Abstract
The inhibitory effects of Asparagus cochinchinensis against inflammatory response induced by lipopolysaccharide (LPS), substance P and phthalic anhydride (PA) treatment were recently reported for some cell lines and animal models. To evaluate the hepatotoxicity and nephrotoxicity of A. cochinchinensis toward the livers and kidneys of ICR mice, alterations in related markers including body weight, organ weight, urine composition, liver pathology and kidney pathology were analyzed in male and female ICR mice after oral administration of 150, 300 and 600 mg/kg body weight/day saponin-enriched extract of A. cochinchinensis (SEAC) for 14 days. The saponin, total flavonoid and total phenol levels were found to be 57.2, 88.5 and 102.1 mg/g in SEAC, respectively, and the scavenging activity of SEAC gradually increased in a dose-dependent manner. Moreover, body and organ weight, clinical phenotypes, urine parameters and mice mortality did not differ between the vehicle and SEAC treated group. Furthermore, no significant alterations were measured in alkaline phosphatase (ALP), alanine aminotransferase (ALT), aspartate aminotransferase (AST), lactate dehydrogenase (LDH), blood urea nitrogen (BUN) and the serum creatinine (Cr) in the SEAC treated group relative to the vehicle treated group. Moreover, the specific pathological features induced by most toxic compounds were not observed upon liver and kidney histological analysis. Overall, the results of the present study suggest that SEAC does not induce any specific toxicity in the livers and kidneys of male and female ICR mice at doses of 600 mg/kg body weight/day.
A. cochinchinensis is a perennial herb belonging to the Liliaceae family that is widely distributed in China, Japan and Korea, and has long been considered a therapeutic drug because of its anti-inflammatory, diuretic, antiseptic, antitussive, antibacterial, nervine, sialogogue, antipyretic and stomachic effects. A. cochinchinensis is also administered in combination with other herbs as a medicine to treat lung disease, immune system-associated diseases and aging [12].
Among various therapeutic activities, the anti-inflammatory activity of the extract of A. cochinchinensis roots had been investigated in previous studies to generate scientific evidence and found to significantly inhibit production of pro-inflammatory cytokines and tumor necrosis factor (TNF)-α in lipopolysaccharide (LPS)- and substance P-stimulated mouse astrocytes [3]. Moreover, the nitric oxide (NO) concentration decreased in response to compounds 2, 3 and 4 from the ethanol extract of A. cochinchinensis roots in LPS-stimulated BV-2 microglial cells. These compounds also greatly decreased indicators of skin inflammation progression including the degree of ectopic edema, ear thickness, cytokine secretion and myeloperoxidase (MPO) activity in a skin inflammation model induced with 12-O-tetradecanoyl-phorbol-13-acetate (TPA) [4]. Furthermore, the aqueous extract of A. cochinchinensis suppressed TNF-α-induced cytotoxicity of HepG2 cells [5], enhanced superoxide dismutase (SOD) activity and the spleen index, and decreased the level of malondialdehyde (MDA) in mice [1]. Moreover, saponin-enriched extract of A. cochinchinensis (SEAC, ethyl acetate extrect) successfully suppressed skin inflammation of IL-4/Luc/CNS-1 transgenic mice induced by phthalic anhydride (PA) treatment [6]. Overall, the results of the aforementioned studies suggest that SEAC can be considered a potential drug for inflammation related diseases in humans. However, its toxicity toward specific organs of mice has not been investigated to date, despite the increased attention and publications regarding the therapeutic effects of SEAC.
In this study, we investigated SEAC toxicity in the livers and kidneys of male and female ICR mice following short-term treatment. The results indicate that there is a scientific basis for determining the optimal concentration of SEAC as a therapeutic drug for the treatment of human chronic diseases.
The roots of A. cochinchinensis used in this study were obtained from Gochang National Agricultural Cooperation Federation in Korea and dried in a drying machine (FD5510S and FD5520S, Ilshinbiobase Co., Dongducheon, Korea) at 60℃. Voucher specimens of A. cochinchinensis roots (WPC-14-003) were deposited in the functional materials bank of the Wellbeing RIS Center at Pusan National University. Dried roots were reduced to powder using a pulverizer (MF-3100S, Hanil Electric Co., Seoul, Korea), after which SEAC was obtained at 50℃ for 24 h in a 1:10 fixed liquor ratio of solid powder of A. cochinchinensis to ethyl acetate solvent ratio using circulating extraction equipment (SHWB-30/45, Woori Science Instrument Co., Pocheon, Korea). These solutions were subsequently passed through a 0.4 µm filter, after which they were concentrated by vacuum evaporation and lyophilization using circulating extraction equipment (IKA Labortechnik, Staufen, Germany) (Figure 1A). Finally, the collected SEAC powder was dissolved in 0.5% Tween 20 Solution (in dH2O) to 600 mg/kg, then further diluted to the required concentration.
Total phenolic contents in SEAC were determined using the Folin-Ciocalteu method as previously described [7]. Briefly, a mixture of SEAC solution (1 mL) and Folin-Ciocalteu reagent (5mL, Sigma-Aldrich Co., St. Louis, MO, USA) was incubated at room temperature for 5 min. This mixture was subsequently added to 15 mL of 20% Na2CO3 and vortexed for 30 sec, after which the absorbance was repeatedly measured at 765 nm using a Versa-max plate reader (Molecular Devices, Sunnyvale, CA, USA). A standard calibration curve was then made using different concentrations of gallic acid (Sigma-Aldrich Co.), and the concentration of total phenolic contents in SEAC was presented as gallic acid equivalent (mg) of extract.
The flavonoid contents in SEAC were measured as previously described [8]. Briefly, 200 µL of several different concentrations of SEAC were mixed with 60 µL of 5% NaNO2 (Sigma-Aldrich Co.) and 60 µL of 10% AlCl3 (Sigma-Aldrich Co.). Following incubation at 25℃, the absorbance was measured using a Versa-max plate reader (Molecular Devices). A standard calibration curve was then made using different concentrations of catechin (Sigma-Aldrich Co.). The flavonoid contents in SEAC are presented as catechin equivalents (mg) of extract.
Finally, the total saponins content was determined using the Helaly method as previously described [9]. Briefly, extract of n-butanol was redissolved in 0.5 mL of 80% MeOH, then mixed with 0.5 mL of 8% vanillin in ethanol and 5 mL of 72% H2SO4 in water. Next, these mixtures were placed in a 60℃ water bath for 20 min, then cooled at 0℃ for 5 min, after which the absorbance was measured at 544 nm using a Versa-max plate reader (Molecular Devices). The saponin content was calculated from a calibration curve constructed using purified saponin standard (Sigma-Aldrich Co.).
The scavenging activity of 2,2-diphenyl-1-picrylhydrazyl (DPPH) radical was measured as previously described [10]. Briefly, each 100 µL sample in eleven different concentrations of SEAC (0-2,000 µg/mL) was mixed with 100 µL DPPH (0.1 mM; Sigma-Aldrich Co.) in 95% ethanol solution or 100 µL 95% ethanol solution, then incubated for 30 min at room temperature. The absorbance of the reaction mixture was subsequently measured at 517 nm using a VersaMax plate reader (Molecular Devices). The DPPH radical scavenging activity of the SEAC was expressed as the percent decrease in absorbance relative to the control. The half maximal inhibitory concentration (IC50) was defined as the concentration of substrate that causes a 50% loss in DPPH activity.
The animal protocol used for this experiment was reviewed and approved by the Pusan National University-Institutional Animal Care and Use Committee (PNU-IACUC; Approval Number PNU-2015-0779). All mice were handled in the Pusan National University-Laboratory Animal Resources Center, which is accredited by the Korea Food and Drug Administration (KFDA, Accredited Unit Number-000231) and AAALAC International (Accredited Unit Number; 001525). Seven-week-old female and male ICR mice were purchased from Samtako Bio Korea (Osan, Korea) and provided with ad libitum access to a standard irradiated chow diet (Samtako Bio Korea) consisting of moisture (12.5%), crude protein (25.43%), crude fat (6.06%), crude fiber (3.9%), crude ash (5.31%), calcium (1.14%) and phosphorus (0.99%) and water throughout the feeding study. Before starting the animal experiment, the mice were given at least 1 week to adapt to the experimental environment. During the experiment, mice were maintained in a specific pathogen-free state under a strict light cycle (lights on at 08:00 hr and off at 20:00 hr) at 23±2℃ and 50±10% relative humidity.
ICR mice (n=40) were assigned to one of the following four groups: vehicle treated group (n=10), low dosage SEAC treated group (SEACL, n=10), middle dosage SEAC treated group (SEACM, n=10), high dosage SEAC treated group (SEACH, n=10). As a control, one group of ICR mice received an equivalent volume of water via gavage daily (vehicle treated group), whereas the other groups received 150 mg (SEACL), 300 mg (SEACM) and 600 mg (SEACH)/kg body weight/day of SEAC via oral gavage. The administration period for the toxicity test was based on the Guideline for Drug Toxicity published by the Korea Food and Drug Administration. After SEAC treatment for 14 days, all mice were immediately sacrificed using CO2 gas, after which urine, serum and tissue samples were prepared.
Several pathological signs including dead and abnormal behaviors were recorded more than twice a day for 14 days. In addition, alterations in body weight were observed using an electronic balance (Mettler Toledo, Greifensse, Switzerland) every day according to the KFDA guidelines. The weights of eight organs (brain, ovary (or testis), kidney, spleen, liver, thymus, heart and lung) collected from the sacrificed mice were determined using the same method employed to detect the body weight.
All mice were sacrificed using CO2 gas at 24 h after the final administration, at which time urine was collected from their bladders and assayed for bilirubin, urobilinogen, ketones, protein, pH, specific gravity and leucocytes using an URiSCAN optima II urine analyzer (Yeongdong Electronics Co., Ltd., Yongin, Korea). All assays were conducted in duplicate using fresh urine.
After fasting for 8 h, whole blood from each mouse in all groups was collected from their abdominal veins and incubated for 30 min at room temperature in a serum separating tube (BD Container, Franklin Lakes, NJ, USA). Serum was then obtained by centrifugation at 1,500×g and analyzed for alkaline phosphatase (ALP), alanine aminotransferase (ALT), aspartate aminotransferase (AST), lactate dehydrogenase (LDH), blood urea nitrogen (BUN) and creatinine (Cr) using an automatic biochemical analyzer (BS-120, Mindray, China). All assays were conducted in duplicate using fresh serum.
Liver and kidney tissues were dissected from mice and fixed in 4% neutral buffered formaldehyde (pH 6.8) overnight, after which each liver was dehydrated and embedded in paraffin wax. Next, a series of liver sections (4 µm) was cut from paraffin-embedded tissue using a Leica microtome (Leica Microsystems, Wetzlar, Germany). These sections were then deparaffinized with xylene, rehydrated with ethanol at a graded decreasing concentration of 100–70%, and finally washed with distilled water. The slides with liver sections were stained with hematoxylin (Sigma-Aldrich Co.) and eosin (Sigma-Aldrich Co.), then washed with dH2O, after which pathological changes were measured using the Leica Application Suite (Leica Microsystems).
Significant differences between vehicle and SEAC treated ICR mice were identified by one-way analysis of variance (ANOVA) using SPSS for Windows, Release 10.10, Standard Version (SPSS Inc., Chicago, IL, USA). All values are reported as the means±standard deviation (SD) and a p<0.05 was considered significant.
The main components of SEAC were analyzed to verify their biochemical properties. Among these compounds, crudal saponins was found to be present in the greatest abundance (57.2 mg/g), while the levels of flavonoid and polyphenol were 88.5 and 102.1 µg/g, respectively (Figure 1). To investigate the inhibitory activity of SEAC against oxidative stress, the scavenging activity of SEAC against DPPH radicals was measured. The scavenging activity was gradually increased in a dose-dependent manner and the IC50 value was 835.90 µg/mL (Figure 1C). Therefore, the present results suggest that SEAC contain various compounds with high anti-oxidant activity.
To investigate whether SEAC administration affects phenotypic toxicity, the clinical phenotypes and mortality were observed in male and female ICR mice for 14 days. No mice treated with any of the investigated concentrations of SEAC showed significant pathological symptoms including body weight loss, hypokinesia, melancholy, gait abnormality and tremors. No significant differences in body weight were observed between the vehicle and SEAC treated group throughout the 14 day experimental period, although the male group was higher than female group (Table 1). Moreover, dead mice were not observed in the SEAC treated group throughout the experimental period. These results show that SEAC does not induce any significant pathological symptoms or mortality and thus the minimum lethal dose (MLD) is higher than 600 mg/kg of SEAC.
To investigate the effects of SEAC administration on the weights of organs and urine composition of animals, the weights of eight organs and seven urine factors were measured in male and female ICR mice treated with SEAC. No significant differences in the weights of any of the organs were observed between the vehicle and SEAC treated groups (Table 1). Moreover, the SEAC treated ICR mice showed no morphological changes in the brain, ovary (or testis), kidney, spleen, liver, thymus, heart and lungs when compared to the vehicle treated group. Finally, similar levels of urine parameters (bilirubin, urobilinogen, leucocytes, protein, pH, specific gravity and ketones) were measured in the vehicle and SEAC treated group (Table 2). These results suggest that SEAC treatment for 14 days does not induce any toxicity toward organ weights or urine parameters in male and female ICR mice.
To investigate whether SEAC treatment for 14 days induced hepatotoxicity in ICR mice, alteration of several enzymes related to liver metabolism were measured in the serum of male and female ICR mice treated with SEAC. No significant differences in liver toxicity indicators such as ALP, AST, ALP and LDH were observed between the vehicle and SEAC treated group (Table 3). Furthermore, we investigated whether the lack of alterations of toxicity indicators in serum could completely reflect the histopathological structure of liver tissue. To achieve this, liver sections were stained with H&E and observed microscopically. There were no significant pathological changes such as inflammation, necrosis, apoptosis, or fibrosis observed in the liver tissue of SEAC treated groups. Moreover, the histological structure was very similar to that of the vehicle treated male and female ICR mice (Figure 2). Therefore, these results suggest that SEAC administration does not induce hepatotoxicity in the liver of male and female ICR mice.
Finally, the nephrotoxicity against the treatment of SEAC was investigated in male and female ICR mice using serum biochemical and histological analyses of the kidney. BUN and Cr were maintained at constant levels between the vehicle treated group and the SEAC treated group without any significant alteration (Table 3). In addition, no specific pathological symptoms including degeneration and necrosis of the glomerulus and renal tubes were observed in the kidney tissue of any SEAC treated groups (Figure 3). Taken together, these results suggest that SEAC treatment for 14 days does not induce nephrotoxicity in male or female ICR mice.
There are still many issues that must be overcome to successfully develop new drugs, including the large-scale procurement of sufficient source materials for bulk production, the potency and inherent toxicity of many natural products, and the development of suitable vehicles and dosing schedules for the administration of new drugs to clinical patients [11], although natural products have long been contributed as a source of leads during the development of therapeutic drugs available for the treatment of human diseases [1213]. It is very important to consider toxicological problems when developing novel drugs for treatment of human disease and to extend the therapeutic potential of conventional drugs [14]. In this study, we investigated the hepatotoxicity and nephrotoxicity of SEAC in ICR mice after short term administration to verify the possibility of using SEAC for clinical study because therapeutic effects of SEAC have been reported in inflammatory disease. The results presented here are the first to demonstrate that treatment with SEAC did not induce significant toxicity against the livers and kidneys of male and female ICR mice at less than 600 mg/kg body weight.
Saponins are well known secondary metabolites of glycosidic nature that are abundantly distributed in higher plants, as well as some animal sources [1516]. These compounds show varying ranges of pharmacological and biological activities such as anti-inflammatory, expectorant, vasoprotective, hypocholesterolemic, immunomodulatory, hypoglycemic, molluscicidal, anti-fungial, anti-parasitic and many others activities, while they also used for adjuvant production [171819]. Despite their beneficial effects against clinical diseases, several studies have reported toxicity of saponin mixtures and saponin-enriched extracts. The oral administration (100 and 200 mg/kg) of water extract of saponins from Argania spinose for 3 months induced increased blood Cr levels and renal pathology without other pathological alterations [20]. However, treatment with a saponin mixture isolated from Schefflera leucantha Viguier resulted in no significant differences in the histopathological structure of a control group and treated group, although BUN, Cr, AST, ALT and ALP levels were slightly increased in the group treated with the saponin mixture [21]. Furthermore, lambs treated with saponin-containing Yucca schidigera Roetzl juice (63 and 126 mg saponin/kg) showed acute tubular necrosis, dehydration and watery content in the gastrointestinal tract, as well as increased serum Cr and urea levels [22]. A 13 week subchronic toxicity study revealed that the lowest-observed-adverse-effect level (LOAEL) of saponin-rich and isoflavones-containing soybean extract was 707.2 and 751.8 mg/kg body weight/day, respectively [23]. In the present study, we investigated the hepato-toxicity and nephrotoxicity of ICR mice treated with 150 (8.5 mg/kg of saponin), 300 (17 mg/kg of saponin) and 600mg/kg (34mg/kg of saponin) of SEAC. No significant toxicity was detected based on the body or organ weight, urine parameters, serum parameters or histological structure. These differences may have occurred because the concentration of saponins administered to animals was higher in most of the studies referenced above (>63 mg) than in the present study (34 mg).
The liver is well known as a primary target responsible for metabolism of various compounds including natural products, chemicals, drugs and alcohol, food additives and pesticides [24]. Some toxicity markers including the degree of steatosis, lobular inflammation, hepatocellular ballooning, and fibrosis can be detected during evaluation of liver toxicity caused by various compounds [25]. Among these, alterations in the levels of ALT, AST, ALP and LDH have been widely applied to measure liver toxicity [2627282930]. Following treatment with saponin mixture isolated from Schefflera leucantha Viguier, the level of AST, ALT and ALP increased [21]. However, the AST levels were slightly decreased in male rats treated with 2.5 and 5% of saponin-enriched and isoflavones-containing soybean extract, while ALT and ALP were maintained at constant levels [23]. Our results were very similar to those of a study in which animals were treated with soybean extract, although there are a few difference in the AST level.
In toxicological studies, the kidney is commonly considered an indicator organ for toxicity [31]. Generally, kidney dysfunction is caused by cellular damage induced by metabolites of the toxic chemicals that are excreted from the kidneys [32], and the severity of such damage is evaluated based on increases in red blood cells in the glomerulus and tubule luminal diameter upon histological analysis [33]. Other key markers for nephrotoxicity are serum levels of BUN and Cr that were dramatically increased in patients with renal trauma [34]. Therefore, the above parameters have been widely applied to evaluate kidney toxicity during preclinical and clinical experiments [35]. Moreover, in previous studies, treatment with high concentrations saponin and saponin mixtures induced significant alterations in the kidney histological structure and the level of serum markers [36]. Following saponin administration (200 mg/kg), increased Cr levels and deterioration of renal tubules were observed [2037]. Moreover, the levels of BUN and Cr increased in groups treated with saponin mixture isolated from S. leucantha Viguier (1,000 mg/kg for 14 days), saponin-containing Y. schidigera Roetzl juice (3,000 mg/kg for 21 days) and saponin rich soybean extract (5% of diet for 13 weeks) [212223]. In the present study, no significant alterations in kidney weights or histopathological structure were detected throughout the experiment. Additionally, the levels of BUN and Cr remained constant in the serum of ICR mice treated with SEAC for 7 days. Our results differ from those of previous studies showing kidney toxicity of saponin related mixtures. These difference can be caused by differences in the dose of saponin containing mixtures applied to animals.
Taken together, the results of the present study suggest that SEAC with high antioxidant activity has no toxic effects on liver and kidney tissues of male and female ICR mice. Furthermore, these findings provide vital information regarding the development of SEAC as a therapeutic drug for the treatment of various inflammatory diseases.
Acknowledgments
This study was supported by grants to Professor Dae Youn Hwang from the Korea Institute of Planning Evaluation for Technology of Food, Agriculture, Forestry and Fisheries (Grant no. 114034-03-1-HD030).
References
1. Xiong D, Yu LX, Yan X, Guo C, Xiong Y. Effects of root and stem extracts of Asparagus cochinchinensis on biochemical indicators related to aging in the brain and liver of mice. Am J Chin Med. 2011; 39(4):719–726. PMID: 21721152.
2. Xiao PG. Modern chinese material medica. Beijing: Chemical Industry Press;2002. p. 150.
3. Kim H, Lee E, Lim T, Jung J, Lyu Y. Inhibitory effect of Asparagus cochinchinensis on tumor necrosis factor-alpha secretion from astrocytes. Int J Immunopharmacol. 1998; 20(4-5):153–162. PMID: 9730251.
4. Lee DY, Choo BK, Yoon T, Cheon MS, Lee HW, Lee AY, Kim HK. Anti-inflammatory effects of Asparagus cochinchinensis extract in acute and chronic cutaneous inflammation. J Ethnopharmacol. 2009; 121(1):28–34. PMID: 18691647.
5. Luo J, Long QD, Li CX, Li L, Huang NH, Nie M, Tang PX. Comparison of antitussive, expectorant and anti-asthmatic effect between ALWB and ACM. J GuiYang Med Coll. 1998; 23:132–134.
6. Sung JE, Lee HA, Kim JE, Go J, Seo EJ, Yun WB, Kim DS, Son HJ, Lee CY, Lee HS, Hwang DY. Therapeutic effect of ethyl acetate extract from Asparagus cochinchinensis on phthalic anhydride-induced skin inflammation. Lab Anim Res. 2016; 32(1):34–45. PMID: 27051441.
7. Singleton VL, Rossi JA. Colorimetry of total phenolics with phosphomolybdic-phosphotungstic acid reagents. Am J Enol Vitic. 1965; 16(3):144–158.
8. Zhishen J, Mengcheng T, Jianming W. The determination of flavonoid contents in mulberry and their scavenging effects on superoxide radicals. Food Chem. 1999; 64:555–559.


9. Helaly FM, Soliman HSM, Soheir AD, Ahmed AA. Controlled release of migration of molluscicidal saponin from different types of polymers containing Calendula officinalis. Adv Polym Techn. 2001; 20(4):305–311.
10. Oh H, Ko EK, Kim DH, Jang KK, Park SE, Lee HS, Kim YC. Secoiridoid glucosides with free radical scavenging activity from the leaves of Syringa dilatata. Phytother Res. 2003; 17(4):417–419. PMID: 12722154.
11. Cragg GM, Newman DJ. Drug discovery and development from natural products: the way forward. In : 11th NAPRECA, Symposium Book of Proceedings; Antananarivo. 2005. p. 56–69.
12. Newman DJ. Natural products as leads to potential drugs: an old process or the new hope for drug discovery? J Med Chem. 2008; 51(9):2589–2599. PMID: 18393402.


13. Efange SMN. Natural products: a continuing source of inspiration for the medical chemist. In : Wootton JC, editor. Advances in phytomedicine. 5nd ed. Amsterdam: Elsevier Science;2002. p. 61–69.
14. Parasuraman S. Toxicological screening. J Pharmacol Pharmacother. 2011; 2(2):74–79. PMID: 21772764.


15. Bruneton J. Pharmacognosie, Phytochimie, Plantes Médicinales. Paris: ditions Technique & Documentation;1995. p. 1120.
16. Francis G, Kerem Z, Makkar HP, Becker K. The biological action of saponins in animal systems: a review. Br J Nutr. 2002; 88(6):587–605. PMID: 12493081.


17. Sparg SG, Light ME, van Staden J. Biological activities and distribution of plant saponins. J Ethnopharmacol. 2004; 94(2-3):219–243. PMID: 15325725.


18. Sahu NP, Banerjee S, Mondal NB, Mandal D. Steroidal saponins. Fortschritte der Chemie Organischer Naturstoffe/progress in the chemistry of organic natural products, fortschritte der chemie organischer naturstoffe/progress in the chemistry of organic natural products, vol 89. Vienna: Springer;2008. p. 45–141.
19. Podolak I, Galanty A, Sobolewska D. Saponins as cytotoxic agents: a review. Phytochem Rev. 2010; 9(3):425–474. PMID: 20835386.


20. Alaoui K, Belabbes M, Cherrah Y, Hassar M, Charrouf Z, Amarouch H, Roquebert J. Acute and chronic toxicity of saponins from Argania spinosa. Ann Pharm Fr. 1998; 56(5):213–219. PMID: 9805821.
21. Witthawaskul P, Panthong A, Kanjanapothi D, Taesothikul T, Lertprasertsuke N. Acute and subacute toxicities of the saponin mixture isolated from Schefflera leucantha Viguier. J Ethnopharmacol. 2003; 89(1):115–121. PMID: 14522442.
22. Wisløff H, Uhlig S, Scheie E, Loader J, Wilkins A, Flåøyen A. Toxicity testing of saponin-containing Yucca schidigera Roetzl. juice in relation to hepato- and nephrotoxicity of Narthecium ossifragum (L.) Huds. Toxicon. 2008; 51(1):140–150. PMID: 17942132.
23. Cho YM, Imai T, Ito Y, Takami S, Hasumura M, Yamazaki T, Hirose M, Nishikawa A. A 13-week subchronic toxicity study of dietary administered saponin-rich and isoflavones-containing soybean extract in F344 rats. Food Chem Toxicol. 2009; 47(8):2150–2156. PMID: 19501625.


24. Bissell DM, Gores GJ, Laskin DL, Hoofnagle JH. Drug-induced liver injury: mechanisms and test systems. Hepatology. 2001; 33(4):1009–1013. PMID: 11283870.


25. Kleiner DE, Brunt EM, Van Natta M, Behling C, Contos MJ, Cummings OW, Ferrell LD, Liu YC, Torbenson MS, Unalp-Arida A, Yeh M, McCullough AJ, Sanyal AJ. Design and validation of a histological scoring system for nonalcoholic fatty liver disease. Hepatology. 2005; 41(6):1313–1321. PMID: 15915461.


26. Giannini E, Botta F, Fasoli A, Ceppa P, Risso D, Lantieri PB, Celle G, Testa R. Progressive liver functional impairment is associated with an increase in AST/ALT ratio. Dig Dis Sci. 1999; 44(6):1249–1253. PMID: 10389705.
27. Sheth SG, Flamm SL, Gordon FD, Chopra S. AST/ALT ratio predicts cirrhosis in patients with chronic hepatitis C virus infection. Am J Gastroenterol. 1998; 93(1):44–48. PMID: 9448172.


28. Yoon SH, Park EJ, Oh KH, Chung YG, Kwon OJ. The effect of Lithospermi radix on benzo (a) pyrene-induced hepatotoxicity. J Korean Soc Food Sci Nutr. 1993; 22(2):144–148.
29. Hayes AW. Principles and methods of toxicology. New York: Raven Press;1982. p. 447–474.
30. Kim DH, Deung YK, Lee YM, Yoon YS, Kwon KR, Park DB, Park YK, Lee KJ. The liver protecting effect of pomegranate (Punica granatum) seed oil in mice treated with CCl4. Korean J Electron Microsc. 2006; 36(3):173–182.
31. Michael B, Yano B, Sellers RS, Perry R, Morton D, Roome N, Johnson JK, Schafer K, Pitsch S. Evaluation of organ weights for rodent and non-rodent toxicity studies: a review of regulatory guidelines and a survey of current practices. Toxicol Pathol. 2007; 35(5):742–750. PMID: 17849357.


32. Singhal PC, Sharma P, Sanwal V, Prasad A, Kapasi A, Ranjan R, Franki N, Reddy K, Gibbons N. Morphine modulates proliferation of kidney fibroblasts. Kidney Int. 1998; 53(2):350–357. PMID: 9461094.


33. Falconer IR, Hardy SJ, Humpage AR, Froscio SM, Tozer GJ, Hawkins PR. Hepatic and renal toxicity of the blue-green alga (cyanobacterium) Cylindrospermopsis raciborskii in male Swiss albino mice. Environ Toxicol. 1999; 14(1):143–150.
34. Horiguchi H, Oguma E, Kayama F, Sato M, Fukushima M. Dexamethasone prevents acute cadmium-induced hepatic injury but exacerbates kidney dysfunction in rabbits. Toxicol Appl Pharmacol. 2001; 174(3):225–234. PMID: 11485383.


35. Bonventre JV, Vaidya VS, Schmouder R, Feig P, Dieterle F. Next-generation biomarkers for detecting kidney toxicity. Nat Biotechnol. 2010; 28(5):436–440. PMID: 20458311.


36. Ezejiofor AN, Orish CN, Orisakwe OE. Effect of aqueous leaves extract of Costus afer Ker Gawl (Zingiberaceae) on the liver and kidney of male albino Wistar rat. Anc Sci Life. 2013; 33(1):4–9. PMID: 25161323.
37. Diwan FH, Abdel-Hassan IA, Mohammed ST. Effect of saponin on mortality and histopathological changes in mice. East Mediterr Health J. 2000; 6(2-3):345–351. PMID: 11556022.


Figure 1
Schematic procedure of sample preparation and antioxidant properties of SEAC. (A) SEAC was obtained from the roots of A. cochinchinensis using ethyl acetate solution under the conditions described in the Materials and methods. (B) Components of SEAC. Total saponins, flavonoids and polyphenols were analyzed in several doses of SEAC. (C) Free radical scavenging activity of SEAC. DPPH radical scavenging activity was assayed in a mixture containing 0.1 mM DPPH and a range of concentrations of SEAC (0–2,000 µg/mL). DPPH, 2,2-diphenyl-1-picrylhydrazyl radical; IC50, half maximum inhibitory concentration. Values are presented as the means±SD of three replicates.
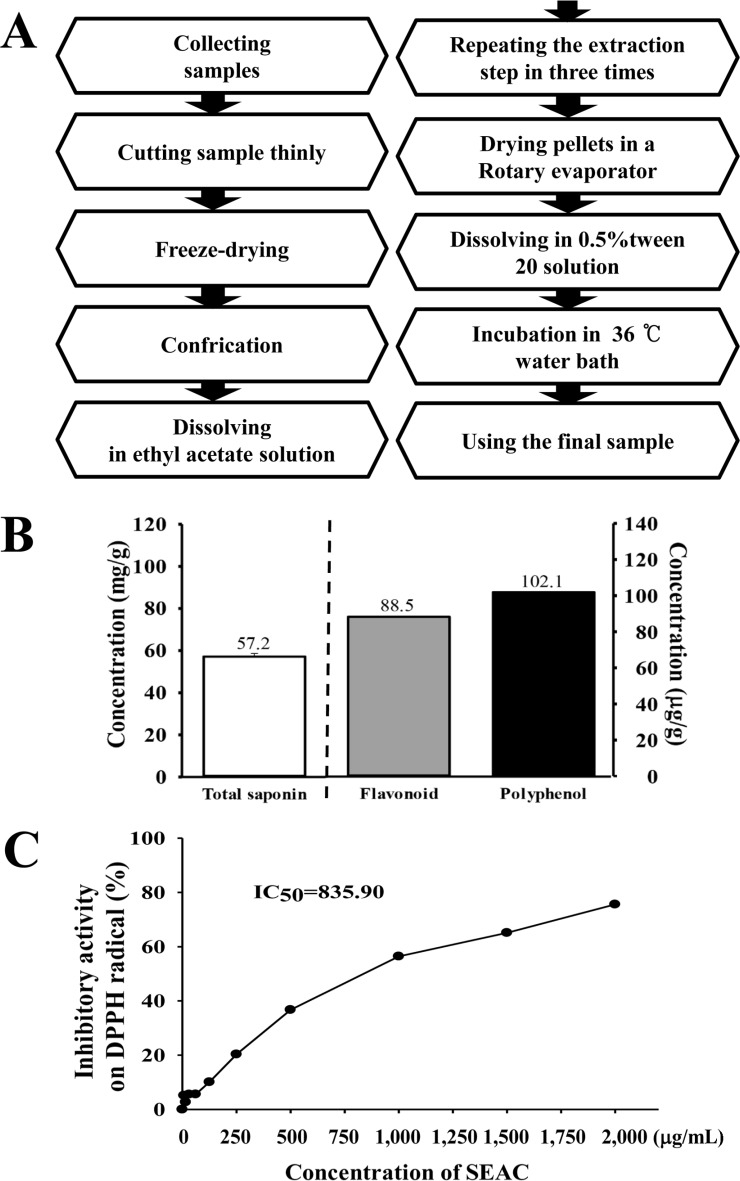
Figure 2
Effects of SEAC on liver toxicity in ICR mice. Liver tissues of male and female ICR mice were stained with H&E and histological structure was viewed at 400× magnification.
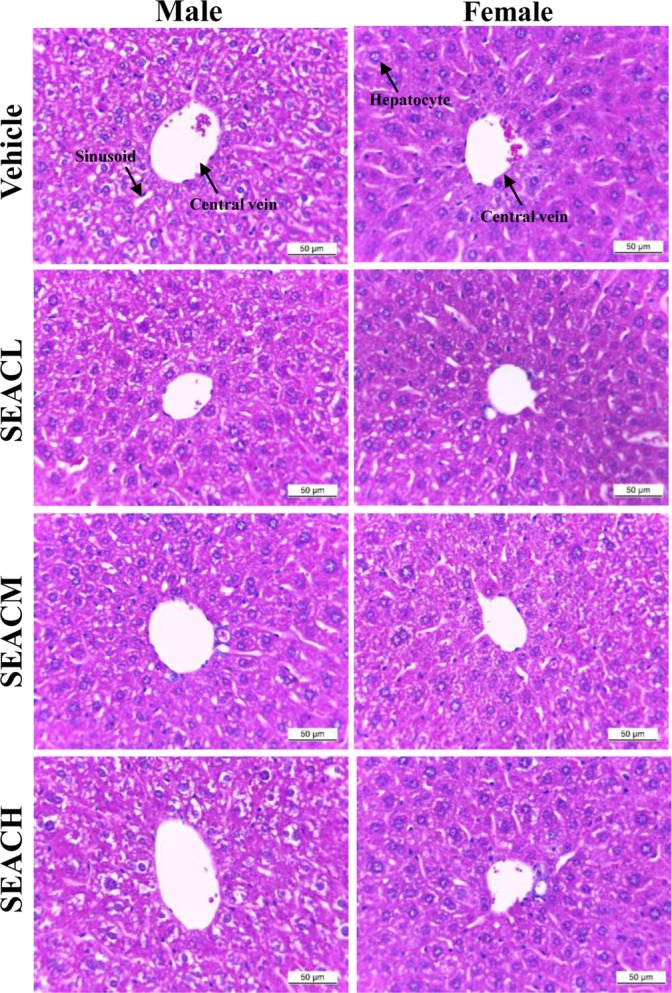
Figure 3
Effects of SEAC on the kidney toxicity in ICR mice. Kidney tissues of male (A) and female (B) ICR mice were stained with H&E, and histological structure was viewed at 400× magnification.
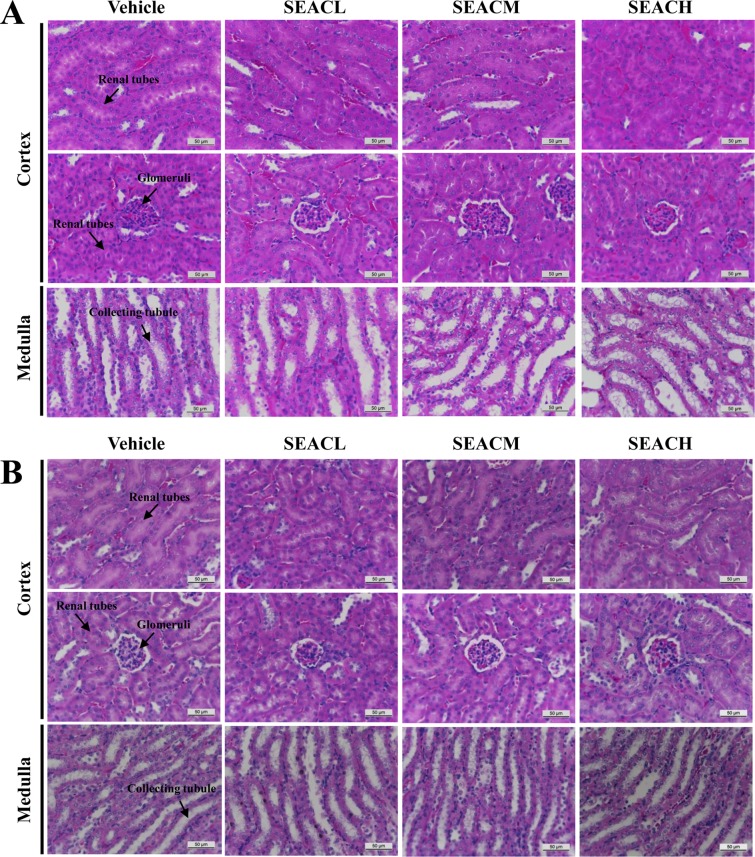
Table 1
Alteration of body and organ weights of female and male ICR mice treated with saponin-enriched extract of A. cochinchinensis (SEAC) for 14 days
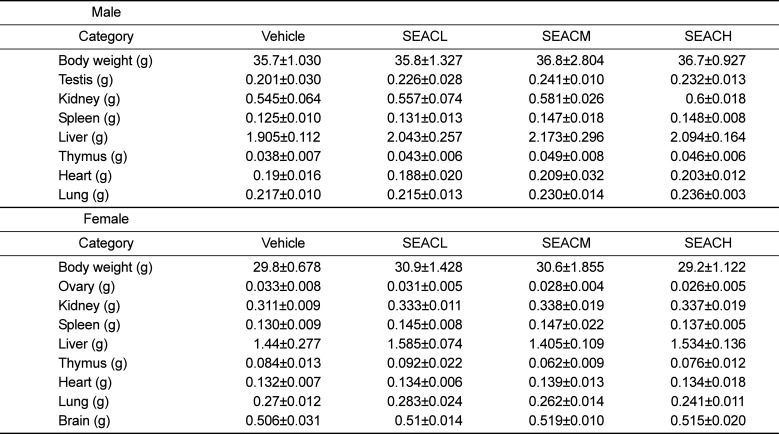
Table 2
Alteration on the urine parameters in male and female ICR mice after saponin-enriched extract of A. cochinchinensis (SEAC) treatment
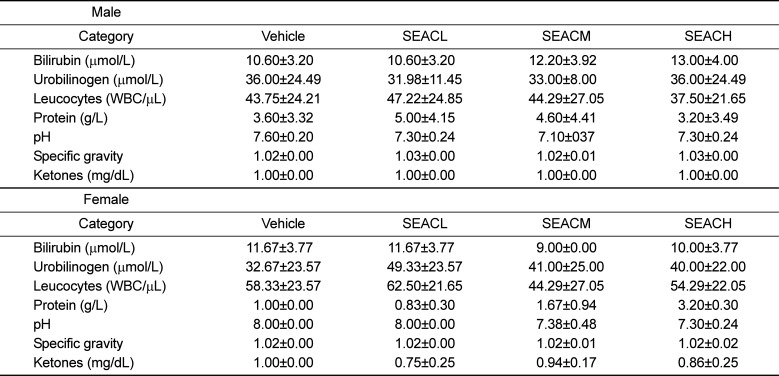
Table 3
Alteration on the serum parameters in male and female ICR mice after saponin-enriched extract of A. cochinchinensis (SEAC) treatment
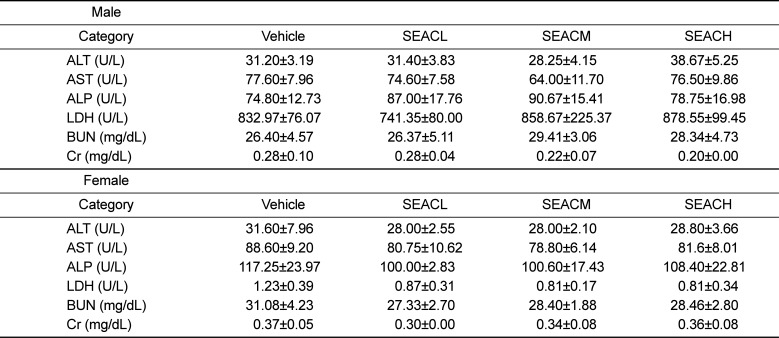