in facial deformities and functional disorders of masticatory system. Therefore, the aim of reconstruction after the mandibular resection must include not only restoration of the contour of the mandible, but also restoration of overall masticatory functions.123 Currently, vascularized autogenous bone grafting has been used as one of the most frequent methods for mandibular reconstruction. This method allows a fibula free flap to be used to place dental implants in the reconstructed mandibular area.456
The success of the implant-supported fixed prosthesis in the reconstructed mandible depends not only on the quality and amount of bone being grafted, but also on the biomechanical aspect of the stress distribution from the occlusal load. Concentration of the stress on a particular site hinders successful reconstruction and may result in bone resorption.78 Excessive stresses may occur because of improper positioning and insufficient number of implants. In addition, segmentation of the prosthesis for a long span implant-supported fixed prosthesis may cause stress around the implant fixture, which can result in an inflammatory reaction or bone resorption.91011 Therefore, it is important to consider the design of the implant prosthesis for implant rehabilitation.
Three-dimensional finite element analysis helps provide information that is difficult to obtain directly in the oral cavity, and thus expands its application in dental researches.1213 Several studies have been conducted on the analysis of the relationship among various super-structured implants on the normal edentulous and partially edentulous mandibles. Meijer et al.14 set up a three-dimensional model of a human mandible with two dental implants in the interforaminal region. Stress distribution patterns were compared for implants connected by a bar structure with unconnected individual implants. As a result, only a little difference in the stress concentration was observed between the models with and without a bar superstructure. Korioth and Johann15 reported the influence of prosthetic superstructure shape, diverse loading conditions on the implant, extent range, and prosthetic material properties. Analysis of reconstructed mandible models were further developed by Tie et al.16 as they created reconstructed mandible models via autogenous bone grafts with different defect regions. The stress distribution on the cortical bone was evaluated by applying vertical occlusal force. These models were created as edentulous mandibles without a prosthetic superstructure. Nagasao et al.17 organized a study, in which they applied occlusal loading on implants and a bar superstructure in various types of mandibular reconstruction models. The stress around the fixtures significantly differed among the models. While minimization of the stress around the implant fixtures in the mandible is a major research topic in dentistry, few studies have focused on stress around implant-supported fixed prostheses in reconstructed mandibles. The stress distribution patterns around the fixed prosthesis of multiple implants vary significantly by their designs, particularly depending on whether they are splinted or nonsplinted. Kregzde18 suggested a prosthesis design that minimizes the force applied on implants and consequently reduces the stress in the supporting bone by investigating how implant positions and different schemes of prosthesis splinting affect induced stress in the bone. Bal et al.19 stated that the splinted prosthesis offered specific advantages according to the three-dimensional finite element analysis on dental implants of the premaxilla. On the other hand, separating the long-span superstructure into small pieces has some advantages, such as the improvement of marginal discrepancy after sectioning a long span prosthesis.20 A separated prosthesis is expected to reduce mandibular deflection in biomechanical aspects.3437 In addition, the use of segmented prostheses minimizes the cost of repair and remake when a local failure occurs in an implant-supported prosthesis.21
There have been many biomechanical studies using the finite element analysis of the dental implant on a natural mandible. However, it is not easy to find the biomechanical study on the effect of the splinting of the implant prosthesis in reconstructed fibula. The purpose of this study was to analyze the effects of splinted implant prosthesis in a reconstructed mandible using three-dimensional finite element analysis.
Based on the computed tomography (CT) data of a 24-year-old female patient, the finite element models were developed. The mandible of the patient was resected from the left mandibular canine to the right mandibular condyle. The mandible was reconstructed using a fibula bone graft and dental implants were placed to restore dentition of the patient. The implants were placed in areas of both canines, premolars, and molars. The external connection type implants were used, with the diameter of 3.3 mm on canines, 3.75 mm on the first premolar, and 4.0 mm on the second premolar and molars. All of the implants were 15.0 mm long. The left mandibular premolars and molars remained intact on the natural mandible. After reconstruction surgery, three-dimensional finite element models were generated from a patient's CT data. The CT data was converted to stereolithography (STL) files composed of triangular surface elements. The geometry of the components was then obtained using a segmentation program (Amira, FEI, Hillsboro, OR, USA).
The finite element models were composed of nine parts. The nine parts were the cortical bone, cancellous bone, implant fixtures, gold screws, abutments, anterior and posterior implant prostheses, natural teeth, and the periodontal ligament (PDL) (Fig. 1). Tetrahedral elements for the finite element analysis were constructed via meshing program (Visual-Mesh, ESI group, Paris, France), in which the final model had 93,494 nodes and 442,430 elements. The procedures of the study were reviewed and approved by the Institutional Review Board at Seoul National University Dental Hospital.
In order to simplify complicated calculations, all of the simulation models, including the supporting bones and prosthetic fixtures, were assumed to be linearly elastic and isotropic.12 Mechanical properties of the materials were based on previous studies,121724252627282930313233343536373839404142 as indicated in Table 1. The implant prosthesis was considered as a zirconia fixed partial denture.
The attachment sites for the masticatory muscles such as the masseter, the medial pterygoid, the lateral pterygoid, the temporal, and the suprahyoid muscles were determined from CT images obtained from the patient for the normal mandible. The same procedure was used for the reconstructed area. Nodes in the attachment areas of muscles were fixed in all directions.17 The contacts among the implant fixtures and the abutments, screws, covering prosthesis, and the surrounding bone were assumed to be perfectly bounded.14
Oblique loading was applied on the occlusal surface of the implant-supported prosthesis and those of natural teeth. Based on previous studies,1617272829 oblique loading was applied unilaterally on the occlusal surface of the second premolars and the first and second molars of the right mandible. The same procedure was carried out on the left mandible. The magnitude of the oblique loading was 300 N, and the ratio of the horizontal to vertical component was 1:5. The oblique loads were applied at 11.54° to the long axis of the implant, perpendicular to the central grooves of a dental arch from buccal to lingual direction.
Three finite element models were generated to evaluate the biomechanical behavior of the reconstructed mandible, which had implant-supported prostheses on fibula and natural teeth in intact mandible. The models were categorized based on the splinting of the prosthesis. The first model was composed of a one-piece prosthesis from the left mandibular canine to the right mandibular second molar. The oblique load of 300 N was applied on the implant-supported crowns (FB1) and then applied on the left natural teeth (MN1).
The second model was composed of the two-piece segmented prostheses, from the left canine to the right canine and from the right first premolar to the right second molar. The oblique load was applied on the prosthesis of the reconstructed fibula (FB2), and the same load was applied to the crowns of the intact mandible (MN2).
The third model had the three-piece prostheses, in which it was segmented into three pieces from the left canine to the right canine, the right first premolar to the second premolar, and the right first molar to the second molar. The oblique load was applied to the fibula side (FB3) and then the mandible side (MN3). The detailed description of those models is summarized in Table 2.
The three-dimensional finite element analysis program, Ansys v14.5 (Swanson Analysis system Inc., Houston, PA, USA) was used to analyze the biomechanical behavior of the models. In order to evaluate the biomechanical behavior, the maximum von Mises stress and displacement of the components were calculated.
When the loading was applied on the natural teeth, the high stress concentrations were observed in the posterior region of the molar, anterior mandibular ramus, region of the mandibular angle, coronoid process, and lower part of the condyle. However, when the loading was applied on the implants, the high stress concentrations were located in the bone around the implants. The von Mises stress distribution of each model is shown in Fig. 2. With same amount of load, all of the three models showed the higher maximum von Mises stress values in the reconstructed fibula.
Figure 3 shows the stress distributions in both cortical and cancellous bones around the implants and teeth. When the loading was applied on the area of reconstructed fibula, the high stress concentrations were observed. The highest von Mises stresses in the cortical bones ranged from 4.9 to 45.3 MPa in FB1, 6.4 to 52.4 MPa in FB2, and 21.7 to 56.1 MPa in FB3. Stress was concentrated at the neck of the implant fixtures, with greater degree of stress around the distal implant and the implant in the reconstructed site. On the other hand, the stress distribution of the normal mandible is uniformly distributed along the cortical bone. The highest von Mises stresses in the cortical bones ranged from 3.3 to 7.1 MPa in MN1, 2.4 to 7.4 MPa in MN2, and 2.6 to 7.7 MPa in MN3. These are summarized in Fig. 4.
Figure 5 shows the stress distributions in the implants and teeth. When the loading was applied on the area of implants, the stress tended to be highly concentrated. The highest von Mises stresses in the implants ranged from 38.1 to 67.7 MPa in FB1, 39.1 to 69.6 MPa in FB2, and 31.1 to 83.2 MPa in FB3. It was observed that stress was concentrated at the top of fixture and the inferior part of abutment in all models, whereas the stress distribution of the teeth is uniformly distributed. The highest von Mises stresses in the teeth ranged from 8.7 to 11.3 MPa in MN1, 8.7 to 11.6 MPa in MN2, and 8.8 to 11.6 MPa in MN3. These are expressed in Fig. 6.
Stress level varied with different superstructure designs. In FB1 and FB2, the highest maximum von Mises stresses were observed around the implant at the right second molar location as 45.3 MPa and 52.4 MPa, respectively. In FB3, the high stress concentrations were found in the bone around the right second premolar and molar implants, ranging from 21.7 to 53.9 MPa.
The value of maximum von Mises stress in the cortical bone was relatively small when the oblique load was applied to the natural teeth. In the one-piece prosthesis model, the highest maximum von Mises stress value in the cortical bone on MN1 was 15.7% of that on FB1. In the two-piece prosthesis model, the value on MN2 was 14.1% of that on FB2, and in the three-piece prosthesis model, the value on MN3 was 13.7% of that on FB3. While the maximum von Mises stress in the cortical bone of FB1, FB2, and FB3 showed greatly different values, the maximum von Mises stress values of MN1, MN2, and MN3 were similar. In addition, the stress distribution patterns of MN1, MN2, and MN3 were also similar. Stress distribution was more uniform on the normal mandible with natural teeth than on the reconstructed fibula with implants. FB1 and FB2 showed a similar pattern in stress distribution, whereas FB3 demonstrated a different pattern. Unlike FB1 and FB2, the stress was concentrated not only in the bone around the second molar region, but also in the bone around the first molar and second premolar regions in FB3. The maximum von Mises stress on the mandibular second molar region on FB3 resulted in 23.8% and 7.1% greater than that of FB1 and FB2, respectively. The mandibular first molar region on FB3 showed 738.6% and 582.3% greater than those of FB1 and FB2, and the mandibular second premolar region showed 342.9% and 312.2% greater than those of FB1 and FB2.
Displacements of the components were greater on sites farther away from muscle attachment areas (Fig. 7). When the loading was applied on the natural teeth side, the displacements of the components did not show significant difference. The displacement values for MN1, MN2, and MN3 were measured to be 83.4 µm, 85.1 µm, and 87.4 µm, respectively. However, in the reconstructed side, FB3 showed the greatest displacement compared to the others, as the values of FB1, FB2 and FB3 were 107.2 µm, 111.2 µm, and 130.6 µm, respectively. In FB1 and FB2, the right canine and first premolar showed the greatest displacements, whereas the right canine, premolars, and the mesial area of the first molar showed the greatest displacements in FB3.
This study used the mandibular reconstruction model with a fibula and implant prosthesis. The fibula, unlike other bone grafts, features a long dimension with a comparatively thick cortical bone layer, making it suitable for mandibular reconstruction.14 However, its achievable bone height and diameter are shorter than those of normal mandible.30 If stress is not properly distributed, subsequent large strain may interrupt the bone remodeling process to facilitate bone resorption or fracture.710 In this study, an equal level of oblique loading was applied to both natural teeth on the mandible and implants on the reconstructed fibula. The patterns of von Mises stress distribution of the natural mandibular side were similar to those of previous studies.143132 A tendency towards uniform stress distribution was seen throughout the mandible, with a maximum value ranging from 7.1 to 7.7 MPa. When the loading was applied on the reconstructed side, the highest stress value was shown at the neck of the implant. The posterior region of the fibula showed a stress concentration. The highest stress ranged from 45.3 to 56.1 MPa, which was six to eight times higher than that of natural teeth. The presence of a periodontal ligament (PDL) around the natural teeth may help efficient stress distribution.33 In addition, the thick cortical bone of the mandible might contribute to the favorable stress distribution. Implants are directly engaged in the bone without PDL and the width of the fibula is relatively thinner than that of the mandible, inducing the stress distribution less favorable.15
An adequate amount of mechanical stress below the stress bearing threshold will strengthen the bone.34 On the contrary, overload may occur when the stress is concentrated on the bone surrounding an implant. In addition, excessive stress beyond the threshold will cause fatigue and microdamage that may result in bone resorption.35 Although it has been suggested that there is no constant linear relationship between stress and bone failure, the stress level required for sudden fracture of bone was reported to be 120 MPa.36 According to the results of this study, the maximum stress that occurred in the region of the reconstructed mandible was 69.7 MPa, which was considerably lower than the stress level required for sudden fracture that Isidor36 proposed. Thus, it is fair to say that a fibula reconstruction with implants is relatively safe from the sudden bone fracture. However, the individual differences in the occlusal load should not be disregarded.
In this study, the difference in the stress distribution of various prosthetic designs was measured. There have been several studies on the effects of prosthetic splints on the bone concerning stress distribution. In 1999, Yang and Thompson32 insisted that splints on natural teeth abutment effectively dispersed the stress applied on the bone and eventually reduced stress, as determined through finite element analysis. Guichet et al.37 compared the load transfer of splinted and non-splinted external connection type implants using photoelastic analysis and reported that a splinted prosthesis showed a better load distribution than a nonsplinted prosthesis. Clelland et al.38 compared the stress distribution of splinted and nonsplinted prostheses with internal conical connection type implants using a 3-D image correlation technique. They reported that the splinted prosthesis generated more uniform stress distribution. Bal et al.19 asserted from their evaluation of the effect of splinting on implant stress distributionthat when the implants were splinted, stresses were reduced in the supporting bone under both horizontal and vertical loading conditions. Other studies have reported insignificant improvement in implant success rates of splinting implants in fixed partial prostheses, complete arch, or overdentures when compared to segmented implants.213940 Alvarez-Arenal et al.22 used three-dimensional finite element analysis to assess the stress distribution associated with splinted and nonsplinted implant-supported fixed complete denture designs in mandible. A framework segmented into three-pieces transferred the least stress to the surrounding bone. However, assessments require further analysis as masticatory muscle activities were excluded and the models were constructed on the basis of an edentulous mandible. In the present study, the results were in accordance with previous studies, which reported that splinted fixed prostheses transmitted less stress to the implant supporting bone than nonsplinted prostheses.193237 Because of the force distribution over wider area, splinted prostheses showed better load sharing than nonsplinted prostheses. From the left mandibular canine to the right second molar, stress was more evenly distributed when the prosthesis was fabricated in one or two pieces than in three pieces. In the same manner, the displacement value was higher in the three-piece prosthesis than in the one-piece or two-piece prosthesis. Because the reconstructed fibula showed a comparably unfavorable stress distribution, the presence of splinting in the superstructure design of the multi-unit implant prosthesis is recommended. From this point of view, the fabrication of the prosthesis in one or two-pieces is recommended in order to achieve improved stress distribution on the reconstructed mandible.
Models used in this study were on the basis of several assumptions. The anisotropic material properties of cortical and cancellous bone were assumed to be linearly elastic and isotropic. All structures were assumed to be perfectly bonded, which would be highly unlikely to occur in clinical situations. Frictional contact and microroughness could have a great influence on the force transfer, but not considered in this study. Preloading force on the abutment screw and surface contact between the fixture and abutment needs to be considered in the study design for further analysis. The mandible presents complex biomechanical behavior under the functional loading due to the complex structure of the bone and tissue.41 The biomechanical mandibular flexure could build up stress in the fixed prosthesis.42 It is necessary to make refinements concerning these problems in future studies.
Within the limitations of this study, multiple implant-supported fixed prostheses of the reconstructed fibula represent biomechanically successful treatment options. Splinted types of prosthesis design showed more advantages in terms of stress distribution in compromised situations such as mandibular reconstruction.
References
1. Flemming AF, Brough MD, Evans ND, Grant HR, Harris M, James DR, Lawlor M, Laws IM. Mandibular reconstruction using vascularised fibula. Br J Plast Surg. 1990; 43:403–409. PMID: 2393766.


2. Komisar A. The functional result of mandibular reconstruction. Laryngoscope. 1990; 100:364–374. PMID: 2181219.


3. August M, Tompach P, Chang Y, Kaban L. Factors influencing the long-term outcome of mandibular reconstruction. J Oral Maxillofac Surg. 2000; 58:731–737. PMID: 10883687.


4. Chang YM, Santamaria E, Wei FC, Chen HC, Chan CP, Shen YF, Hou SP. Primary insertion of osseointegrated dental implants into fibula osteoseptocutaneous free flap for mandible reconstruction. Plast Reconstr Surg. 1998; 102:680–688. PMID: 9727431.


5. Hidalgo DA. Fibula free flap: a new method of mandible reconstruction. Plast Reconstr Surg. 1989; 84:71–79. PMID: 2734406.
6. Zlotolow IM, Huryn JM, Piro JD, Lenchewski E, Hidalgo DA. Osseointegrated implants and functional prosthetic rehabilitation in microvascular fibula free flap reconstructed mandibles. Am J Surg. 1992; 164:677–681. PMID: 1463123.


7. Kuiper JH, Huiskes R. The predictive value of stress shielding for quantification of adaptive bone resorption around hip replacements. J Biomech Eng. 1997; 119:228–231. PMID: 9285333.


8. Sugiura T, Horiuchi K, Sugimura M, Tsutsumi S. Evaluation of threshold stress for bone resorption around screws based on in vivo strain measurement of miniplate. J Musculoskelet Neuronal Interact. 2000; 1:165–170. PMID: 15758514.
9. Hoshaw SJ, Brunski JB, Cochran GVB. Mechanical loading of Brånemark implants affects interfacial bone modeling and remodeling. Int J Oral Maxillofac Implants. 1994; 9:345–360.
10. Brunski JB. In vivo bone response to biomechanical loading at the bone/dental-implant interface. Adv Dent Res. 1999; 13:99–119. PMID: 11276755.


11. Kopp CD. Overdentures and osseointegration. Case studies in treatment planning. Dent Clin North Am. 1990; 34:729–739. PMID: 2227043.
12. Geng JP, Tan KB, Liu GR. Application of finite element analysis in implant dentistry: a review of the literature. J Prosthet Dent. 2001; 85:585–598. PMID: 11404759.


13. Teixeira ER, Sato Y, Akagawa Y, Shindoi N. A comparative evaluation of mandibular finite element models with different lengths and elements for implant biomechanics. J Oral Rehabil. 1998; 25:299–303. PMID: 9610858.


14. Meijer HJ, Starmans FJ, Steen WH, Bosman F. A three-dimensional, finite-element analysis of bone around dental implants in an edentulous human mandible. Arch Oral Biol. 1993; 38:491–496. PMID: 8343071.


15. Korioth TW, Johann AR. Influence of mandibular superstructure shape on implant stresses during simulated posterior biting. J Prosthet Dent. 1999; 82:67–72. PMID: 10384165.


16. Tie Y, Wang DM, Ji T, Wang CT, Zhang CP. Three-dimensional finite-element analysis investigating the biomechanical effects of human mandibular reconstruction with autogenous bone grafts. J Craniomaxillofac Surg. 2006; 34:290–298. PMID: 16781160.


17. Nagasao T, Kobayashi M, Tsuchiya Y, Kaneko T, Nakajima T. Finite element analysis of the stresses around endosseous implants in various reconstructed mandibular models. J Craniomaxillofac Surg. 2002; 30:170–177. PMID: 12220996.


18. Kregzde M. A method of selecting the best implant prosthesis design option using three-dimensional finite element analysis. Int J Oral Maxillofac Implants. 1993; 8:662–673. PMID: 8181829.
19. Bal BT, Cağlar A, Aydin C, Yilmaz H, Bankoğlu M, Eser A. Finite element analysis of stress distribution with splinted and nonsplinted maxillary anterior fixed prostheses supported by zirconia or titanium implants. Int J Oral Maxillofac Implants. 2013; 28:e27–e38. PMID: 23377079.


20. Schiffleger BE, Ziebert GJ, Dhuru VB, Brantley WA, Sigaroudi K. Comparison of accuracy of multiunit one-piece castings. J Prosthet Dent. 1985; 54:770–776. PMID: 3908654.


21. Zarone F, Apicella A, Nicolais L, Aversa R, Sorrentino R. Mandibular flexure and stress build-up in mandibular fullarch fixed prostheses supported by osseointegrated implants. Clin Oral Implants Res. 2003; 14:103–114. PMID: 12562372.


22. Alvarez-Arenal A, Brizuela-Velasco A, DeLlanos-Lanchares H, Gonzalez-Gonzalez I. Should oral implants be splinted in a mandibular implant-supported fixed complete denture? A 3-dimensional-model finite element analysis. J Prosthet Dent. 2014; 112:508–514. PMID: 24560983.


23. Maezawa N, Shiota M, Kasugai S, Wakabayashi N. Three-dimensional stress analysis of tooth/lmplant-retained long-span fixed dentures. Int J Oral Maxillofac Implants. 2007; 22:710–718. PMID: 17974104.
24. Dittmer MP, Kohorst P, Borchers L, Stiesch-Scholz M. Finite element analysis of a four-unit all-ceramic fixed partial denture. Acta Biomater. 2009; 5:1349–1355. PMID: 19117821.


25. Rees JS, Jacobsen PH. Elastic modulus of the periodontal ligament. Biomaterials. 1997; 18:995–999. PMID: 9212195.


26. Sakaguchi RL, Borgersen SE. Nonlinear contact analysis of preload in dental implant screws. Int J Oral Maxillofac Implants. 1995; 10:295–302. PMID: 7615325.
27. Curtis DA, Plesh O, Miller AJ, Curtis TA, Sharma A, Schweitzer R, Hilsinger RL, Schour L, Singer M. A comparison of masticatory function in patients with or without reconstruction of the mandible. Head Neck. 1997; 19:287–296. PMID: 9213107.


28. Curtis DA, Plesh O, Hannam AG, Sharma A, Curtis TA. Modeling of jaw biomechanics in the reconstructed mandibulectomy patient. J Prosthet Dent. 1999; 81:167–173. PMID: 9922429.


29. O'Mahony AM, Williams JL, Spencer P. Anisotropic elasticity of cortical and cancellous bone in the posterior mandible increases peri-implant stress and strain under oblique loading. Clin Oral Implants Res. 2001; 12:648–657. PMID: 11737110.
30. Bähr W, Stoll P, Wächter R. Use of the “double barrel” free vascularized fibula in mandibular reconstruction. J Oral Maxillofac Surg. 1998; 56:38–44. PMID: 9437980.


31. Sulik WD, White JT. Modification of stresses surrounding abutment teeth for fixed partial dentures induced by various levels of periodontal support: a photoelastic study. J Prosthet Dent. 1981; 46:32–35. PMID: 7024515.


32. Yang HS, Thompson VP. A two-dimensional stress analysis comparing fixed prosthodontic approaches to the tilted molar abutment. Int J Prosthodont. 1991; 4:416–424. PMID: 1811637.
33. Poiate IA, de Vasconcellos AB, de Santana RB, Poiate E. Three-dimensional stress distribution in the human periodontal ligament in masticatory, parafunctional, and trauma loads: finite element analysis. J Periodontol. 2009; 80:1859–1867. PMID: 19905956.


34. Frost HM. Perspectives: bone's mechanical usage windows. Bone Miner. 1992; 19:257–271. PMID: 1472896.


35. Hansson S. A conical implant-abutment interface at the level of the marginal bone improves the distribution of stresses in the supporting bone. An axisymmetric finite element analysis. Clin Oral Implants Res. 2003; 14:286–293. PMID: 12830797.
36. Isidor F. Influence of forces on peri-implant bone. Clin Oral Implants Res. 2006; (17 Suppl 2):8–18.


37. Guichet DL, Yoshinobu D, Caputo AA. Effect of splinting and interproximal contact tightness on load transfer by implant restorations. J Prosthet Dent. 2002; 87:528–535. PMID: 12070516.


38. Clelland NL, Seidt JD, Daroz LG, McGlumphy EA. Comparison of strains for splinted and nonsplinted implant prostheses using three-dimensional image correlation. Int J Oral Maxillofac Implants. 2010; 25:953–959. PMID: 20862409.
39. Stoumpis C, Kohal RJ. To splint or not to splint oral implants in the implant-supported overdenture therapy? A systematic literature review. J Oral Rehabil. 2011; 38:857–869. PMID: 21480943.


40. Barão VA, Delben JA, Lima J, Cabral T, Assunção WG. Comparison of different designs of implant-retained overdentures and fixed full-arch implant-supported prosthesis on stress distribution in edentulous mandible-a computed tomography-based three-dimensional finite element analysis. J Biomech. 2013; 46:1312–1320. PMID: 23518207.
41. Ashman RB, Van Buskirk WC. The elastic properties of a human mandible. Adv Dent Res. 1987; 1:64–67. PMID: 3326617.


42. Fischman BM. The influence of fixed splints on mandibular flexure. J Prosthet Dent. 1976; 35:643–647. PMID: 784954.


Fig. 1
Three-dimensional finite element model. (A) Reconstructed mandible, (B) Cortical bone, (C) Cancellous bone, (D) Posterior implant prosthesis, (E) Anterior implant prosthesis, (F) Implant fixture, gold screw, and abutment.
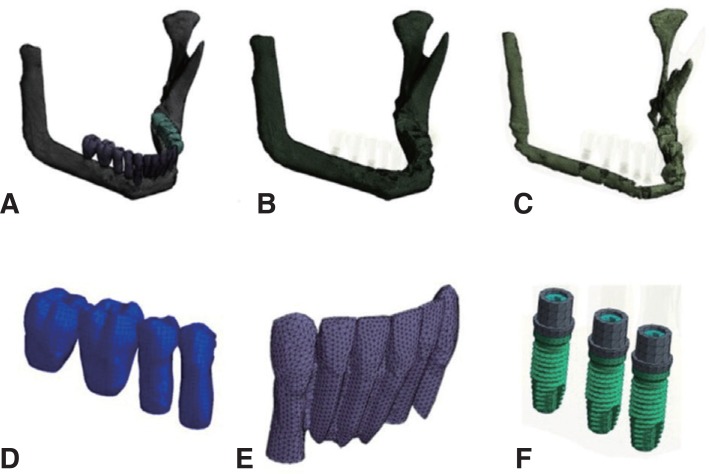
Fig. 3
Stress distributions in cortical and cancellous bones. (A) FB1, (B) FB2, (C) FB3, (D) MN1, (E) MN2, (F) MN3.

Fig. 5
Stress distributions in implants and teeth. (A) FB1, (B) FB2, (C) FB3, (D) MN1, (E) MN2, (F) MN3.
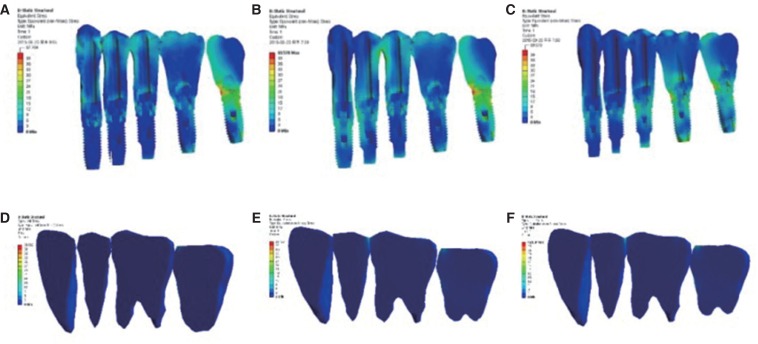
Fig. 7
The displacement tendency on each model. (A) FB1, (B) FB2, (C) FB3, (D) MN1, (E) MN2, (F) MN3.
