Abstract
STATEMENT OF PROBLEM
Zirconia-based restorations have the common technical complication of delamination, or porcelain chipping, from the zirconia core. Thus the shear bond strength between the zirconia core and the veneering porcelain requires investigation in order to facilitate the material's clinical use.
PURPOSE
The purpose of this study was to evaluate the bonding strength of the porcelain veneer to the zirconia core and to other various metal alloys (high noble metal alloy and base metal alloy).
MATERIAL AND METHODS
15 rectangular (4×4×9mm) specimens each of zirconia (Cercon), base metal alloy (Tillite), high noble metal alloy (Degudent H) were fabricated for the shear bond strength test. The veneering porcelain recommended by the manufacturer for each type of material was fired to the core in thickness of 3mm. After firing, the specimens were embedded in the PTFE mold, placed on a mounting jig, and subjected to shear force in a universal testing machine. Load was applied at a crosshead speed of 0.5mm/min until fracture. The average shear strength (MPa) was analyzed with the one-way ANOVA and the Tukey's test (α= .05). The fractured specimens were examined using SEM and EDX to determine the failure pattern.
RESULTS
The mean shear strength (± SD) in MPa was 25.43 (± 3.12) in the zirconia group, 35.87 (± 4.23) in the base metal group, 38.00 (± 5.23) in the high noble metal group. The ANOVA showed a significant difference among groups, and the Tukey's test presented a significant difference between the zirconia group and the metal group. Microscopic examination showed that the failure primarily occurred near the interface with the residual veneering porcelain remaining on the core.
For the past 40 years the porcelain-fused-to-metal systems have been extensively used in fixed partial dentures (FPDs) and still represents the gold standard.1 The advantages of the PFM systems are to combine the fracture resistance of the metal substructure with the esthetic property of the porcelain. However, recently the increasing demand for esthetic restorations as well as the questionable biocompatibility of some dental metal alloys has accelerated the development and improvement of metal-free restorations.2
In the early 1990s, yttrium oxide partially stabilized tetragonal zirconia polycrystal (Y-TZP) was introduced to dentistry as a core material for all-ceramic restoration and has been applied to clinical use through the CAD/CAM technique. Due to the transformation toughening mechanism, Y-TZP has been shown to have superior mechanical properties compared to other all-ceramic systems. (flexural strength of 900 - 1200 MPa, and fracture toughness of 9 - 10 MPa·m1/2)3 Due to its mechanical property, zirconia has enough strength to withstand the high occlusal stress.2,4 Therefore, it can be used in extensive all-ceramic FPDs having more than 4 units.5
According to clinical studies, the Y-TZP core ceramic exhibited high stability as a framework material. No fractures of the zirconia framework have been reported so far.6-8 However, delamination or minor chip-off fracture of veneering porcelain was described as the most frequent reason for the failures of zirconia FPDs. The incidence of veneer fractures in zirconia FPDs was significantly higher compared with those in metal-ceramic FPDs.6 Therefore, the bond between core and veneer or the veneer material itself is one of the weaknesses in layered zirconia based restorations and plays a significant role in their long-term success.9
The adhesion mechanism between metal and porcelain is believed to be the micro-mechanical bond, compatible coefficient of thermal expansion (CTE) match, van der Waals force, and mainly the suitable oxidation of metal and interdiffusion of ions between the metal and porcelain.10 Data presented in literature has shown the bond strength of ceramic or resin to metal substrates to be in the range of 54 - 71 MPa11, and a sufficient bond for metal-ceramic has been accepted when the fracture stress is greater than 25 MPa.12,13
However, the bonding mechanisms for veneering ceramic to the zirconia are up to now unclear. According to investigations on the wettability of the zirconia core with the veneering ceramic, micromechanical interactions were merely regarded. Moreover, there are less information available on the bond strength values between the all-ceramic core and veneering materials, and there exists no accurate test method for obtaining information on core/veneer adhesion in bi-layered all-ceramic materials in dentistry.
Many variables may affect the zirconia core-veneer bond strength; such as the surface finish of the core, which can affect mechanical retention; residual stress generated by mismatch in coefficient of thermal expansion (CTE); development of flaws and structure defects at core-veneer interface; and wetting properties and volumetric shrinkage of the veneer.14 The cause of core-veneer bond failure may be related to multiple factor.
The purpose of this study was to evaluate the shear bond strength of zirconia and metal alloys with their corresponding veneering porcelains. Scanning electron microscopy (SEM) was used to classify the failure pattern, and the interface chemistry was evaluated using energy dispersive X-ray microanalysis (EDX).
The materials tested for this study were listed in Table I.
Three types of core-veneer combinations (N = 45, n = 15/group) were fabricated by one dental technician according to the manufacturer's instructions. The corresponding porcelains for each core were veneered to zirconia (Group I), base metal alloy (Group II), and high noble metal alloy (Group III).
Fully sintered Cercon®blocks (Degudent, Hanau, Germany) (23 × 15 × 9 mm) were used for this study. The Cercon® Base blocks were sandblasted with 110 µm Al2O3 particles at 2.5 bar pressure according to the manufacturer's pre-treatment recommendation. The bars were steam-cleaned and air-dried. After a thin liner (Cercon® Ceram Kiss Liner, Degudent Hanau, Germany) layer was fired, the veneering ceramic (Cercon® ceram kiss, Degudent, Hanau, Germany) was built up to the final dimension (thickness of 3 mm) according to the firing program of the manufacturer (Austromat 3001, Dekema Dental-Keramiköfen GmbH & Co, Freilassing, Germany). Due to the shrinkage of porcelain, three separate firings were required to establish the correct dimension.
The blocks were cut in a sawing machine with diamond wheels to 15 bars (4 × 4 × 12 mm: 9 mm core / 3 mm veneer) (Fig. 1). After surface examinations with a magnifying glass, the intact specimens were selected.
The bars (4 × 4 × 9 mm) were cast in Ni-Cr base metal ceramic alloy (Tillite, Talladium Inc., LA,USA), high noble metal ceramic alloy (Degudent H, Degudent, Hanau, Germany), according to the manufacturer's instructions. The veneering ceramic (Vita VM13, VitaZahnfabrik, BadSäckingen, Germany) was built up to thickness of 3mm after degassing, second layer of opaque firing procedures. All specimens were examined and excess porcelain was removed using a high speed diamond bur with a low-speed handpiece. The final dimensions of bars of group II and III were identical to those of group I.
Each bar was embedded in the customized polytetrafluoroethylene (PTFE) mold using PMMA resin. Every effort was made to place the core-veneer interface on the same level as the upper plane of the mold (Fig. 2).
The core-veneer interface of the specimen was placed on the same level as the upper plane of the mold using discs for horizontal plane adjustment.
The PTFE molds holding the specimen were first inserted into a custom-made shear test jig (Instron, Canton, MA, USA), and the jig was secured in a bench vice. Then, the specimens were stressed in shear at a constant crosshead speed of 0.5 mm/min15 until failure occurred using an Instron Universal Testing machine (Model 3345, Instron, Canton, MA, USA). The test was carried out at room temperature. Force was applied to the specimen so that shear load was exerted adjacent to and directly to the bonding interface (Fig. 3).
Load deflection curves and ultimate load to failure were recorded automatically and displayed by the computer software of the testing machine (Bluehill® Lite software, Instron Canton MA, USA). Shear bond force was recorded in Newtons, and the average shear bond strength (MPa) was calculated through dividing the load (N) at which failure occurred by the bonding area (mm2).
Shear stress (MPa)= Load (N) ÷ Area (mm2)
To determine the mode of failure, the broken specimens were examined under scanning electron microscopy (SEM) (s-4700, Hitachi, Japan) under × 30 to × 1000 magnifications.
The definition for failure modes are presented in Table II. And the chemical composition at the fractured core was analyzed using energy dispersive X-ray microanalysis (EDX).
Statistical analysis was carried out using statistical software (SPSS 14.0, SPSS, Inc., Chicago, IL, USA). The data was analyzed using the one-way analysis of variance test (ANOVA) and the Tukey's multiple comparison test. The test was performed at a level of significance of 0.05.
Table III shows the mean shear strength of the core-veneer interface of 3 groups. The highest mean shear strength was recorded for group III (38.00 ± 5.23 MPa) followed by group II (35.87 ± 4.23 MPa) and group I (25.43 ± 3.12 MPa).
The one-way ANOVA showed a significant difference for the shear bond strength among the materials tested at the significant level of 0.05 (Table IV). The Tukey's multiple comparisons of the test were computed to make all pair-wise comparisons among the 3 groups in this study. These comparisons are listed in the last column of Table III. The P values of the different comparisons did not show significant difference between the metal groups (II, III). But the zirconia group (group I) had significantly lower values than Group II and Group III (P < .05).
Upon examination under the SEM (× 30), the zirconia group exhibited mixed cohesive/adhesive failures with only small remnants of porcelain attached to the core material. A SEM images of the zirconia group under high magnification showed many small pores in the veneering porcelain, where fracture originated and propagated in the veneering ceramics. Careful examination exhibited a thin layer of veneering porcelain covering the fracture surface(Fig. 4A, 4B, and 4C). Also, EDX results revealed that fractured zirconia surfaces were mainly covered by liner or veneer material and some zirconia crystals were exposed (Fig. 7A).
Some base metal alloy specimens revealed mixed cohesive/adhesive failures with porcelain attached to the loaded side of the core. But many specimens presented a cohesive failure between the metal oxide and alloy, with a thin metal oxide layer covering areas of the fractured surface. Under high magnification evaluation, there were many pores in veneering porcelain and internal pores acted as the fracture origin (Fig. 5A, 5B and 5C). Fractographic analysis by SEM showed that the fracture origin in the veneering porcelain was mostly on the loaded surface. EDX results revealed that the fractured core surface mainly failed in oxide layer or at oxide layer/metal interface (Fig. 7B).
In high noble metal alloy group (III), SEM image and EDX analysis of the fractured surface showed that cohesive failures within the veneering porcelain were predominant (Fig. 6).
The bond strength measurement of metal ceramic system was standardized by the Organization of Standardization through the Schwickerath crack initiation test (three point bending test), and the mean debonding strength/crack initiation strength should be greater than 25 MPa to meet the ISO requirement.13 Due to the brittleness of all-ceramic core materials this test setup cannot be applied to all-ceramic multilayered system.16 To date an adequate standardized test set-up and a minimum required bond strength for bi-layered all-ceramic materials has not been determined.17
In a survey of the literature, few articles utilized various bond strength test methods for all-ceramic core and veneering ceramic, such as the shear bond strength test17-20, three and four point loading test21, biaxial flexure strength test14, and the microtensile bond strength test.19,22,23,28 However, each test has a common limitation which is the difficulty in determining the core-veneer bond strength from applied force at failure on the sample in the specific test setup. In this study, the shear bond strength test method was selected because of its simplicity, such as the ease of specimen preparation, simple test protocol and the ability to rank different products according to bond strength values. But the SBS test has some disadvantages such as high standard deviations, occurrence of non-uniform interfacial stresses, and the influence from specimen geometry. Therefore, the standardization of specimen preparation, cross-sectional surface area, rate of loading application are important for improving the clinical usefulness of SBS test.
The specimens tested in this study were fabricated in rectangular forms so as to standardize the cross-sectional area easily. But there are some limitations in the methodology of this study. The first problem lies in the fixation of the test specimens by embedding in the customized PTFE mold using PMMA resin. When the strength of PMMA resin is weaker than the metal-veneer bond strength (especially the high noble metal-veneer group), failures occurred within the PMMA resin. Thus, improved method for fixation of specimens should be required. And the other limitation is that the specimens had to be custom fabricated and subjected to grinding, which may have produced some flaws or cutting defects in the specimens.
In previous studies, Dundar et al.18 reported shear bond strength in the range of 23 - 41 MPa and Al-Dohan17 reported shear bond strength in range of 22 - 31 MPa for commercially available core-veneer all-ceramic systems. In this study, the SBS value of veneering ceramic to zirconia core was 25.43 MPa, confirming the findings of previous studies. However unlike in Al-Dohan's study17, our study's results indicate a significant difference in mean SBS values between the zirconia group and metal groups. This difference in findings could be attributed to many factors, such as study design, methodology, skill and experience of the operator, and different properties of different materials.
Although there were no significant differences in mean SBS values between base metal alloy and high noble metal alloy to the corresponding veneer, the value of the high noble alloy group(38 MPa) was higher than that of the base metal group (35. 87 MPa).
The longevity of metal ceramic restorations depends on reliable bonding between metal and ceramic, primarily produced by the oxide layer.10 If the oxide layer is absent or thin, it would be completely eliminated during ceramic sintering, resulting in poor bonding. However, a heavy oxide layer should be avoided because it has poor cohesive strength24 it would obstruct the mechanical bond. According to the manufacturer, thicker oxide layers occur in nickel- and cobalt-based alloy because they contain elements that easily oxidate during the initial step of oxidation. Therefore, the initial oxidation step is not recommended. In this study, initial oxidation step was performed on the both base metal alloy and high noble metal alloy, which may cause the low values of SBS for base metal alloy group.
The predominance of cohesive failures of ceramic in the high noble metal group (Group III) suggests that the adhesive zone (opaque ceramic/oxide layer interface, oxide layer and oxide layer/alloy interface) had higher strength than that of the ceramic. In contrast, for the base metal alloys, a predominance of interface failures was noted, suggesting that the oxide layer was weaker than that of the veneering ceramic.
Understanding the failure mechanics of dental ceramic is one of the key points in developing stronger ceramic materials. Fractography has always been a powerful tool in understanding the failure mechanics of brittle materials such as dental ceramics. Identifying location, size, and types of crack initiation illustrates how cracks start, propagate, and extend to a macroscopic level, ending ultimately in fracture restoration.25
SEM evaluation revealed that the fracture originated in veneering porcelain in both the zirconia and metal ceramic groups. The failure modes of the specimens from the metal ceramic and zirconia groups suggest the importance of the mechanical properties of the veneering porcelain, since cracks initiated in the veneering porcelain. It is possible that pores and internal defects of veneer lead to the initiation of fracture. Thus, the fabrication technique has critical procedures, such as layering, firing, surface finishing, and polishing of veneering porcelain.26 In addition, the strength of the veneering porcelain, which is also related to the degree of crystallinity of the veneering porcelain, is paramount to the longevity of the restorations.27
Recently, a new generation of ceramic has been introduced for the veneering zirconia framework adopting the pressing technology. The advantages of this system are simplicity, quickness, and defect-free structures. Also the higher tensile strength of these press-on veneers, in addition to their superior interface quality and higher bond strength with zirconia, makes them optimal materials for application.28 Thus further studies comparing different veneering techniques, such as the conventional layering technique and pressed-on veneering technique, are needed.
The results of this study are in agreement with the observed clinical behavior of zirconia based system. In some clinical studies, the mechanical failures of zirconia-based systems were in the form of minor cohesive porcelain chipping. Improving the bonding strength of zirconia core-veneer and the strength of veneering porcelain may reduce the failure due to chipping or delamination of veneering porcelain.
As the limitation of this study was the fact that a static test was performed in a dry environment. Water would be constantly present in the actual oral environment, which would undergo repeated temperature and pH changes. According to most studies on the bond strength, the actual bond strength would be lower than expected since the bond strength would decrease further with thermocycling or artificial aging.29 Therefore thermocycling or artificial aging procedures should be included in subsequent studies.
Within the limitations of this study, the following conclusions can be drawn.
1. There was no significant difference among metal-ceramic groups (Group II vs Group III) in the shear bond strength. (P < .05)
2. There was a significant difference between the metal ceramic groups and zirconia group in the shear bond strength (P < .05).
3. Surface analysis of failure modes showed combined failure modes: cohesive failures in the veneer (loaded side) and adhesive failures at the core veneer interface (unloaded side). SEM evaluation showed that the fractures originated in the veneering porcelain in both the zirconia and metal groups and the fracture origin in the veneering porcelain was mostly on the loaded surface. In the case of interfacial fractures, a thin layer of the veneering ceramic or oxide layer remained on the core materials.
Figures and Tables
Fig. 4
SEM image of zirconia-veneer group (Group I).
(A) The arrow indicates the direction of load. The loaded side demonstrates cohesive failure within the veneering porcelain (original magnification × 30), (B) Note many pores within veneering 'porcelain (arrow), where fracture originated. The fractured Cercon Ceram kiss veneer demonstrates multiple cracks extending in a vertical direction (Hackle patterns) (original magnification × 250), (C) High magnification SEM image exhibited a very thin layer of porcelain covering zirconia grains (original magnification × 1000).
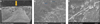
Fig. 5
SEM image of base metal alloy-veneer group (Group II).
(A) The arrow indicates the direction of load. The loaded side demonstrates cohesive failure within the veneering porcelain (original magnification × 30), (B) Interface of the veneering porcelain and the metal core (original magnification × 250), (C) High magnification SEM image exhibited an opaque layer and an oxide layer (original magnification × 1000).
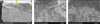
Fig. 6
SEM image of high noble metal alloy-veneer group (Group III)
(A) The arrow indicates the direction of load (original magnification × 30), (B) Predominance of cohesive failure (original magnification × 250), (C) High magnification SEM image exhibited an opaque layer and oxide layer (original magnification × 1000).
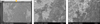
Fig. 7
EDX results of Group I, II, III.
(A) EDX results of Group I showed the presence of a thin porcelain layer over the zirconia, (B) EDX results of the fractured base metal alloy surface (Group II) demonstrated an exposed metal surface with some ceramic remainder, (C) EDX results of the fractured high noble metal alloy surface (Group III) presented no exposed metal surface.
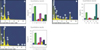
References
1. Tan K, Pjetursson BE, Lang NP, Chan ES. A systemic review of the survival and complication rates of fixed partial dentures (FPDs) after an observation period of at least 5 years. Clin Oral Implants Res. 2004. 15:654–666.
2. Raigrodski AJ. Contemporary materials and technologies for all-ceramic fixed partial dentures: a review of the literature. J Prosthet Dent. 2004. 92:557–562.
3. Guazzato M, Albakry M, Ringer SP, Swain MV. Strength, fracture toughness and microstructure of a selection of all-ceramic materials. Part II. Zirconia based dental ceramics. Dent Mater. 2004. 20:449–456.
4. Tinschert J, Natt G, Mohrbotter N, Spiekermann H, Schulze KA. Lifetime of alumina- and zircoina ceramics used for crown and bridge restorations. J Biomed Mater Res B Appl Biomater. 2007. 80:317–321.
5. Sundh A, Sjogren G. A comparison of fracture strength of yttrium-oxide-partially-stabilized zirconia ceramic crowns with varying core thickness, shapes and veneer ceramics. J Oral Rehabil. 2004. 31:682–688.
6. Sailer I, Pjetursson BE, Zwahlen M, Hammerle CHF. A Systematic review of the survival and complication rates of all-ceramic and metal-ceramic reconstructions after an observation period of at least 3 years. Part II: Fixed partial prostheses. Clin Oral Implants Res. 2007. 18:86–96.
7. Sailer I, Feher A, Filser F. Prospective clinical study of zirconia posterior fixed partial dentures: 3-year follow-up. Quintessence Int. 2006. 37:685–693.
8. Raigrodski AJ, Chiche GJ, Potiket N. The efficacy of posterior three-unit zirconium-oxide-based ceramic fixed partial denture prostheses: a prospective clinical pilot study. J Prosthet Dent. 2006. 96:237–244.
9. Guazzato M, Proos K, Sara G, Swain MV. Strength, reliability, and mode of fracture of bilayered porcelain/core ceramics. Int J Prosthodont. 2004. 17:142–149.
10. Anusavice KJ. Phillips' science of dental materials. 2003. 11th Ed. Philadelphia: W.B. Saunders;621–654.
11. Ozcan M, Niedermeier W. Clinical study on the reasons and location of the failures of metal-ceramic restorations and survival of repairs. Int J Prosthodont. 2002. 15:299–302.
12. Craig RG, Powers JM. Restorative dental materials. 2002. 11th ed. Mosby.
13. ISO 9693 Metal-ceramic bond characterization (Schwickerath crack initiation test). 1999. Geneva, Switzerland: International Organization for Standardization.
14. Isgro G, Pallav P, van der Zel JM, Feilzer AJ. The influence of the veneering porcelain and different surface treatments on the biaxial flexural strength of a heat-pressed ceramic. J Prosthet Dent. 2003. 90:465–473.
15. Hara AT, Pimenta LA, Rodrigues AL Jr. Influence of crosshead speed on resin-dentin shear bond strength. Dent Mater. 2001. 17:165–169.
16. Albakry M, Guazzato M, Swain MV. Fracture toughness and hardness evaluation of three pressable all-ceramic dental materials. J Dent. 2003. 31:181–188.
17. Al-Dohan HM, Yaman P, Dennison JB, Razzoog ME, Lang BR. Shear strength of core-veneer interface in bi-layered ceramics. J Prosthet Dent. 2004. 91:349–355.
18. Dündar M, Ozcan M, Cömlekoglu E, Güngör MA, Artunç C. Bond strengths of veneering ceramics to reinforced ceramic core materials. Int J Prosthodont. 2005. 18:71–72.
19. Dündar M, Ozcan M, Gökçe B, Cömlekoglu E, Leite F, Valandro LF. Comparison of two bond strength testing methodologies for bilayered all-ceramics. Dent Mater. 2007. 23:630–636.
20. Guess PC, Kulis A, Witkowski S, Wolkewitz M, Zhang Y, Strub JR. Shear bond strengths between different zirconia cores and veneering ceramics and their susceptibility to thermocycling. Dent Mater. 2008. 24:1556–1567.
21. White SN, Miklus VG, McLaren EA, Lang LA, Caputo AA. Flexural strength of a layered zirconia and porcelain dental all-ceramic system. J Prosthet Dent. 2005. 94:125–131.
22. Aboushelib MN, de Jager N, Kleverlaan CJ, Feilzer AJ. Microtensile bond strength of different components of core veneered all-ceramic restorations. Dent Mater. 2005. 21:984–991.
23. Aboushelib MN, Kleverlaan CJ, Feiler AJ. Microtensile bond strength of different components of core veneered all-ceramic restorations. Part II: zirconia veneering ceramics. Dent Mater. 2006. 22:857–863.
24. de Melo RM, Travassos AC, Neisser MP. Shear bond strengths of a ceramic system to alternative metal alloys. J Prosthet Dent. 2005. 93:64–69.
25. Mecholsky J. Fractography: Determining the sites of fracture initiation. Dent Mater. 1995. 11:113–116.
26. Kelly JR, Giordano R, Pober R, Cima MJ. Fracture surface analysis of dental ceramics: clinically failed restorations. Int J Prosthodont. 1990. 3:430–440.
27. Quinn JB, Sundar V, Lloyd IK. Influence of microstructure and chemistry on the fracture toughness of dental ceramics. Dent Mater. 2003. 19:603–611.
28. Aboushelib MN, Kleverlaan CJ, Feilzer AJ. Microtenisile bond strength of different componentsof core veneered all-ceramic restorations. Part 3: double veneer tenchnique. J Prosthodont. 2008. 17:9–13.
29. Sobrinho LC, Cattell MJ, Glover RH, Knowles JC. Investigation of the dry and wet fatique properties of three all-ceramic crown systems. Int J Prosthodont. 1998. 11:255–262.