This article has been
cited by other articles in ScienceCentral.
Abstract
Chronic obstructive pulmonary disease (COPD) was the 3rd leading cause of death in 2012 worldwide. It is particularly severe in the elderly, who are at risk of death by coughing, mucous hypersecretion, and finally breathlessness. Recently, anti-COPD drug development has increased, and many animal screening systems have been studied. Tobacco smoke animal models are the best known animal screening system, but have several preparation requirements, such as a tobacco smoke generator and a separate facility to prevent smoke release. Accordingly, we evaluated the properties of a lipopolysaccharide (LPS) murine model for COPD screening and the effect of the time elapsed from 0 to 72 hr after LPS intranasal instillation on various biomarkers of COPD severity, such as WBC and neutrophils in bronchoalveolar fluid (BALF), IgE in serum, histopathology in the lung, and cytokines (IL-8, TNF-α, IFN-γ, and TGF-β) and chemokines (CCL-2, CXCL1, CXCL9, CXCL10, and CXCL11) in the respiratory system. Although from 48 hr after LPS treatment several factors which could be evaluated as biomarkers for COPD establishment such as WBC and neutrophil in BALF, IgE in serum, cytokines (IL-8, TNF-α, and IFN-γ), and chemokines (CCL-2, CXCL1, CXCL9, CXCL10, and CXCL11) increased at 72 hr the increment of important factors for COPD establishment such as IgE, fibrosis in the lung, and cytokines (IL-8, TNF-α, and IFN-γ) was more clear. Based on our results, we concluded that the optimal time after LPS intranasal instillation is 72 hr.
Go to :

Keywords: Chemokine, COPD, cytokine, elapse time, LPS
Chronic obstructive pulmonary disease (COPD) is an incurable respiratory disease caused by tobacco smoke, indoor and outdoor air pollution, occupational dust, fumes, etc. It usually occurs in individuals older than 40 or 50 years, and COPD patients have several typical symptoms, such as cough, mucous hypersecretion, and breathlessness [
1]. In 2014, the World Health Organization (WHO) reported that COPD was the third leading cause of death worldwide in 2012, and among the 10 leading causes of death in the world, it accounted for 5.6% of deaths [
2]. In 2015, approximately 3 million deaths were caused by this disease (5% of all deaths globally) [
1].
COPD occurrence begins with pulmonary inflammation and progresses to morphological changes, such as smooth muscle hypertrophy and goblet cell hyperplasia [
3], and changes in several factors, such as cytokines (interferon gamma (IFN-γ), tumor necrosis factor alpha (TNF-α), interleukin-8 (IL-8), transforming growth factor beta (TGF-β), etc.) and chemokines (chemokine c-c motif ligand (CCL)-2, chemokine C-X-C motif ligand (CXCL)-1, CXCL-9, CXCL-10, CXCL-11, etc.) [
4]. Finally, the loss of alveolar parenchyma, fibrosis, and emphysema occur in severe COPD patients, potentially leading to death [
5].
In order to discover drug candidates and to develop new drugs, it is most important to establish an animal screening system that is very similar to the human system, as we cannot apply drug candidates to humans without ensuring safety. Several animal screening systems with applications to COPD have been developed, such as a tobacco smoke model [
6], inhaled pollutants model [
7], protease model [
8], and lipopolysaccharide (LPS) model [
9].
The tobacco smoke model is one of the most well-known systems, but has several disadvantages, such as the long induction time and the need for a tobacco smoke generator and a ventilation system for smoke exhaust. An LPS model is easier to establish than a tobacco smoke model. In this study, we established a COPD murine model and evaluated it with respect to elapsed tie after LPS intranasal instillation.
Materials and Methods
Animal experiments
Using the same methods, two animal studies were conducted at different times. Eighty female BALB/c mice were purchased from Samtako (Osan, Korea) and based on the elapsed time after 20 µg/20 µL/head LPS intranasal instillation, they were divided into 5 groups: (1) 0 hr, (2) 3 hr, (3) 24 hr, (4) 48 hr, and (5) 72 hr (
Figure 1).
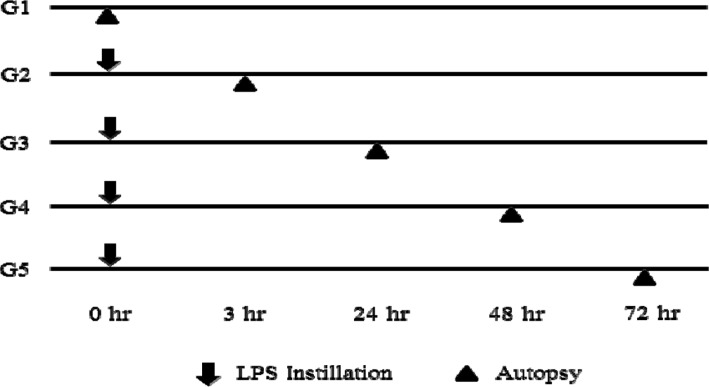 | Figure 1The animal schedule depended on the time elapsed after LPS intranasal instillation.
|
Ethics statement
All experiments were approved by the Institutional Animal Care and Use Committee at Dongshin University (Animal Study Approval No. 2014-08-01).
Bronchoalveolar fluid (BALF) and serum analysis
The same animal study was conducted twice to verify the repeatability. Four mice in the first study were used for BALF evaluation and other mice (N=4) were used for the other studies, such as the morphological analysis (H&E staining and Masson's trichrome staining) and expression analyses of specific proteins associated with COPD occurrence (IHC). The BALF analysis was conducted according to the methods of our previous study [
10]. BALF was collected from all mice after they were anesthetized with 50 mg/kg Zoletil (Virbac, Carros, France), and the tracheas were cannulated using disposable animal feeding needles. Lavages were performed with three 0.4-mL aliquots of cold phosphate-buffered saline (PBS). BALF samples were collected and immediately centrifuged at 3,000 rpm for 5 min (Sorvall Legend Micro 17R; Thermo Fisher Scientific, Inc., Waltham, MA, USA). The cell pellets were resuspended in PBS for total and differential cell counts. The numbers of total cells and differential cells were counted using a Hemavet Multi-Species Hematology System (Drew Scientific Inc., Waterbury, CT, USA). Some animals used for cell collection and others that were not used for cell collection were sacrificed by an additional Zoletil injection. IgE levels in the serum were measured using a specific mouse IgE ELISA Kit (BD Bioscience, catalog number 555248; San Jose, CA, USA) according to the manufacturer's protocols.
Histopathological analysis
A histopathological analysis was conducted as previously described [
10]. Lung tissues were fixed in 10% (v/v) formaldehyde solution, dehydrated in a graded ethanol series (99.9, 90, 80, and 70%), and embedded in paraffin. A total of 8 animals from 2 studies were used, with 4 animals per group for the histological analysis. Lung tissues were sectioned (4 µm) longitudinally and stained with H&E and Masson's trichrome.
Immunohistochemical analysis
An IHC analysis was conducted as previously described [
10]. Deparaffinized tissue sections were treated with 3% hydrogen peroxide in methanol for 10 min to remove endogenous peroxidase. Antigen retrieval was carried out with sodium citrate buffer (0.1 M) using the microwave method. The slides were incubated with normal serum to block nonspecific binding and then incubated for 1 h with primary antibodies (diluted 1:100 to 1:200), such as antibodies against IFN-γ (sc-74104; Santa Cruz Biotechnology, Dallas, TX, USA), TNF-α (MBS175453; MyBioSource, San Diego, CA, USA), TGF-β (MBS462142; MyBioSource), IL-8 (ab110727; Abcam, Cambridge, UK), CCL-2 (PAB16617; Abnova, Taipei, Taiwan), CXCL-1 (PAB8798,; Abnova), CXCL-9 (bs-2551R; Bioss, Woburn, MA, USA), CXCL-10 (bs-1502R; Bioss), and CXCL-11 (bs-2552R; Bioss). The slides were incubated for 10 min with biotinylated secondary antibodies (PK-7800; Vector Laboratories, Burlingame, CA, USA) and horseradish peroxidase-conjugated streptavidin. Signals were detected using the 3,3-diaminobenzidine tetrahydrochloride substrate chromogen solution, and the cells were counterstained with Mayer's hematoxylin. In order to evaluate the expression levels, after 5 circles were drawn with equal diameters in separate areas, without overlap, the positive cells were counted from 4 slides per group.
Statistical analysis
Results are expressed as means±standard deviation (SD). Group differences were evaluated by one-way analysis of variance, followed by Dunnett's multiple comparisons test. P<0.01 and P<0.05 were considered statistically significant.
Go to :

Results and Discussion
At 72 hr after LPS instillation, typical COPD changes in BALF were observed
After LPS intranasal instillation, the number of white blood cells (WBC) increased, and at 48 and 72 hr, a numerical increment in WBCs was observed (
Figure 2A). The change in neutrophil number among WBCs is closely related to COPD; specifically, the neutrophil population is significantly increased in COPD patients [
11]. Although the eosinophil population increased, the increase in neutrophils was statistically significantly greater compared to the population changes of other WBC components (
Figure 2B-D). Additionally, the neutrophil count was significantly higher than the counts in the other groups, such as Group 1 (0 hr), Group 2 (3 hr), or Group 3 (24 hr).
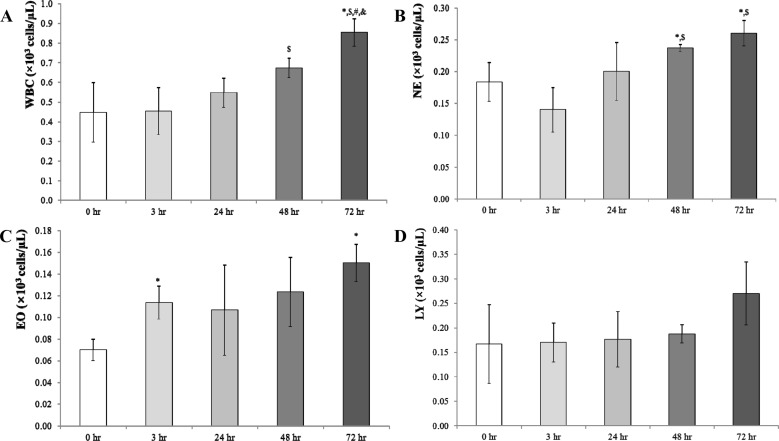 | Figure 2The changes of white blood cells (WBCs) and differential cells in BALF based on the elapsed time after LPS intranasal instillation. (A) WBCs were increased from 48 hr after LPS treatment in BALF. (B) The change in neutrophils was very similar to that of WBCs, and the difference in neutrophil counts was significant. (C) The change in eosinophils was similar to those of WBCs and neutrophils. (D) Lymphocytes did not change with respect to the elapsed time after LPS treatment. Bars represent the means±SD (N=16). *P<0.05 vs. 0 hr; $P<0.05 vs. 3 hr; #P<0.05 vs. 24 hr; &P<0.05 vs. 48 hr.
|
IgE levels depended on the time elapsed after LPS treatment
The IgE level is an important biomarker in COPD; specifically, in COPD patients, IgE levels are much higher than those of healthy people [
12]. Accordingly, the analysis of IgE is a very important method to evaluate the severity of COPD. A shown in
Figure 3, IgE levels in the serum changed depending on the elapsed time after LPS treatment. At 48 hr after LPS instillation, the level was the lowest among all time periods. At 72 hr after LPS intranasal instillation, IgE levels were significantly higher than those at other times.
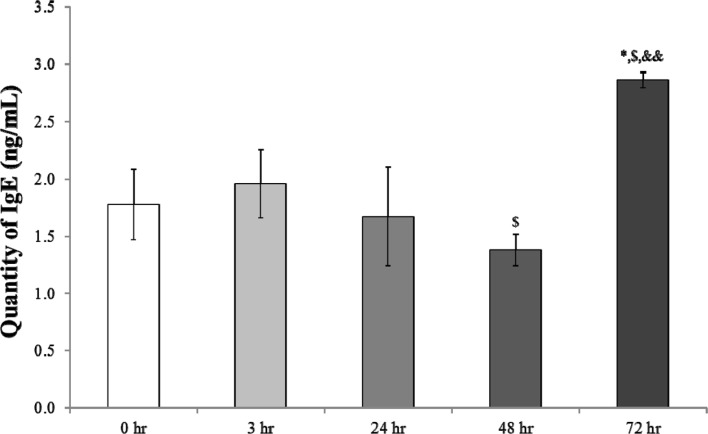 | Figure 3The alteration of IgE population based on the elapsed time after LPS intranasal instillation. IgE levels were lowest at 48 hr and highest at 72 hr. Bars represent the means±SD (N=16). *P<0.05 vs. 0 hr; $P<0.05 vs. 3 hr; &&P<0.001 vs. 48 hr.
|
At 48 hr or longer after LPS instillation, morphological changes associated with COPD were detected
As shown in
Figure 4A (H&E stain), typical morphological changes in the respiratory system were observed, such as inflammatory cell infiltration near the small airway (arrow) and alveolar rupture (
Figure 4Ae), and these changes increased depending on the elapsed time after LPS treatment. A representative change in COPD patients is fibrosis around the small airway [
5] and fibrosis was analyzed in the lung by Masson's trichrome staining (
Figure 4B). Until 24 hr, the fiber contents in the lung were very low (
Figure 4Ba, 4Bb and 4Bc), but at 48 and 72 hr, the fiber content increased significantly around the small airway (
Figure 4Bd and 4Be).
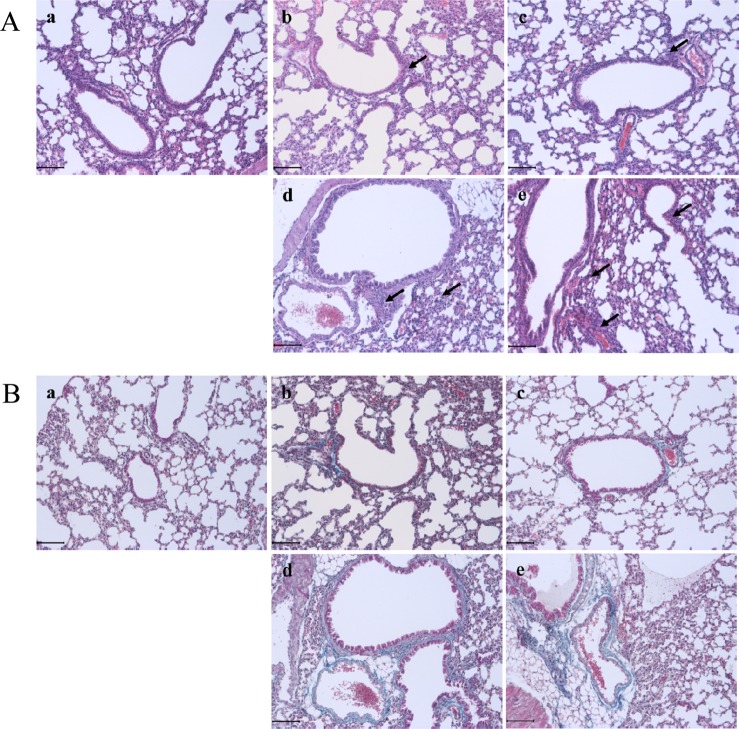 | Figure 4The morphological change of the lung based on the elapsed time after LPS intranasal instillation. (A) In the H&E-stained lung, LPS time-dependently upregulated COPD changes, such as inflammatory cell infiltration (arrows) around the small airway. (B) In the Masson's trichrome-stained lung, in a time-dependent manner, LPS stimulated lung fibrosis around the small airway. N=16. Scale Bar: 100 µm. arrow, inflammatory cell; a, 0 hr; b, 3 hr; c, 24 hr; d, 48 hr; e, 72 hr.
|
COPD-related cytokines increased in a time-dependent manner
To evaluate the expression changes of cytokines related to COPD occurrence the quantitative change of cytokines such as IL-8, TNF-α, IFN-γ, and TGF-β, was evaluated using with immunohistochemistry (IHC) (
Figure 5). The expression of all cytokines is increased significantly at 3, 24, 48, and 72 hr after LPS instillation. The changes in IL-8 levels were the largest among the four cytokines (
Figure 5A), and at 72 hr, the expression of IL-8 was dramatically increased. At 3 hr after LPS intranasal instillation, the level of TNF-α was dramatically improved compared to that in the control (
Figure 5B) and at all time points, the expression of TNF-α was increased. In particular, at 72 h, the level of TNF-α was significantly upregulated. Regardless of the elapsed time, IFN-γ was increased by LPS treatment (
Figure 5C); at every time point, the expression of IFN-γ was upregulated compared to that in the control group. Although there was little difference in the expression among elapsed times after LPS treatment, the expression of TGF-β was significantly upregulated in all LPS treatment groups (
Figure 5D). Although there were differences in the levels of all cytokines at every time point, the most susceptible cytokine in response to LPS treatment was TGF-β, and the most useful cytokine for the evaluation of COPD establishment was IL-8.
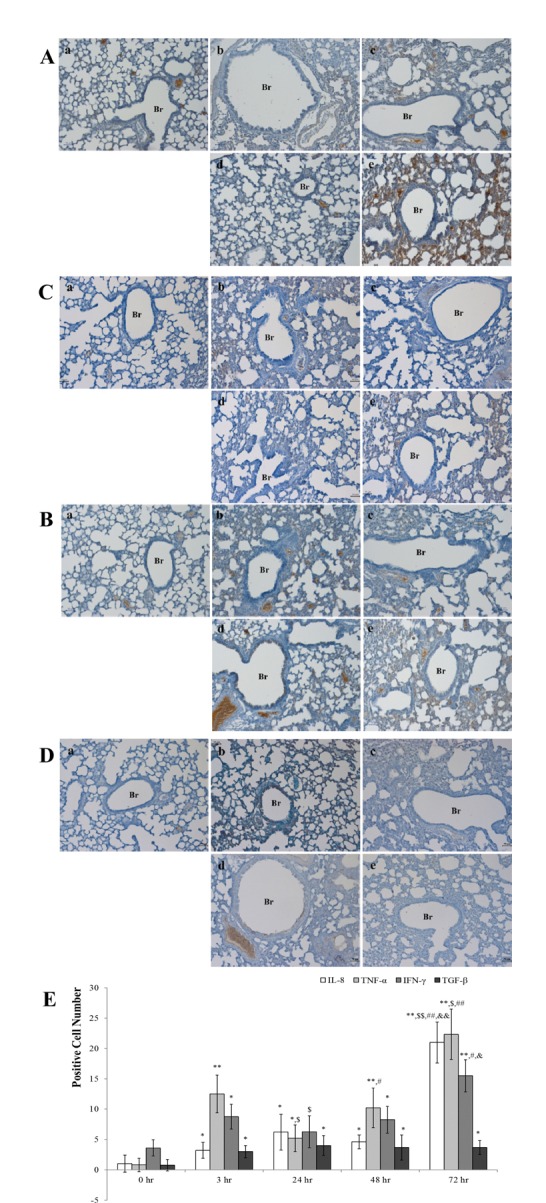 | Figure 5The changes of cytokines-related with COPD establishment based on the elapsed time after LPS intranasal instillation. (A) The expression of IL-8 was time-dependently upregulated by LPS treatment. There was a minor difference between levels at 48 hr and at 72 hr. (B) Although the level of TNF-α was significantly increased at 3 hr, its expression then decreased slightly, and at 72 hr, it dramatically increased. (C) The changes in IFN-γ levels were similar to those of TNF-α; the level at 3 hr was dramatically upregulated, but at 48 hr, a slight down-regulation was observed. At 72 hr, the expression of TNF-α was the highest compared to the others. (D) The changes in the level of TGF-β were different from those of the other cytokines, such as IL-8, TNF-α, and IFN-γ. There was no difference between the expression levels with respect to the elapsed time, but LPS treatment significantly increased the level of TGF-β compared to the levels observed at 0 h. N=16. Scale Bar: 50 µm. arrow, inflammatory cell; a, 0 hr; b, 3 hr; c, 24 hr; d, 48 hr; e, 72 hr.; *P<0.05 vs. 0 hr; **P<0.001 vs. 0 hr; $P<0.05 vs. 3 hr; $$P<0.01 vs. 3 hr; #P<0.05 vs. 24 hr; ##P<0.01 vs. 24 hr; &P<0.05 vs. 48 hr; &&P<0.001 vs. 48 hr.
|
LPS regulated COPD-related chemokines
Despite minimal changes at 3 hr, at both 48 and 72 hr, CCL-2 levels (
Figure 6Ad and 6Ae) were much higher than those at 24 hr (
Figure 6Ac). Only CXCL1 (
Figure 6Bb) and CXCL11 (
Figure 6Eb) were upregulated at 3 hr. The changes in CXCL1 and CXCL11 exhibited a key difference; CXCL1 increased in a time-dependent manner (
Figure 6B), while CXCL11 only increased in response to LPS treatment (
Figure 6E). These findings suggest that the severity of COPD can be assessed based on the level of CXCL1, but changes in the level of CXCL11 could indicate LPS treatment or a lack of treatment. At 48 or 72 hr, the expression of CXCL9 was not upregulated, unlike CCL-2, but the level of CXCL9 increased in a time-dependent manner (
Figure 6C). Changes in CXCL10 expression exhibited a similar pattern to changes in CXCL9 (
Figure 6D). Until 3 hr (
Figure 6Da and 6Db), there was no difference in expression, but after 24 hr, the level of CXCL10 (
Figure 6Dc, 6Dd and 6De) increased significantly.
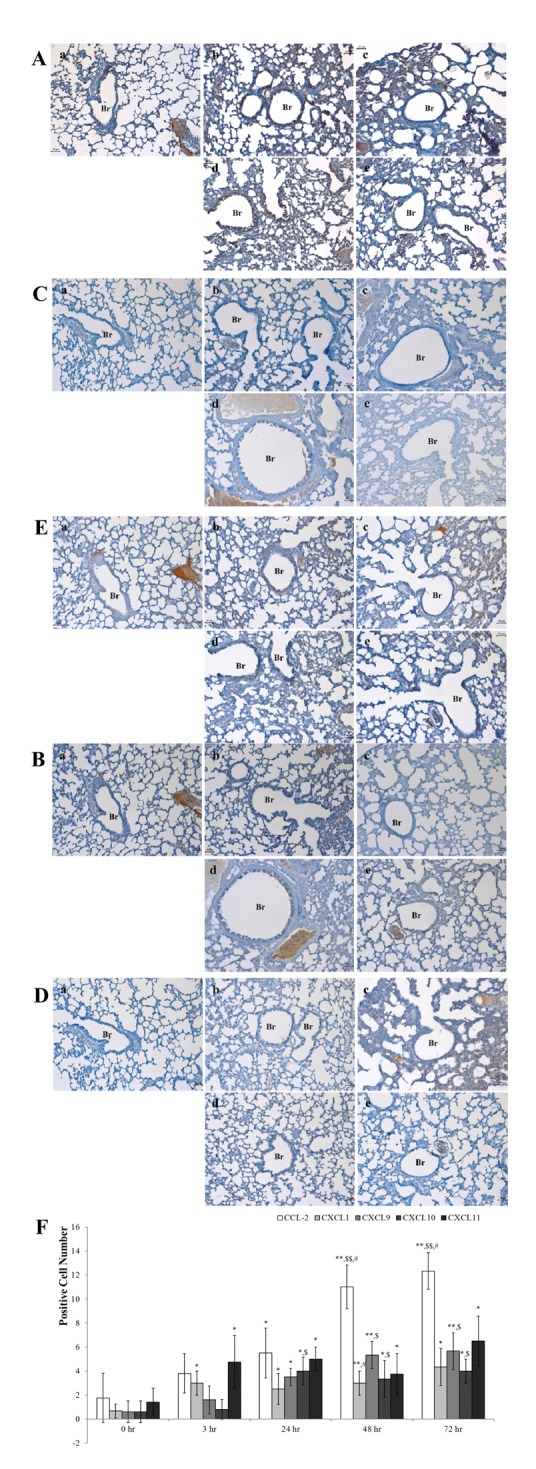 | Figure 6The changes of chemokines-related with COPD occurrence based on the elapsed time after LPS intranasal instillation. (A) LPS stimulated increases in the CCL-2 level, and there was a significant difference in levels between 48 and 72 hr. (B) LPS induced changes in the CXCL1 level compared to the levels observed in the control group. (C) Although the upregulation of CXCL9 was not much greater than that observed for CCL-2, it increased in a time-dependent manner. (D) After 24 hr, the expression of CXCL10 was increased. (E) The change in CXCL11 expression exhibited a similar pattern to the change in TGF-β. The change in CXCL11 was only dependent on LPS treatment. N=16. Scale Bar: 50 µm. arrow, inflammatory cell; a, 0 hr; b, 3 hr; c, 24 hr; d, 48 hr; e, 72 hr.; *P<0.05 vs. 0 hr; **P<0.001 vs. 0 hr; $P<0.05 vs. 3 hr; $$P<0.01 vs. 3 hr; #P<0.05 vs. 24 hr.
|
COPD is a chronic pulmonary disease and a leading cause of death in the elderly [
2]. It is related to various irritants, such as tobacco smoke, air pollutants, occupational dust, chemicals (such as vapors, irritants, and fumes), and infection [
1]. These irritants stimulate the proliferation of COPD-related factors, such as inflammatory cells (neutrophils, macrophages, etc.), cytokines (IL-8, TNF-α, IFN-γ, TGF-β, etc.), and chemokines (CCL-2, CXCL1, CXCL9, CXCL10, CXCL11, etc.) [
4]. Proliferation factors lead to functional changes, such as fibrosis, alveolar wall destruction (emphysema), mucous hypersecretion, etc., and finally can lead to patient death [
5].
Although COPD is an incurable disease, many recent efforts have been made to identify drug candidates [
13]. To develop new drugs, the establishment of a screening system for analyses of therapeutic effects is a very important step. There are several animal models for COPD drug development, such as a tobacco smoke model [
6], inhaled pollutants model [
7], protease model [
8], and LPS model [
9]. Although much is known about LPS, a COPD murine model that depends on elapsed time has not been established. Especially Håkansson HF et al. reported the LPS inhalation model which 5 mg/mL LPS was challenged using with nebulizer for 10 min but in this study 20 µg/20 µL/head LPS was intranasal instilled only using with micropipette not with nebulizer. Our method might be more useful than Håkansson HF's method as the amount used was smaller and it didn't need the equipment for LPS challenge.
We examined a COPD animal model according to elapsed time from 0 to 72 hr after LPS intranasal instillation. In BALF, the populations of WBCs and neutrophils increased in a time-dependent manner after 48 hr of LPS treatment. The level of IgE in the serum changed over time; it was lowest at 48 hr and highest at 72 hr. Similarities to the lungs of COPD patients were observed after 48 hr, such as inflammatory infiltration, alveolar wall destruction (emphysema), and fibrosis around small airways. The cytokines related to COPD occurrence, such as IL-8, TNF-α, IFN-γ, and TGF-β, were analyzed by IHC. All increased in a time-dependent manner. In particular, IL-8 might be the most important biomarker for the evaluation of the COPD severity, as its expression increased in a time-dependent manner. Additionally, TGF-β might be the most important factor for the assessment of pulmonary inflammation, as after 3 hr, its levels improved significantly. Several COPD-related chemokines, such as CCL-2, CXCL1, CXCL9, CXCL10, and CXCL11, were measured by IHC. The results obtained for each cytokine were very similar. The levels of CXCL1 changed in a time-dependent manner, suggesting that it is a potential biomarker to evaluate COPD severity. The expression of CXCL11 might be an indicator of LPS usage, as after 3 hr, it was dramatically upregulated.
Previous analyses determined the irritant-induced COPD mechanism explaining the relationships between cytokine/chemokine levels and COPD symptoms [
14]. TGF-β released from pulmonary epithelial cells could induce fibrosis around the small airway, and several chemokines, such as CXCL9, CXCL10, and CXCL11, secreted from epithelial cells could destroy the alveolar wall (emphysema). CXCL1 from macrophages could stimulate protease secretion, and CCL-2 could stimulate monocytes and macrophages via a feed-back system. When a disease animal model is established, the correlation between the animal model and human patients is very important. As the COPD severity increases, various biomarkers should change. In a disease animal model, biomarker discovery is important to evaluate disease severity. In this study, we analyzed changes in biomarkers with respect to elapsed time after LPS intranasal instillation. Although at 48 hr, the typical changes related to COPD establishment were observed, such as WBCs and neutrophils in BALF, histopathological changes in the lung, and cytokine and chemokine expression, the levels of IgE increased at 72 hr and accordingly the proper time after LPS intranasal instillation is 72 hr.
Go to :

Acknowledgment
This research was supported by a grant (13182 MFDS667) from Ministry of Food and Drug Safety, Korea in 2013.
Go to :

Notes
Go to :

References
1. World Health Organization. November 2016 Chronic obstructive pulmonary disease (COPD). 2016. Fact sheet.
2. World Health Organization. The top 10 causes of death. 2014. Fact sheet No310.
3. Hogg JC, Chu F, Utokaparch S, Woods R, Elliott WM, Buzatu L, Cherniack RM, Rogers RM, Sciurba FC, Coxson HO, Paré PD. The nature of small-airway obstruction in chronic obstructive pulmonary disease. N Engl J Med. 2004; 350(26):2645–2653. PMID:
15215480.

4. Barnes PJ. The cytokine network in chronic obstructive pulmonary disease. Am J Respir Cell Mol Biol. 2009; 41(6):631–638. PMID:
19717810.

5. Hogg JC, Timens W. The pathology of chronic obstructive pulmonary disease. Annu Rev Pathol. 2009; 4:435–459. PMID:
18954287.

6. Churg A, Cosio M, Wright JL. Mechanisms of cigarette smoke-induced COPD: insights from animal models. Am J Physiol Lung Cell Mol Physiol. 2008; 294(4):L612–L631. PMID:
18223159.

7. Maes T, Provoost S, Lanckacker EA, Cataldo DD, Vanoirbeek JA, Nemery B, Tournoy KG, Joos GF. Mouse models to unravel the role of inhaled pollutants on allergic sensitization and airway inflammation. Respir Res. 2010; 11(1):7. PMID:
20092634.

8. Birrell MA, Wong S, Hele DJ, McCluskie K, Hardaker E, Belvisi MG. Steroid-resistant inflammation in a rat model of chronic obstructive pulmonary disease is associated with a lack of nuclear factor-kappaB pathway activation. Am J Respir Crit Care Med. 2005; 172(1):74–84. PMID:
15805185.
9. Håkansson HF, Smailagic A, Brunmark C, Miller-Larsson A, Lal H. Altered lung function relates to inflammation in an acute LPS mouse model. Pulm Pharmacol Ther. 2012; 25(5):399–406. PMID:
22975080.

10. Bang MA, Seo JH, Seo JW, Jo GH, Jung SK, Yu R, Park DH, Park SJ. Bacillus subtilis KCTC 11782BP-produced alginate oligosaccharide effectively suppresses asthma via T-helper cell type 2-related cytokines. PLos One. 2015; 10(7):e0130510. PMID:
26132168.

11. Gompertz S, O'Brien C, Bayley DL, Hill SL, Stockley RA. Changes in bronchial inflammation during acute exacerbations of chronic bronchitis. Eur Respir J. 2001; 17(6):1112–1119. PMID:
11491152.

12. Samaha HMS, Elsaid AR, NasrEldin E. Total serum IgE level in COPD patients. Egypt J Chest Dis Tuberc. 2015; 64(3):573–577.

13. America's Biopharmaceutical Research Companies 2012. Medicines in development COPD;2012. Report.
14. Barnes PJ. Immunology of asthma and chronic obstructive pulmonary disease. Nat Rev Immunol. 2008; 8(3):183–192. PMID:
18274560.

Go to :
