Abstract
The purpose of this study was to investigate the immunomodulatory activity of ice plant (Mesembryanthemum crystallinum) extract (IPE) in vitro and in vivo. Raji (a human B cell line) and Jurkat (a human T cell line) cells were treated with various doses of IPE and cell proliferation was measured by WST assay. Results showed that IPE promoted the proliferation of both Raji and Jurkat cells in a dose-dependent manner. IPE also enhanced IL-6 and TNF-α production in macrophages in the presence of lipopolysaccharide (LPS), although IPE alone did not induce cytokine production. Moreover, IPE treatment upregulated iNOS gene expression in macrophages in a time- and dose-dependent manner and led to the production of nitric oxide in macrophages in the presence of IFNγ. In vivo studies revealed that oral administration of IPE for 2 weeks increased the differentiation of CD4+, CD8+, and CD19+ cells in splenocytes. These findings suggested that IPE has immunomodulatory effects and could be developed as an immunomodulatory supplement.
The immune system is the body's defense against foreign antigens, and produces many cells and molecules that distinguish and eliminate foreign matter. The immune mediators released from macrophages play significant roles in these processes [12]. After cells have been infected with bacterial endotoxin lipopolysaccharide (LPS), macrophages are activated, which generate numerous inflammatory mediators such as interleukin (IL)-6, tumor necrosis factor (TNF)-α, and nitric oxide (NO) [3]. IL-6, a soluble mediator, which is promptly produced in response to infection and tissue injury, contributes to host defense through the stimulation of acute phase response, hematopoiesis, and immune reactions by the regulation of cell growth, proliferation, and differentiation [4]. NO is a major component in the innate immune reaction of the host to various pathogens including bacteria, viruses, and fungi. It is involved in the pathogenesis and control of infectious diseases, tumors, and autoimmune processes [5]. B and T lymphocytes are major cellular components of the adaptive immune response and generate specific responses to maximally eliminate specific pathogens. Because low immunity or an immunocompromised state can alter the host susceptibility to pathogens, and this phenomenon was related to disease [6], it can be expected that raising immune ability would be very important in the maintenance of health.
The modulation and activation of the immune response to alleviate disease has been of interest where plants may represent an ideal adjunct to existing chemotherapy for the treatment of various diseases. Hence, many plants that can activate the immune system have been suggested [78910]. For example, the extract of Gelidium amansii, a red algae cultivated on the northeastern coast of Taiwan, activated macrophages through an increase in cell proliferation and enhanced the production of NO, TNF-α, IL-1β and IL-6 [2].
Mesembryanthemum crystallinum is a halophyte that changes from C3 photosynthesis to Crassulacean acid metabolism (CAM) in response to high photon flux density, drought, and salinity [11]. The plant is covered with trichomes called epidermal bladder cells (EBC), which is reflected by its common names: crystalline ice plant or ice plant [12].
Ice plant is capable of growing under high salt conditions through the synthesis of protective osmolytes and antioxidant molecules such as beta cyanins and other flavonoids [13]. Ice plant has been reported to improve the proliferation of keratinocytes and lipolysis [1415]. In addition, ice plant has been found to contain a significant amount of D-pinitol, which has been reported to show potent anti-oxidant [16], anti-diabetic [17], anticancer [18], and anti-inflammatory activity [19]. However, little is known about the immunomodulatory effects of ice plant. The present study was therefore designed to investigate the potential effects of IPE on the immune-enhancing function in vitro, in several immune cell lines, and in vivo, in mice administered IPE for 2 weeks.
Ultrapure lipopolysaccharide (LPS) from Escherichia coli O111:B4 was purchased from InvivoGen (San Diego, CA).
A single seed of ice plant (Mesembryanthemum crystallinum) was planted in a plastic pot containing a sponge and germinated in a green house at 25℃ with an ordinary nutrient solution. One month after the seeds were germinated, plantlet samples were freeze-dried in a vacuum for a minimum of 72 h and ground into a fine powder using a mortar and pestle. One hundred grams of each powdered sample was extracted with 10 L of 70% ethanol for 4 h at 40℃ in a shaking incubator. After centrifugation to remove impurities, the ethanol of the supernatant was evaporated using a rotary vacuum evaporator and the concentrate was diluted with distilled water to a final volume of 1 L. After filtration through a 0.45-µm PVDF filter, the extract was ready for use in experiments.
Seven-week-old male C57BL/6 mice were purchased from Koatech (Pyeongtaek, Gyeonggi-do, Korea). The animals were maintained for 1 week on a commercial pellet diet and then randomly divided into four groups (n=4), which were orally administered IPE (25, 125, and 250 µL/mL) or vehicle once a day for 2 weeks. All animal studies were approved by the Institutional Animal Care and Use Committee of Chonnam National University (Approval No. CNU IACUC-YB-2016-53).
Raji and Jurkat cells were purchased from American Type Culture Collection (Manassas, VA, USA). Raji and Jurkat cells are suspensions cell and the first continuous human cell lines from B lymphocytes and T lymphocytes, respectively. Both cell types were maintained in RPMI-1640 medium supplemented with 10% FBS and 1% penicillin/streptomycin in a 5% CO2 incubator at 37℃. Bone marrow-derived macrophages (BMDMs) derived from murine bone marrow were prepared as previously described [20]. Briefly, BMDMs were cultured in complete Iscove's modified Dulbecco's medium (IMDM, Gibco, Grand Island, NY, USA) supplemented with 30% L929 cell culture supernatant, 10% FBS, 1% sodium pyruvate, 1% MEM Non-Essential Amino Acids (MEM NEAA), and 1% penicillin/streptomycin in a 5% CO2 incubator at 37℃. After 3 days, 10 mL of fresh medium was added, and the cells were incubated for an additional 2 days.
Cell proliferation was measured by the EZ-Cytox (Daeillab service, Seoul, Korea) assay kit based on water-soluble tetrazolium salt (WST). Briefly, Raji and Jurkat cells were seeded in tripliate in a 48-well plate at a density of 8×105 cells/well and incubated for 24 h. Subsequently, the cells were treated with different concentrations of IPE. After 24 h, the WST reagent solution (10 µL) was added to each well. The plate was incubated for 3 h in a CO2 incubator and measured by using an ELx808 microplate spectrophotometer at a wavelength of 450 nm.
To determine the production of IL-6 and TNFα, BMDMs were seeded in triplicate in a 48-well plate at a concentration of 8×105 cells/well and treated with LPS (10 ng/mL) in the absence or presence of IPE (2.5, 5, and 10 µL/mL) for 24 h. The concentrations of IL-6 and TNF-α in culture supernatants were determined by using a commercial enzyme-linked immunosorbent assay (ELISA) kit (R&D Systems, Minneapolis, MN, USA).
iNOS gene expression levels were determined by real-time qPCR. BMDMs were treated with IPE (1, 2.5, 5 µL/mL) for 6 h, RNA was extracted using the easy-BLUE™ Total RNA Extraction Kit (iNtRON Biotechnology, Seongnam, Korea), and cDNA was prepared from 0.1 µg of RNA using ReverTra Ace® qPCR RT Master Mix (TOYOBO Bio-Technology, Osaka, Japan) in accordance with the manufacturer's instructions. Real-time PCR was performed using the SYBR Green PCR Kit (Qiagen GmbH, Hilden, Germany). The primer sequences for RT-PCR amplification were as follows: iNOS; sense, 5′-GCATTGGAAGTGAAGCGTTTC-3′ and antisense, 5′-GGCAGCCTGTGAGACCTTTG-3′, GAPDH; sense, 5′-CGACTTCAACAGCAACTCCCA CTCTTCC-3′ and antisense, 5′-TGGGTGGTCCAGGG TTTCTTACTCCTT-3′. PCR was performed in a Rotor-Gene Q real-time PCR system (Qiagen) using a two-step protocol of 40 cycles of 95℃ for 10 s followed by 58℃ for 45 s. The results were normalized using GAPDH as an internal control.
BMDMs were plated at a density of 8×105 cells/mL in a 48-well plate and incubated with or without INF-γ (100 ng/mL) in the absence or presence of various concentrations of ice plant extract for 24 h. Nitrite accumulation in supernatants was assessed using the Griess reaction [21]. An aliquot of the culture supernatant (100 µL) was mixed with equal volumes of Griess reagent [0.1% (w/v) N-(1-naphthyl)-ethylenediamine, with 1% (w/v) sulfanilamide in 5% (v/v) phosphoric acid] and incubated at room temperature for 10 min. The absorbance was measured at 577 nm by using a microplate reader, and a series of known sodium nitrite concentrations were used to construct a standard curve of absorption.
Spleens were removed aseptically from mice and placed individually into petri dishes containing 3 mL of complete RPMI 1640 medium. To prepare single cell suspensions, the spleens were chopped into small pieces and the spleen tissue was forced up and down through a 3-mL syringe, as previously described [22]. The suspension was transferred to a 15-mL conical tube containing 3 mL of complete RPMI 1640 and centrifuged at 1700 rpm for 10 min. The cells were re-suspended in 0.87% Tris-NH4Cl and incubated for 10 min at room temperature to lyse the erythrocytes. After two washes in complete RPMI 1640, the suspensions were adjusted to a final concentration of 5×104 cells/wells in complete RPMI 1640.
Flow cytometry, which simultaneously measures multiple characteristics of single cells at a rapid rate, was used in our experiment to detect accessory molecule expression on spleen cells after fluorescence activated cell sorting (FACS). In brief, splenocytes were harvested and washed with 0.5% BSA. The cells were stained with fluorescently labeled monoclonal antibodies (mAbs) to the mouse antigens of interest at optimal concentrations for an additional 30 min at 4℃. Antibodies (BD Biosciences, San Jose, CA) were directly labeled with the following fluorescent tags: fluorescein isothiocyanate (FITC) or phycoerythrin-Cyanine 7 (PE-Cy™7) for CD4 [GK1.5 and Rat (LEW) IgG2b, κ], CD8 [53-6.7 and Rat(LOU) IgG2a, κ], and CD19 [1D3 and Rat (LEW) IgG2a, κ]. In the experiments, CD4- PE-Cy™7 was used as a specific marker for T-helper (Th) lymphocytes and NK-T lymphocytes, CD8-FITC was used as a specific marker for cytolytic T lymphocytes and dendritic cells, and CD19-FITC was used as a specific marker for B cells. Appropriate isotype controls were always included. After centrifugation, the cells were fixed with 1% formalin, and 10000 viable cells per treatment (determined from light scattering profiles) were analyzed by using a BD FACSCalibur™ flow cytometer and CellQuest software (BD Biosciences).
The differences among the mean values for the different groups were evaluated, and all values were expressed as the mean±standard deviation (SD). Statistical calculations were performed using GraphPad Prism version 5.01 software (Graph-Pad Software). Cytokine production was analyzed by one-way ANOVA followed by the Bonferroni post-hoc test for multi-group comparisons. Differences were considered significant when P values were less than 0.05.
We first determined whether IPE promoted the proliferation of B and T cells. Raji (B cells) and Jurkat (T cells) cells were stimulated with various doses of IPE for 24 h and cell proliferation was determined by WST assay. IPE significantly promoted proliferation of Raji cells in a dose-dependent manner (Figure 1A). Moreover, the proliferation of Jurkat cells was significantly enhanced at all concentrations applied (Figure 1B).
We further sought to determine whether IPE induced cytokines production in macrophages. Bone marrow-derived macrophages (BMDMs) were stimulated with various doses of IPE in the range 0.1-50 µL/mL and levels of IL-6 and TNF-α in culture supernatants were determined by ELISA. IPE alone was unable to induce the proliferation of BMDMs and production of IL-6 and TNF-α (data not shown). However, IPE enhanced the LPS-induced production of IL-6 and TNF-α in BMDMs in a dose-dependent manner (Figure 2A, 2B). These results suggested that IPE may be able to cooperate with other immunostimulatory compounds to produce type I cytokines in macrophages.
Nitric oxide (NO) is an antibacterial and antiviral effector of the innate immune system, and IFN-γ-induced inducible NO synthase (iNOS) is involved in the synthesis of NO from arginine. Therefore, we examined the effect of IPE on iNOS gene expression and NO production in BMDMs. IPE (5 µL/mL) induced optimal expression of iNOS mRNA at 6 h after treatment (Figure 3A). To determine dose-responsiveness, BMDMs were treated with the indicated doses of IPE for 6 h. iNOS mRNA expression was upregulated by IPE in a dose-dependent manner (Figure 3B). IPE-induced production of NO in the culture supernatant was determined by the Griess reaction. IPE alone did not produce a detectable level of NO in BMDMs (data not shown), although it did upregulate gene expression of iNOS. However, in the presence of IFN-γ, IPE induced NO production in BMDMs in a dose-dependent manner (Figure 3C).
To investigate the effect of IPE on in vivo immunomodulation, mice were orally administered IPE (200 mL) at doses of 25, 125, and 250 µL/mL for 2 weeks. The effect of IPE on splenocyte population in mice was measured by using flow cytometry to assess the expression of cell surface markers such as CD4+, CD8+, and CD19+. The percentage of CD4+ cells in the spleens was increased in response to IPE at doses of 25 and 125 µL/mL, but not at 250 µL/mL (Figure 4A). The population of CD8+ in the spleens was also increased in all groups administered with IPE compared with the vehicle group (Figure 4B). Differentiation into CD19+ cells was also increased in groups treated with IPE at 25 and 125 µL/mL, but was returned to original levels in the mice administered IPE at 250 µL/mL (Figure 4C).
This study provided evidence of the in vivo immunomodulatory activities of IPE, which increased cell proliferation and the production of immune mediators. A variety of foods or plants have been reported to possess immune-enhancing activities through the activation of macrophages because the increased production of NO and cytokines such as IL-6 and TNF-α by the activated macrophages helps to clear pathogens [23]. IL-6 and TNF-α are pro-inflammatory cytokines and known to contribute to the inflammatory response and the development of various inflammatory diseases [2425]. TNF-α is essential in the containment of intracellular pathogens. It stimulates the recruitment of inflammatory cells to the area of infection and activates macrophages, which then phagocytose and/or kill mycobacteria and other pathogens [26]. In vitro studies have suggested several roles for TNF-α in the regulation of B and T cell proliferation and differentiation [2728]. IL-6 plays a protective role in the immune response against bacterial infection. IL-6 acts as a growth factor for mitogen-activated human B cells, stimulates their final maturation into antibody-producing plasma cells, and is involved in T cell activation and differentiation [2930]. In our study, IPE increased lymphocyte proliferation and enhanced the production of immune mediators, while the extract had no effect on macrophage proliferation. NO is a free radical synthesized from the amino acid L-arginine by the enzymatic activity of iNOS. The biological effects of NO have been identified as the regulation of vascular relaxation, platelet aggregation, and cellular respiration. In addition, it is known that NO can directly and indirectly modulate the immune response through the cytotoxic functions of macrophages against a variety of tumors and micro-organisms [31]. iNOS is absent from resting cells, and is expressed in response to pro-inflammatory cytokines such as IFN-γ, TNF-α, and LPS or microbial compounds [5]. Pyo et al. [32] reported that the immunomodulatory effects of Cimicifugae rhizoma extract comprise NO production and iNOS mRNA expression in macrophages. Therefore, the tumoricidal activity induced by C. rhizoma extract appeared to be mediated by the production of NO. Our study showed that IPE significantly upregulated IFN-γ-induced NO production and iNOS mRNA expression.
The cluster of differentiation (CD) is a protocol used for the identification and investigation of the cell surface molecules present on leukocytes. The two most commonly used CD molecules are CD4 and CD8, which are markers for T helper cells and cytotoxic T cells, respectively. T lymphocytes play a critical role in the development of the acquired immune response [33]. CD19, a human protein encoded by the CD19 gene, is critically involved in the establishment of intrinsic B cell signaling thresholds for antigen receptor-dependent stimulation [34]. Many plants extracts have been reported to exert immunostimulatory activity on different immune cells. For example, Han et al. showed that water extracts from various mushrooms promoted the population of CD3, CD19, CD4, and CD8 positive cells in the spleen [35]. To examine the effect of IPE on the population of T and B lymphocytes in the spleen, flow cytometric analysis was performed. Our data indicated that IPE increased the percentage of CD4, CD8, and CD19 positive cells populations in the groups administered 25 and 125 µL/mL, to a lesser extent, in the group administered 250 µL/mL. Overall, our findings indicated that IPE enhanced immune activities in vitro and in vivo.
In conclusion, the current study demonstrated that IPE was capable of efficiently enhancing immune functions. Overall, we confirmed that IPE possessed immunomodulatory functions, in which IPE may initiate innate immunity by an increase in macrophage proliferation, cytokine secretion, iNOS expression, and lymphocyte proliferation. Moreover, we suggested that IPE application, especially at concentrations of 25 and 125 µL/mL, increased the numbers of T and B cells in mice spleen. Our results support the idea that IPE has potential as an adjunctive immune-enhancing or immunomodulatory agent.
Acknowledgment
This study was supported by the Small and Medium Business Administration (SMBA, Grant No. S2357445).
References
1. Yan ZQ, Hansson GK. Innate immunity, macrophage activation, and atherosclerosis. Immunol Rev. 2007; 219:187–203. PMID: 17850490.


2. Wang ML, et al. Immunomodulatory activities of Gelidium amansii gel extracts on murine RAW 264.7 macrophages. J Food Drug Anal. 2013; 21(4):397–403.


3. Zhang X, Morrison DC. Lipopolysaccharide-induced selective priming effects on tumor necrosis factor alpha and nitric oxide production in mouse peritoneal macrophages. J Exp Med. 1993; 177(2):511–516. PMID: 8426119.


4. Naka T, Nishimoto N, Kishimoto T. The paradigm of IL-6: from basic science to medicine. Arthritis Res. 2002; 4(Suppl 3):S233–S242. PMID: 12110143.
5. Bogdan C. Nitric oxide and the immune response. Nat Immunol. 2001; 2(10):907–916. PMID: 11577346.


6. Prasad S, Sung B, Aggarwal BB. Age-associated chronic diseases require age-old medicine: role of chronic inflammation. Prev Med. 2012; 54(Suppl):S29–S37. PMID: 22178471.


7. Miyazaki Y, Kusano S, Doi H, Aki O. Effects on immune response of antidiabetic ingredients from white-skinned sweet potato (Ipomoea batatas L.). Nutrition. 2005; 21(3):358–362. PMID: 15797679.


8. Lin BF, Chiang BL, Lin JY. Amaranthus spinosus water extract directly stimulates proliferation of B lymphocytes in vitro. Int Immunopharmacol. 2005; 5(4):711–722. PMID: 15710340.
9. Chen X, Nie W, Yu G, Li Y, Hu Y, Lu J, Jin L. Antitumor and immunomodulatory activity of polysaccharides from Sargassum fusiforme. Food Chem Toxicol. 2012; 50(3-4):695–700. PMID: 22120506.


10. Thakur M, Connellan P, Deseo MA, Morris C, Praznik W, Loeppert R, Dixit VK. Characterization and in vitro immunomodulatory screening of fructo-oligosaccharides of Asparagus racemosus Willd. Int J Biol Macromol. 2012; 50(1):77–81. PMID: 22001723.
11. Tsukagoshi H, Suzuki T, Nishikawa K, Agarie S, Ishiguro S, Higashiyama T. RNA-seq analysis of the response of the halophyte, Mesembryanthemum crystallinum (ice plant) to high salinity. PLoS One. 2015; 10(2):e0118339. PMID: 25706745.


12. AMARASINGHE V, WATSON L. Comparative ultrastructure of microhairs in grasses. Bot J Linn Soc. 1988; 98(4):303–319.


13. Vogt T, Ibdah M, Schmidt J, Wray V, Nimtz M, Strack D. Light-induced betacyanin and flavonol accumulation in bladder cells of Mesembryanthemum crystallinum. Phytochemistry. 1999; 52(4):583–592. PMID: 10570827.


14. Deters AM, Meyer U, Stintzing FC. Time-dependent bioactivity of preparations from cactus pear (Opuntia ficus indica) and ice plant (Mesembryanthemum crystallinum) on human skin fibroblasts and keratinocytes. J Ethnopharmacol. 2012; 142(2):438–444. PMID: 22713931.


15. Drira R, Matsumoto T, Agawa M, Sakamoto K. Ice Plant (Mesembryanthemum crystallinum) Extract Promotes Lipolysis in Mouse 3T3-L1 Adipocytes Through Extracellular Signal-Regulated Kinase Activation. J Med Food. 2016; 19(3):274–280. PMID: 26390196.


16. Sivakumar S, Subramanian SP. Pancreatic tissue protective nature of D-Pinitol studied in streptozotocin-mediated oxidative stress in experimental diabetic rats. Eur J Pharmacol. 2009; 622(1-3):65–70. PMID: 19765586.


17. Lee BH, Lee CC, Wu SC. Ice plant (Mesembryanthemum crystallinum) improves hyperglycaemia and memory impairments in a Wistar rat model of streptozotocin-induced diabetes. J Sci Food Agric. 2014; 94(11):2266–2273. PMID: 24374864.


18. Lin TH, Tan TW, Tsai TH, Chen CC, Hsieh TF, Lee SS, Liu HH, Chen WC, Tang CH. D-pinitol inhibits prostate cancer metastasis through inhibition of αVβ3 integrin by modulating FAK, c-Src and NF-κB pathways. Int J Mol Sci. 2013; 14(5):9790–9802. PMID: 23698767.
19. Singh RK, Pandey BL, Tripathi M, Pandey VB. Anti-inflammatory effect of (+)-pinitol. Fitoterapia. 2001; 72(2):168–170. PMID: 11223227.


20. Celada A, Gray PW, Rinderknecht E, Schreiber RD. Evidence for a gamma-interferon receptor that regulates macrophage tumoricidal activity. J Exp Med. 1984; 160(1):55–74. PMID: 6330272.


21. Kim H, Lee HS, Chang KT, Ko TH, Baek KJ, Kwon NS. Chloromethyl ketones block induction of nitric oxide synthase in murine macrophages by preventing activation of nuclear factor-kappa B. J Immunol. 1995; 154(9):4741–4748. PMID: 7536778.
22. Gill HS, Rutherfurd KJ, Prasad J, Gopal PK. Enhancement of natural and acquired immunity by Lactobacillus rhamnosus (HN001), Lactobacillus acidophilus (HN017) and Bifidobacterium lactis (HN019). Br J Nutr. 2000; 83(2):167–176. PMID: 10743496.
23. Ghonime M, Emara M, Shawky R, Soliman H, El-Domany R, Abdelaziz A. Immunomodulation of RAW 264.7 murine macrophage functions and antioxidant activities of 11 plant extracts. Immunol Invest. 2015; 44(3):237–252. PMID: 25564700.


24. Glauser MP. The inflammatory cytokines. New developments in the pathophysiology and treatment of septic shock. Drugs. 1996; 52(Suppl 2):9–17.
25. Hirohashi N, Morrison DC. Low-dose lipopolysaccharide (LPS) pretreatment of mouse macrophages modulates LPS-dependent interleukin-6 production in vitro. Infect Immun. 1996; 64(3):1011–1015. PMID: 8641750.
26. Winthrop KL. Risk and prevention of tuberculosis and other serious opportunistic infections associated with the inhibition of tumor necrosis factor. Nat Clin Pract Rheumatol. 2006; 2(11):602–610. PMID: 17075599.


27. Boyman O, Hefti HP, Conrad C, Nickoloff BJ, Suter M, Nestle FO. Spontaneous development of psoriasis in a new animal model shows an essential role for resident T cells and tumor necrosis factor-alpha. J Exp Med. 2004; 199(5):731–736. PMID: 14981113.
28. Kehrl JH, Miller A, Fauci AS. Effect of tumor necrosis factor alpha on mitogen-activated human B cells. J Exp Med. 1987; 166(3):786–791. PMID: 3040886.


29. Bettelli E, Carrier Y, Gao W, Korn T, Strom TB, Oukka M, Weiner HL, Kuchroo VK. Reciprocal developmental pathways for the generation of pathogenic effector TH17 and regulatory T cells. Nature. 2006; 441(7090):235–238. PMID: 16648838.


30. Kishimoto T, Akira S, Narazaki M, Taga T. Interleukin-6 family of cytokines and gp130. Blood. 1995; 86(4):1243–1254. PMID: 7632928.


31. MacMicking J, Xie QW, Nathan C. Nitric oxide and macrophage function. Annu Rev Immunol. 1997; 15:323–350. PMID: 9143691.


32. Suhkneung P, et al. Immunomodulatory Effects of Cimicifugae Rhizoma Extracts in Macrophages. Prev Nutr Food Sci. 2006; 11(4):268–272.
33. Swain SL, McKinstry KK, Strutt TM. Expanding roles for CD4 T cells in immunity to viruses. Nat Rev Immunol. 2012; 12(2):136–148. PMID: 22266691.


34. Fujimoto M, Poe JC, Inaoki M, Tedder TF. CD19 regulates B lymphocyte responses to transmembrane signals. Semin Immunol. 1998; 10(4):267–277. PMID: 9695183.


35. Han SS, Cho CK, Lee YW, Yoo HS. Antimetastatic and immunomodulating effect of water extracts from various mushrooms. J Acupunct Meridian Stud. 2009; 2(3):218–227. PMID: 20633495.


Figure 1
Proliferative effect of ice plant extract treatment on B and T cells. Different concentrations of IPE treatment were applied to Raji (A) or Jurkat cells (B) for 24 h. Cell proliferation was determined as described in Materials and Methods. Data are presented as mean±SD (n=3). **P<0.01 vs control, ***P<0.001 vs control.
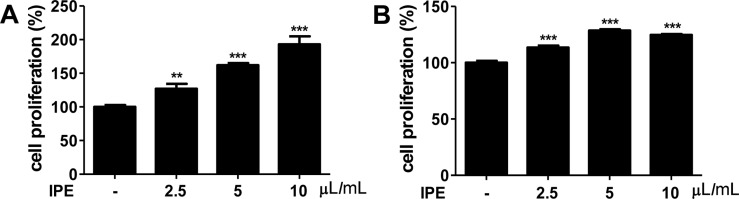
Figure 2
Effect of ice plant extract on proliferation and cytokine production in macrophages. Cells were treated with LPS (10 ng/mL) for 24 h in the absence or presence of the indicated dose of IPE. IL-6 (A) and TNF-α (B) levels in culture supernatants were measured by ELISA. Data are presented as mean±SD (n=3). ***P<0.001 vs LPS-treated group.
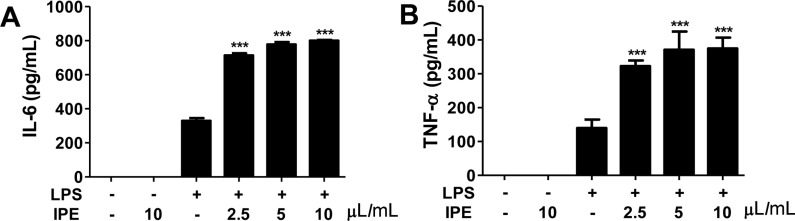
Figure 3
Effect of ice plant extract on the expression of iNOS mRNA in IFN-γ-stimulated macrophages. (A) Cells were treated with IPE (5 µL/mL) and co-treated with IFN-γ (100 ng/mL) for the indicated time. (B) Cells were treated with the indicated concentration of IPE and co-treated with IFN-γ (100 ng/mL) for 6 h. iNOS gene expression was analyzed using real-time qPCR and results were normalized to the expression of GAPDH. (C) Cells were treated with various concentrations of IPE for 24 h. The nitrite content in the medium was determined by Griess reagent. Data are shown as the mean±SD of triplicate samples from one representative experiment of three independent experiments. **P< 0.01 vs control, ***P< 0.001 vs control.
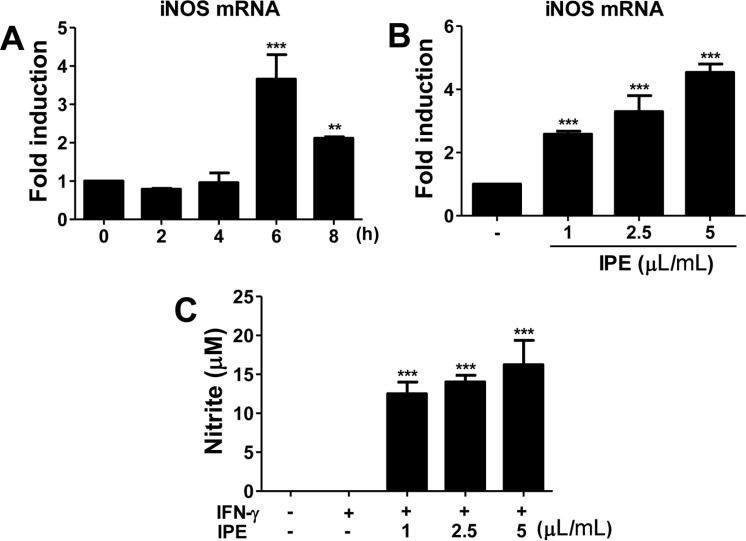
Figure 4
Population changes in lymphocyte subsets in mice administered ice plant extract for 2 weeks. On the day after the final administration, mice were sacrificed and their spleens were collected. Splenic single cells (1×105 cells/well) were stained with FITC- and PE-conjugated monoclonal antibodies specific for the mouse T cell markers CD4 (A), CD8 (B), and the mouse B cell marker CD19 (C). Stained cells were analyzed by flow cytometry using a BD Accuri C6. Values are reported as the percentage of each lymphocyte subset population expressing the CD marker.
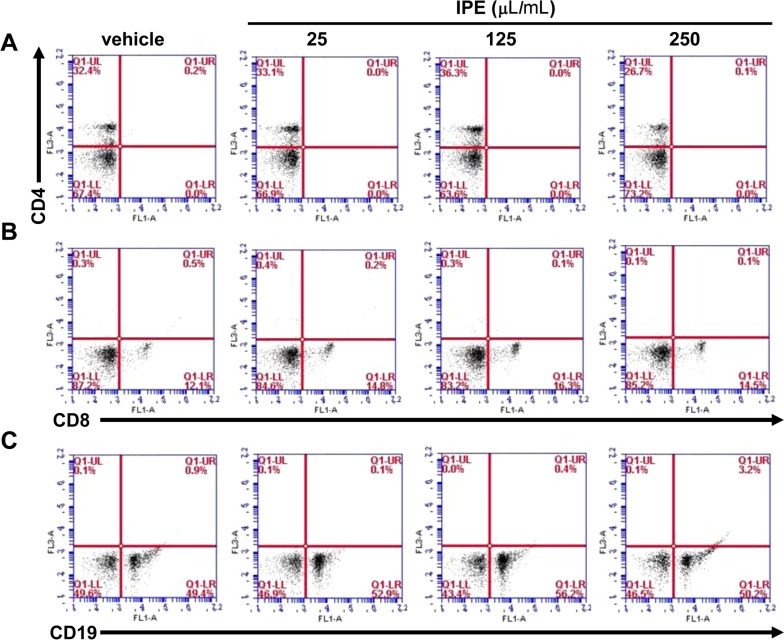