Abstract
Mulberry (Morus alba) leaves are known to have therapeutic effects on lipid metabolism including lipogenesis, lipolysis and hyperlipidemia. However, novel compounds with strong lipolytic ability among 27 extracts of the mulberry leaves fermented with Cordyceps militaris (EMfCs) have not yet been identified. Therefore, the cAMP concentration and cell viability were measured in the primary adipocytes of SD (Sprague Dawley) rats and 3T3-L1 cells after treatment of 27 EMfCs. Briefly, mulberry leaves powders amended with three different concentrations (0, 25 and 50%) of silkworm pupae (SWP) powder were fermented with 10% C. militaris (v/w) during three different periods (3, 4 and 6 weeks). A total of 27 extracts were obtained from the fermented mulberry leaves powders using three different solvents (dH2O, 50% EtOH and 95% EtOH). Among the 27 EMfCs treated groups, a significant increase in the concentration of cAMP was detected in primary adipocytes treated with 10 extracts when compared with the Vehicle treated group. However, their cAMP concentration did not agree completely with the non-toxicity, although most extracts showed non-toxicity. Furthermore, the concentration of cAMP and level of free glycerol gradually increased in a dose dependent manner (100, 200 and 400 µg/mL) of 4M3-95 contained cordycepin without any significant toxicity. Overall, the results of this study provide strong evidence that 4M3-95 extract derived from EMfCs can stimulate the lipolysis of primary adipocytes at an appropriate concentration and therefore have the potential for use as lipolytic agents to treat obesity.
Obesity is serious health problems caused by a positive mismatch between food intake and energy expenditure, as well as the pathological accumulation of triglycerides (TG) in adipose and other tissues [1]. An imbalance between the hydrolysis (lipolysis) and synthesis (lipogenesis) of TG in adipose tissue plays a key role during the initiation and pathogenesis of obesity [2]. In lipolysis, TG is hydrolyzed into fatty acid and glycerol at in lipid droplets of adipose tissue. This process can be controlled by various regulators including some hormones, neurotransmitters and other factors [3]. The cAMP-dependent protein kinase A (PKA) pathway, which is the best-characterized lipolysis mechanism, is involved in the phosphorylation and translocation of hormone sensitive lipase (HSL) [45]. Furthermore, many lipolysis stimulators including novel bioactive compounds and natural extracts have been investigated in adipocytes and animal models [167].
Several parts of mulberry (Morus alba), including the leaves, bark and branches, have long been used in traditional Chinese medicine for treatment of various chronic diseases [8]. Among these, mulberry leaves are widely used to treat some metabolic diseases including diabetes [9], hypoglycemia [1011], hypertension [12], dyslipidemia [13] and fatty liver disease [14] because they contain high concentrations of polyphenolics (rutin, quercetin and 1-deoxynojirimycin) [15] and the flavonol glycoside (quercetin 3-(6''-malonyl)-glucoside) [16]. Several studies have revealed the beneficial effects of mulberry leaves on lipid metabolism. Administration of mulberry leaves to Wistar/ST rats for 7 weeks induced the upregulation of fatty acid oxidation and down-regulation of lipogenesis related genes [17]. Moreover, ethanol extracts of mulberry leaves significantly reduced fat accumulation and protein expression of adipogenesis-related factors in 3T3-L1 adipocytes [18], while inducing decreases in LXRα-mediated lipogenesis markers and the upregulation of lipolysis-associated markers in high fat diet-fed mice [19]. Furthermore, mulberry leaves stimulated the proliferation and differentiation of 3T3-L1 adipocytes and ameliorated the expression of adipocytokines by suppressing oxidative stress in white adipose tissue of db/db mice [2021]. However, no studies have investigated novel extracts of mulberry leaves fermented with Cordyceps militaris under various conditions for lipolytic activity.
In this study, we investigated the lipolytic activity of 27 EMfCs in the primary adipocytes from SD rats by measuring the concentration of cAMP and free glycerol release. The results provide the first scientific evidence that novel extract of mulberry leaves fermented with C. militaris can stimulate the lipolysis in the primary adipocytes of SD rats.
Leaf samples of mulberry (M. alba) were collected from plantations in the Sangju area of Korea in October 2015, then identified by one of the authors (Professor Young Whan Choi). Voucher specimens (accession number Mul-PDRL-1) were deposited in the herbarium of Pusan National University (Miryang, Korea). C. militaris was kindly provided by Professor Sang Mong Lee in the Department of Life Science and Environmental Biochemistry, Pusan National University and silkworm pupae powder was supplied by Jeongeup Agriculture Cooperative Federations for Silkworm Farming (Jeongeup, Korea). Briefly, the mulberry leaves were dried in a hot-air drying machine (JSR, Seoul, Korea) for 24 h at 60℃, then reduced to powder using an electric blender. Following sterilization at 121℃ for 60 min using an autoclave, the mulberry powder was mixed with silkworm pupae (SWP) powder at four different concentrations (0%-M1, 25%-M2 and 50%-M3) and subsequently inoculated with 10% C. militaris (v/w). The samples were then fermented in a shaking incubator at 25℃ for three different periods (3 weeks; 3M1, 3M2 and 3M3, 4 weeks; 4M1, 4M2 and 4M3, or 6 weeks: 6M1, 6M2 and 6M3). On the final day of fermentation, the mixtures were harvested from the flask and extracted using dH2O(W), 50% EtOH(50) or 95% EtOH(95) (Table 1). To prepare each extract of fermented mulberry, these powders were mixed with each solvent in a fixed liquor ratio (mulberry powder/solvent ratio, 1:10), then sonicated for 1 h using JAC ultrasonic equipment (KODO, Hwangseong, Korea). The supernatant was collected from this sonicated extract after centrifugation at 3,000 rpm for 10 min. Next, 9 mL of the same solvent was also added into the pellet and then sonicated under the same conditions. Following collection of the supernatant, the above procedure was repeated, after which the samples were filtered through a 0.4 µm filter and concentrated by vacuum evaporation. Finally, 27 EMfCs were lyophilized using circulating extraction equipment (IKA Labortechnik, Staufen, Germany) (Figure 1). Next, these extracts were dissolved in dH2O or DMSO to 40 mg/mL.
The animal protocol used in this study was reviewed and approved by the Pusan National University-Institutional Animal Care and Use Committee (PNU-IACUC; Approval Number PNU-2017-1461). All SD rats were handled in the Pusan National University-Laboratory Animal Resources Center, which is accredited by the Korea Food and Drug Administration (FDA) (Accredited Unit Number-000231) and AAALAC International (Accredited Unit Number; 001525). Eight-week-old male SD rats were purchased from Samtako Bio-Korea Inc. (Osan, Korea) and provided with ad libitum access to water and a standard irradiated chow diet (Samtako Inc.). During the experiment, rats were maintained in a specific pathogen-free state under a strict light cycle (lights on at 08:00 h and off at 20:00 h) at 23±2℃ and 50±10% relative humidity.
Briefly, the intra-abdominal adipose tissues were dissected from eight-week-old male SD rats after sacrifice using CO2 gas. These tissues (30 g) were then minced in 5 mL of DMEM supplemented with 1 mg/mL type I collagenase (Worthington Biochemical Co., Freehold, NJ, USA) and 1% BSA (MP Biomedicals, Illkirch, France) and subsequently incubated at 37℃ for 30 min in a shaking incubator (JSR, Gongju-City, Korea). The homogenate of adipose tissue was filtered through a 100 µm nylon mesh and washed three times in KRH (Krebs ringer/HEPES solution: 25 mM NaHCO3, 125 mM NaCl, 5 mM glucose, 2.5 mM KCl, 1.25 mM NaH2PO4, 2 mM CaCl2, 1 mM MgCl2, 25 mM HEPES) containing 1% BSA. Finally, the pellets of adipocytes harvested with centrifugation were diluted in KRH containing 3% BSA to generate a cell suspension. Primary adipocytes were grown in a humidified incubator at 37℃ under 5% CO2 and 95% air in KRH containing 3% BSA. Thereafter, these adipocytes were seeded onto 24-well plates for each experimental purpose and incubated with different concentration of EMfCs to measure the release of free glycerol.
The concentration of cAMP in the culture supernatant of adipocytes was determined using a cAMP ELISA kit (STA-500, Cell Biolabs Inc., Ltd., Wuhan, China) according to the manufacturer's protocol. Following treatment with Vehicle (dH2O or DMSO), or 10 µM of isoproterenol hydrochloride (Sigma-Aldrich Co., St. Louis, MO, USA), or 100, 200 and 400 µg/mL of EMfCs on adipocytes (4×105 cells/mL) for 24 h, cell culture medium samples were collected by centrifugation at 1,000 rpm for 3 min at 4℃, following which the supernatant was collected for analysis. An acetylation reagent, peroxidase cAMP tracer conjugation and anti-cAMP polyclonal antibody were added and samples were incubated at room temperature for 2 h, followed by the addition of substrate solution for 5 min at room temperature. The reaction was terminated by the addition of stop solution and the plates were read at an absorbance of 450 nm using a VersaMax Plate reader (Molecular Devices, LLC, Sunnyvale, CA, USA).
Free glycerol release was measured using free glycerol reagent (Sigma-Aldrich Co., St. Louis, MO, USA) according to the manufacturer's protocols [1]. To measure the glycerol level, adipocytes were seeded at a density of 2×105 cells/mL in KRH and grown at 37℃ incubator. After 24 h, they were either untreated (No group), treated with Vehicle (dH2O or DMSO), or pretreated with 10 µM of isoproterenol hydrochloride (Sigma-Aldrich Co.), or 100, 200 and 400 µg/mL of EMfCs. Following incubation for 24 h, the culture medium was collected from primary adipocytes treated with EMfCs and heated at 65℃ for 15 min to inactivate any enzymes released by the adipocytes. The inactivated medium (10 µL) was then mixed with 200 µL of glycerol detection reagent, after which the absorbance was read at 570 nm directly in the wells using a VERSA max plate reader (Molecular Devices, Sunnyvale, CA, USA).
Cell viability was determined using the tetrazolium compound 3-[4,5-dimethylthiazol-2-yl]-2,5-diphenyl-tetrazolium bromide (MTT) (Sigma-Aldrich Co.). To determine the cell viability, 3T3-L1 cells were seeded at a density of 1×104 cells/0.2 mL and then grown for 24 h in a 37℃ incubator. When the cells attained 70-80% confluence, they were treated with Vehicle (dH2O or DMSO), 100, 200, or 400 µg/mL of 27 EMfCs. Following incubation for 24 h, the supernatants of the 3T3-L1 cells were discarded, after which 0.2 mL of fresh DMEM media and 50 µL of MTT solution (2 mg/mL in PBS) were added to each well. The cells were then incubated at 37℃ for 4 h, after which the formazan precipitate was dissolved in DMSO and the absorbance at 570 nm was read directly in the wells using a VERSA max Plate reader (Molecular Devices).
The one-dimensional HPLC system (Agilent 1100; Agilent Technologies, Inc., Santa Clara, CA, USA) consisted of a quaternary pump, an auto-sampler, a degasser, an automatic thermostatic column compartment and a diode array detector. Chromatographic conditions for AD, HPC and UD analysis were as follows: A Phenomenex Luna C18 column (150×4.6 mm internal diameter; 5 mm particle size; Phenomenex, Torrance, CA, USA) was used; gradient elution was performed with (A) 0.025% formic acid in water and (B) acetonitrile (0-10 min, 0-5% B; 10-20 min, 5% B; 20-30 min, 5-15% B; 30-40 min, 15% B; 40-50 min, 15-100% B; 50-55 min, 100% B; and 55-60 min, 100-0% B); the flow rate was 0.5 mL/min; and the column temperature was 30℃. For DD and 5-HMF analysis, gradient elution was performed with (A) deionized water and (B) acetonitrile (0-25 min, 30-90% B; and 25-40 min, 90% B). A flow rate of 1.0 mL/min was used. The flow rate and pressure were maintained at 1.53mL/min and 35±2 psi, respectively. The wavelength was set at 254 nm and the output signal of the detector was recorded using Clarity™ Chromatography Software version 6.0 (DataApex, Prague, Czech Republic).
One-way ANOVA was used to identify significant differences between No and other treated groups (SPSS for Windows, Release 10.10, Standard Version, Chicago, IL, USA). Differences in the responses of the Vehicle and EMfCs treated groups, or those treated with 100 µg/mL of EMfCs and other concentrations of EMfCs were evaluated using a post-hoc test (SPSS for Windows, Release 10.10, Standard Version). All values are reported as the mean±standard deviation (SD) and a P value of <0.05 was considered significant.
To identify novel extracts with lipolytic activity among 27 EMfCs, the cAMP levels were analyzed in the culture medium of primary adipocytes derived from SD rats following treatment with 27 EMfCs. Increases in the cAMP level were detected in most groups treated with extracts purified using 4 weeks fermentation product, regardless of the SWP powder concentration, with significant increases observed in primary adipocytes treated with 5 extracts (4M1-W, 4M1-50, 4M1-95, 4M2-W, 4M2-50 and 4M3-95) compared with the Vehicle treated group (Figure 2). Also, several groups treated with extracts purified using 50% EtOH (3M1-50, 3M2-50, 3M3-50 and 6M3-50) showed a significant enhanced level of cAMP (Figure 2). Therefore, these results indicate that some novel extracts derived from fermented mulberry leaves have very strong lipolytic activity.
To evaluate the cytotoxicity of EMfCs against adipocytes, the cell viability was measured in 3T3-L1 adipocytes following treatment with 200 µg/mL of 27 EMfCs. Among all extracts, the most groups treated EMfCs showed some low level of toxicity although their decrease rate was varied. However, eight groups treated with extracts purified using 95% EtOH (3M1-95, 3M2-95, 4M1-95, 4M2-95, 4M3-95, 6M1-95, 6M2-95 and 6M3-95) did not show any significant toxicity in 3T3-L1 cells compared with the Vehicle treated group (Figure 3). These results show that some extracts of 27 EMfCs can't induce any significant toxicity in 3T3L-1 adipocytes at dose of 200 µg/mL.
We finally selected two extracts with high lipolytic activity based on the results of the concentration of cAMP and toxicity (Table 2). To verify the dose dependent effects of these extracts on the lipolytic activity and toxicity, the concentration of cAMP, the levels of free glycerol and cell viability were measured in the culture medium of primary adipocytes and 3T3-L1 cells after treatment with 100, 200 and 400 µg/mL of the extracts. In two groups, the cAMP and glycerol level increased significantly in a dose dependent manner, with a high level being detected in the 400 µg/mL of the extracts treated groups (Figure 4A and B). But, the cell viability decreased in response to treatment with increasing concentrations of 4M3-95 (400 µg/mL), while 4M3-95 treated group were maintained a constant level (Figure 4C). Overall, these findings indicate that 4M3-95 may promote lipolysis of adipocytes derived from SD rats without any significant toxicity.
Cordycepin has been known to exhibit lipid lowering effects as well as multiple-biological effects including modulation of immune response, inhibition of tumor growth, hypotensive and vasorelaxation activities, and promoting secretion of adrenal hormone [22]. Therefore, the presence of the cordycepin in 4M3-95 was confirmed using HPLC analysis. As presented in Figure 5, the peak represented cordycepin were detected in the HPLC chromatogram of 4M3-95 under the optimal conditions, when it compared to standard cordycepin (100 µg/mL). Therefore, these results show that the lipolysis activity of 4M3-95 can be associated with codycepin, one of famous compounds with lipid-lowering effect, in the primary adipocytes.
Novel therapeutic drugs with lipolytic and lipogenetic activity have long received great attention because high accumulation of body fat is considered a major risk factor of chronic diseases including hypertension, diabetes, hyperlipidemia, cardiovascular disease and cancer [2324]. To date, many studies have reported that several lipolytic agents including norepinephrine, isoproterenol, forskolin, dibutyryl-cAMP (DBcAMP) and theophylline can induce lipolysis in fat cells [25]. Indeed, various natural products have been reported to be good alternative strategies to identify active compounds and develop effective and safe anti-obesity drugs [26]. In this study, we measured the free glycerol release of primary adipocytes treated with 27 EMfCs to identify novel natural products with lipolytic activity. The results demonstrated that several novel extracts derived from fermented mulberry have strong activity for lipolysis without any significant toxicity.
Previous studies have reported that extracts of mulberry leaves were associated with improved lipid metabolism. For example, analysis of lipolytic activity revealed the up-regulation of lipolysis-associated markers such as lipoprotein lipase in HFD fed mice treated with ethanol extract of mulberry leaves [19]. In addition, the anti-lipogenic activity of mulberry extracts has been investigated in 3T3-L1 cells and HFD fed rats. Extracts of mulberry leaves induced the down-regulation of some genes involved in lipogenesis, improvement of LXRα-mediated lipogenesis, and reduction of fat accumulation and adipogenesis markers [171819]. Finally, evaluation of hypolipidemic activity revealed that water extracts of mulberry leaves improved the plasma level of TG, HDL and LDL in atherogenic diet fed rats [27], while flavonoids isolated from mulberry leaves led to remarkable reductions in TG, TC and LDL-C levels in hyperlipidemic mice [28]. However, the effects of mulberry leaves fermented by various methods were not investigated. Therefore, we attempted to identify the novel extracts of fermented mulberry leaves with high lipolytic activity and no significant cytotoxicity. The results of our study showed some of the investigated extracts could stimulate lipolysis in primary adipocytes.
It is important to consider toxicological problems when developing novel drugs for treatment of human diseases and to extend the therapeutic potential of conventional drugs [29]. Several recent studies investigated the toxicity of mulberry leaf extracts in 3T3-L1 cells and mice. No significant effects on cell viability were detected in 3T3-L1 adipocytes treated with 100 µg/mL of ethanol extracts of mulberry leaves [18]. However, this extract induced significant toxicity in mice. Specifically, oral administration of this extract (2,000 mg/kg) induced a reduction of immune cells, turgidity of contorted tubules in kidneys, infiltration of leukocytes around the liver centrilobular vein, and high dispersion of spleen white pulp [30]. Moreover, intraperitoneal administration of 300 and 2,000 mg/kg bw of mulberry leaf ethanol extracts for 14 days induced a greater degree of toxicity toward biochemical, hematological, and histological parameters in mice [30]. In the current study, aqueous extracts of mulberry leaves administered orally at doses of 1,000, 2,000 and 4,000 mg/kg bw did not induce any significant alterations in bw/weight gain, food consumption, ophthalmoscopy, clinical pathology, gross pathology, organ weights or histopathology over 28 days [31]. Our findings for EMfCs were differenced from those observed upon investigation of the cell viability of 3T3-L1 adipocytes treated with 100 µg/mL of ethanol extract of mulberry leaves, because the samples were prepared using different methods and treated with different dosage. Finally, the results of the present study revealed that the no observed adverse effect level of most extracts purified using 95% EtOH was 200 µg/mL for adipocytes.
In conclusion, the results of the present study indicated that one extracts (4M3-95) of fermented mulberry leaves can stimulate lipolysis in the primary adipocytes without any significant cytotoxicity. These findings also indicate that this extract hase the potential for use as lipolytic agents for the treatment of obesity.
Acknowledgment
This research was supported by grants to Dr. Dae Youn Hwang from the Korea Institute of Planning Evaluation for Technology of Food, Agriculture, Forestry and Fisheries (116027-032-HD030).
References
1. Ho JN, Kim OK, Nam DE, Jun W, Lee J. Pycnogenol supplementation promotes lipolysis via activation of cAMP-dependent PKA in ob/ob mice and primary-cultured adipocytes. J Nutr Sci Vitaminol (Tokyo). 2014; 60(6):429–435. PMID: 25866307.
2. Lafontan M, Langin D. Lipolysis and lipid mobilization in human adipose tissue. Prog Lipid Res. 2009; 48(5):275–297. PMID: 19464318.


3. Langin D, Dicker A, Tavernier G, Hoffstedt J, Mairal A, Rydén M, Arner E, Sicard A, Jenkins CM, Viguerie N, van Harmelen V, Gross RW, Holm C, Arner P. Adipocyte lipases and defect of lipolysis in human obesity. Diabetes. 2005; 54(11):3190–3197. PMID: 16249444.


4. Carmen GY, Víctor SM. Signaling mechanisms regulating lipolysis. Cell Signal. 2006; 18(4):401–408. PMID: 16182514.
5. Zimmermann R, Strauss JG, Haemmerle G, Schoiswohl G, Birner-Gruenberger R, Riederer M, Lass A, Neuberger G, Eisenhaber F, Hermetter A, Zechner R. Fat mobilization in adipose tissue is promoted by adipose triglyceride lipase. Science. 2004; 306(5700):1383–1386. PMID: 15550674.


6. Ho JN, Jang JY, Yoon HG, Kim Y, Kim S, Jun W, Lee J. Antiobesity effect of a standardised ethanol extract from Curcuma longa L. fermented with Aspergillus oryzae in ob/ob mice and primary mouse adipocytes. J Sci Food Agric. 2012; 92(9):1833–1840. PMID: 22278718.
7. Hansson B, Medina A, Fryklund C, Fex M, Stenkula KG. Serotonin (5-HT) and 5-HT2A receptor agonists suppress lipolysis in primary rat adipose cells. Biochem Biophys Res Commun. 2016; 474(2):357–363. PMID: 27109474.


8. Bown D. Encyclopedia of herbs and their uses. London, UK: Dorling Kindersley;1995. p. 313–314.
9. Flores MB, Rocha GZ, Damas-souza DM, Osorio-costa F, Dias MM, Ropelle ER, Camargo JA, Carvalho RB, Carvalho HF, Saad MJ, Carvalheira JB. Obesity-induced increase in tumor necrosis factor-alpha leads to development of colon cancer in mice. Gastroenterol. 2012; 143(3):741–753.
10. Taniguchi S, Asano N, Tomino F, Miwa I. Potentiation of glucose-induced insulin secretion by fagomine, a pseudo-sugar isolated from mulberry leaves. Horm Metab Res. 1998; 30(11):679–683. PMID: 9918385.


11. Chen F, Nakashima N, Kimura I, Kimura M. Hypoglycemic activity and mechanisms of extracts from mulberry leaves (folium mori) and cortex mori radicis in streptozotocin-induced diabetic mice. Yakugaku Zasshi. 1995; 115(6):476–482. PMID: 7666358.
12. Kobayashi A, Kang MI, Watai Y, Tong KI, Shibata T, Uchida K, Yamamoto M. Oxidative and electrophilic stresses activate Nrf2 through inhibition of ubiquitination activity of Keap1. Mol Cell Biol. 2006; 26(1):221–229. PMID: 16354693.


13. Azman KF, Amom Z, Azlan A, Esa NM, Ali RM, Shah ZM, Kadir KK. Antiobesity effect of Tamarindus indica L. pulp aqueous extract in high-fat diet-induced obese rats. J Nat Med. 2012; 66(2):333–342. PMID: 21989999.
14. Ou TT, Hsu MJ, Chan KC, Huang CN, Ho HH, Wang CJ. Mulberry extract inhibits oleic acid-induced lipid accumulation via reduction of lipogenesis and promotion of hepatic lipid clearance. J Sci Food Agric. 2011; 91(15):2740–2748. PMID: 22002411.


15. Ann JY, Eo H, Li Y. Mulberry leaves (Morus alba L.) ameliorate obesity-induced hepatic lipogenesis, fibrosis, and oxidative stress in high-fat diet-fed mice. Genes Nutr. 2015; 10(6):46–58. PMID: 26463593.


16. Arabbi PR, Genovese MI, Lajolo FM. Flavonoids in vegetable foods commonly consumed in Brazil and estimated ingestion by the Brazilian population. J Agric Food Chem. 2004; 52(5):1124–1131. PMID: 14995109.


17. Kobayashi Y, Miyazawa M, Kamei A, Abe K, Kojima T. Ameliorative effects of mulberry (Morus alba L.) leaves on hyperlipidemia in rats fed a high-fat diet: induction of fatty acid oxidation, inhibition of lipogenesis, and suppression of oxidative stress. Biosci Biotechnol Biochem. 2010; 74(12):2385–2395. PMID: 21150120.
18. Yang SJ, Park NY, Lim Y. Anti-adipogenic effect of mulberry leaf ethanol extract in 3T3-L1 adipocytes. Nutr Res Pract. 2014; 8(6):613–617. PMID: 25489399.


19. Ann JY, Eo HY, Lim YS. Mulberry leaves (Mours albla L.) ameliorate obesity-induced hepatic lipogenesis, fibrosis, and oxidative stress in high-fat diet-fed mice. Genes Nutr. 2015; 10:46–58. PMID: 26463593.


20. Naowaboot J, Pannangpetch P, Kukongviriyapan V, Prawan A, Kukongviriyapan U, Itharat A. Mulberry leaf extract stimulates glucose uptake and GLUT4 translocation in rat adipocytes. Am J Chin Med. 2012; 40(1):163–175. PMID: 22298456.


21. Sugimoto M, Arai H, Taura Y, Murayama T, Khaengkhan P, Nishio T, Ono K, Ariyasu H, Akamuzu T, Ueda Y, Kita T, Harada S, Kamei K, Yokode M. Mulberry leaf ameliorates the expression profile of adipocytokines by inhibiting oxidative stress in white adipose tissue in db/db mice. Atherosclerosis. 2009; 204(2):388–394. PMID: 19070857.


22. Gao J, Lian ZQ, Zhu P, Zhu HB. Lipid-lowering effect of cordycepin (3'-deoxyadenosine) from Cordyceps militaris on hyperlipidemic hamsters and rats. Yao Xue Xue Bao. 2011; 46(6):669–676. PMID: 21882527.
24. Apostolopoulou M, Savopoulos C, Michalakis K, Coppack S, Dardavessis T, Hatzitolios A. Age, weight and obesity. Maturitas. 2012; 71(2):115–119. PMID: 22226988.


25. Morimoto C, Kameda K, Tsujita T, Okuda H. Relationships between lipolysis induced by various lipolytic agents and hormone-sensitive lipase in rat fat cells. J Lipid Res. 2001; 42(1):120–127. PMID: 11160373.


26. Mayer MA, Hcht C, Puyü A, Taira CA. Recent advances in obesity pharmacotherapy. Curr Clin Pharmacol. 2009; 4(1):53–61. PMID: 19149502.


27. Lee YJ, Choi DH, Kim EJ, Kim HY, Kwon TO, Kang DG, Lee HS. Hypotensive, hypolipidemic, and vascular protective effects of Morus alba L. in rats fed an atherogenic diet. Am J Chin Med. 2011; 39(1):39–52. PMID: 21213397.
28. Chen J, Li X. Hypolipidemic effect of flavonoids from mulberry leaves in triton WR-1339 induced hyperlipidemic mice. Asia Pac J Clin Nutr. 2007; 16:290–294. PMID: 17392121.
29. Parasuraman S. Toxicological screening. J Pharmacol Pharmacother. 2011; 2(2):74–79. PMID: 21772764.


30. de Oliveira AM, Mesquita Mda S, da Silva GC, de Oliveira Lima E, de Medeiros PL, Paiva PM, de Souza IA, Napoleão TH. Evaluation of toxicity and antimicrobial activity of an ethanolic extract from leaves of Morus alba L. (Moraceae). Evid Based Complement Alternat Med. 2015; 2015:513978. PMID: 26246840.
31. Marx TK, Glávits R, Endres JR, Palmer PA, Clewell AE, Murbach TS, Hirka G, Pasics I. A 28-day repeated dose toxicological study of an aqueous extract of Morus Alba L. Int J Toxicol. 2016; 35(6):683–691. PMID: 27733446.
Figure 1
Schematic procedure of EMfCs preparation. After preparation of mulberry leaves powder and silkworm pupae powder, 27 EMfCs were extracted from the fermented mulberry leaves powder using dH2O, 50% EtOH and 90% EtOH as described in the Materials and Methods.
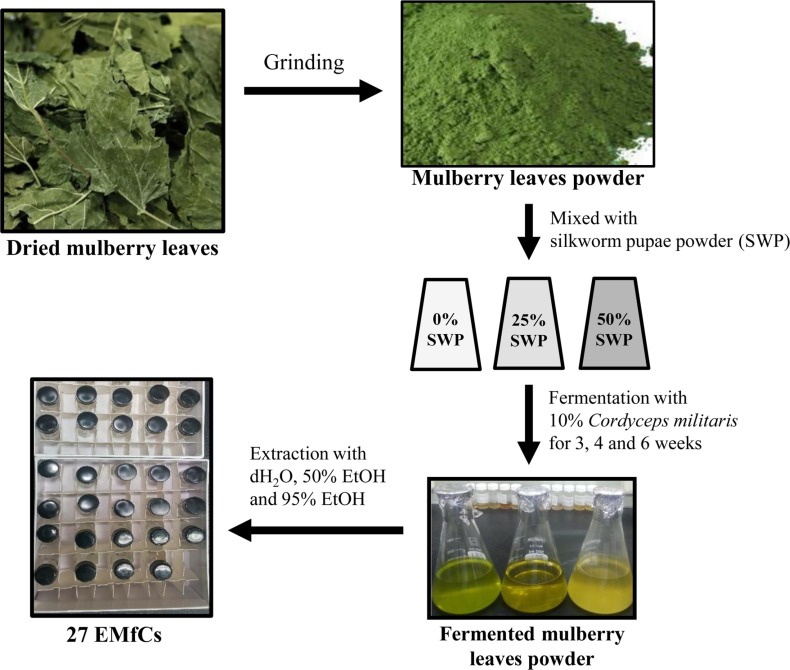
Figure 2
Analysis of cAMP concentration. After 27 EMfCs treatment, the concentration of cAMP was measured in the supernatant of primary adipocytes treated with 27 EMfCs (200 µg/mL) that were fermented for 3 weeks (A), 4 weeks (B) and 6 weeks (C). The cell images of the three groups were observed with an inverted microscope at 100× magnification. The data shown represent the means±SD of three replicates. *P<0.05 relative to the Vehicle treated group. ISOP; isoproterenol.
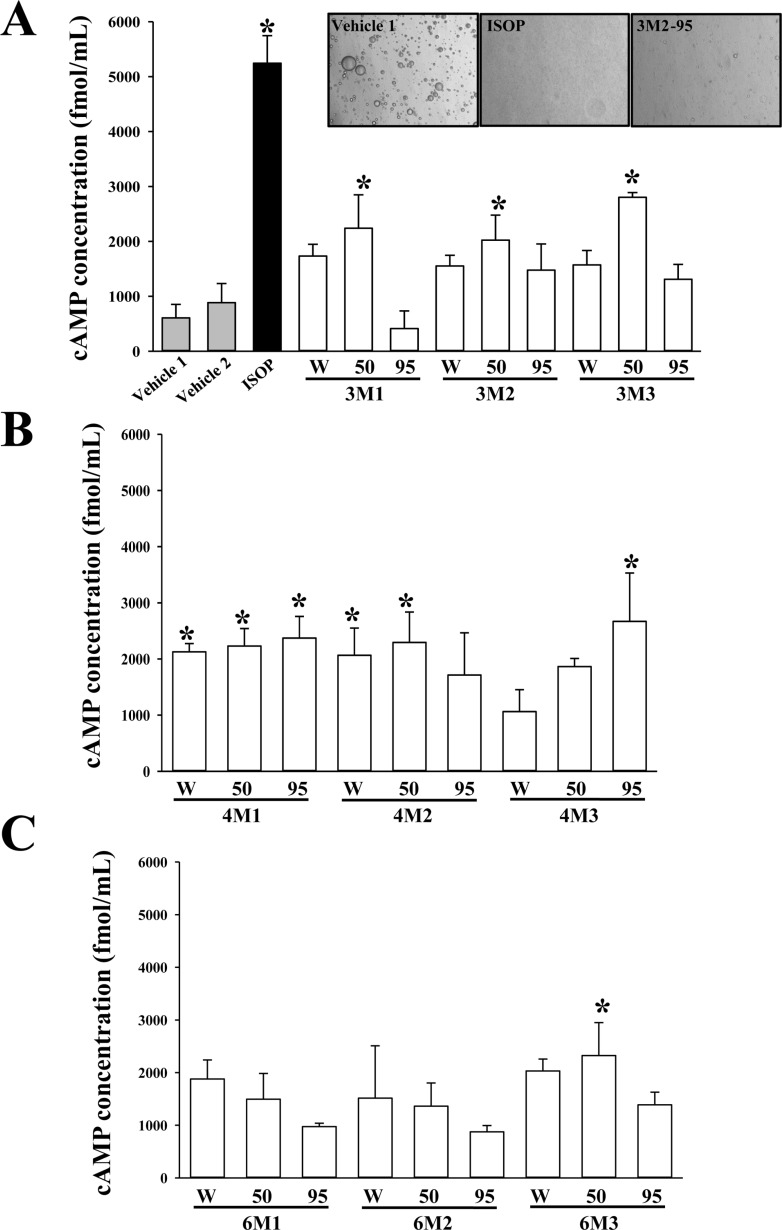
Figure 3
Cytotoxicity of 27 EMfCs. The cell viability was measured in 3T3-L1 adipocytes treated with 27 EMfCs (200 µg/mL) that were fermented for 3 weeks (A), 4 weeks (B) and 6 weeks (C). The cell images of the three groups were observed with an inverted microscope at 100× magnification. The data shown represent the means±SD of three replicates. *P<0.05 relative to the Vehicle treated group.
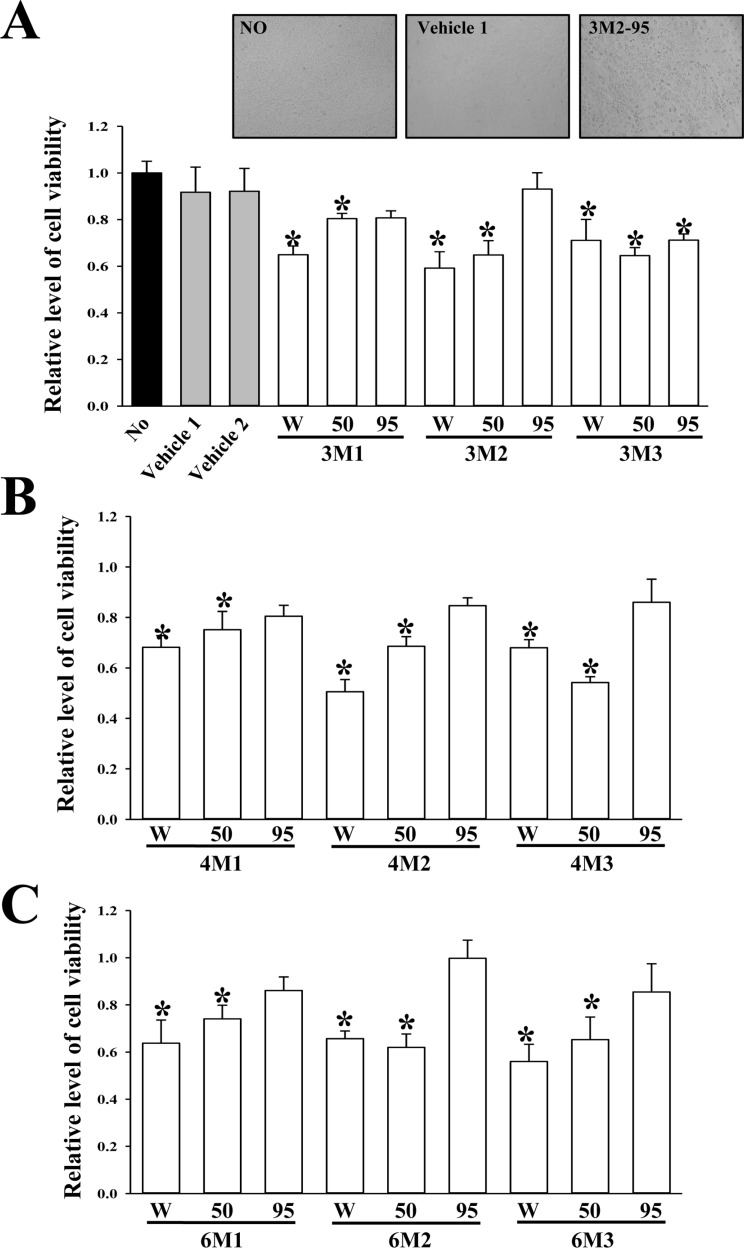
Figure 4
Dose dependent response of two candidates with strong lipolytic activity. The concentration of cAMP (A), free glycerol release (B) and cell toxicity (C) were measured in primary adipocytes and 3T3-L1 cells treated with 100, 200 and 400 µg/mL of two candidates that were fermented for 4 weeks. The data shown represent the means±SD of three replicates. *P<0.05 relative to the Vehicle treated group. ISOP; isoproterenol.
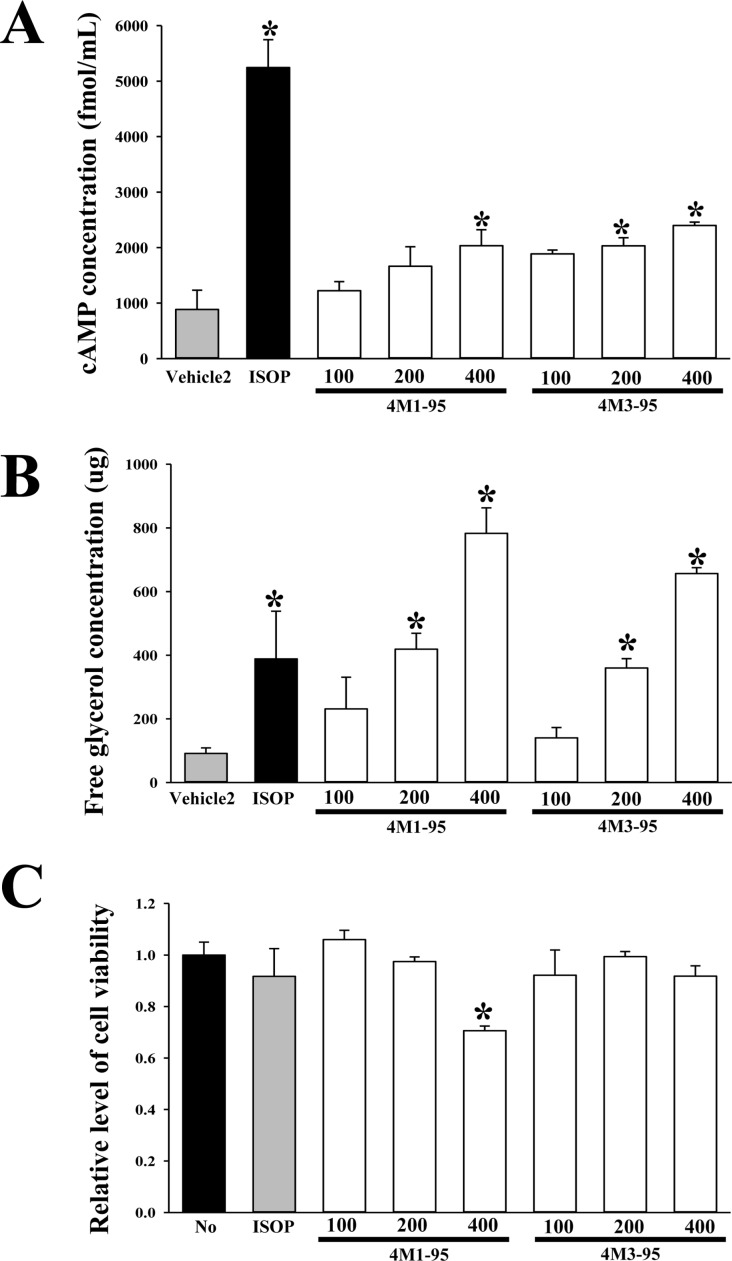
Figure 5
Chromatograms of 4M3-95 containing cordycepin. High performance liquid chromatography were performed the specific condition described in Materials and Methods section. The peak height/area reflected the concentration of the cordycepin in 4M3-95.
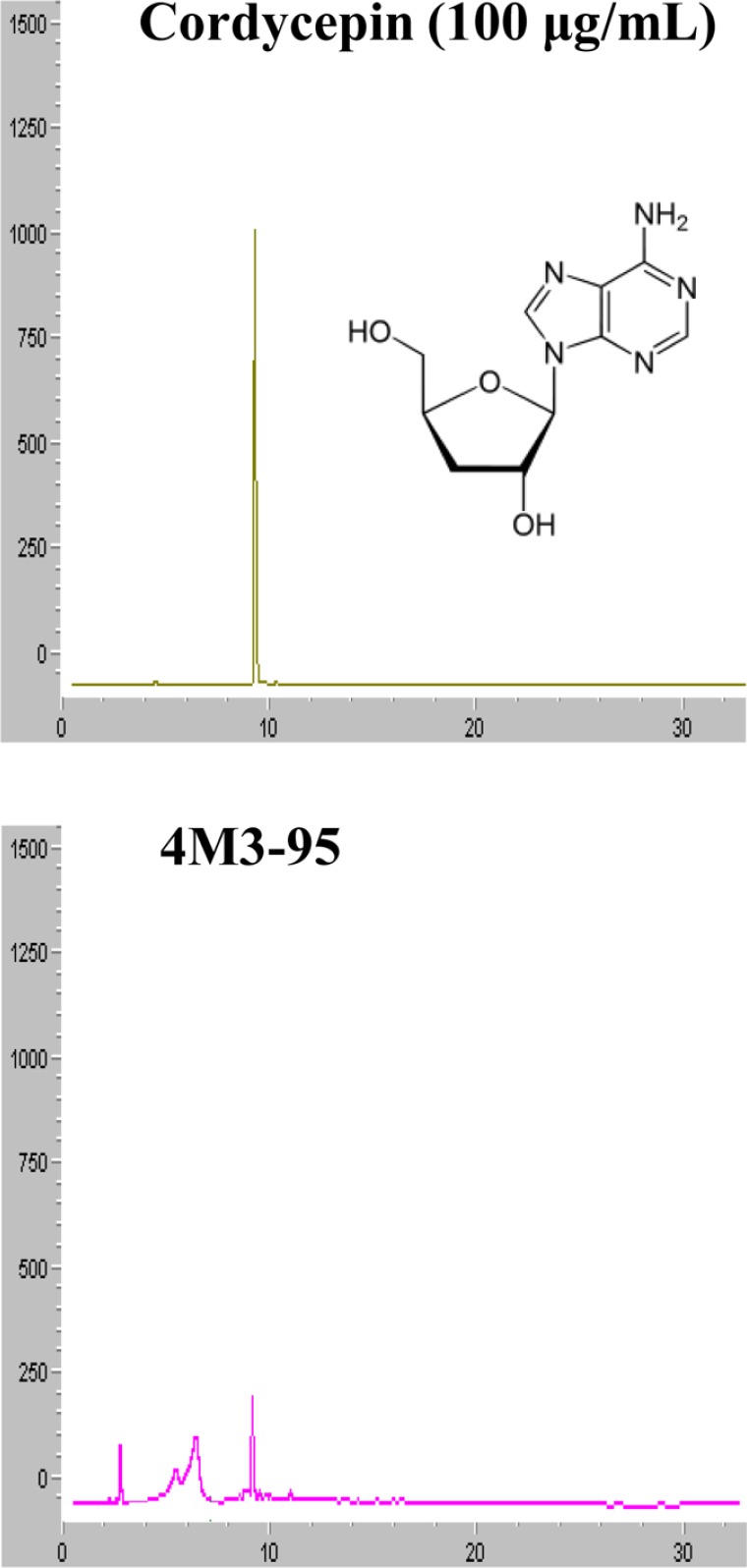
Table 1
List of final products used in this study
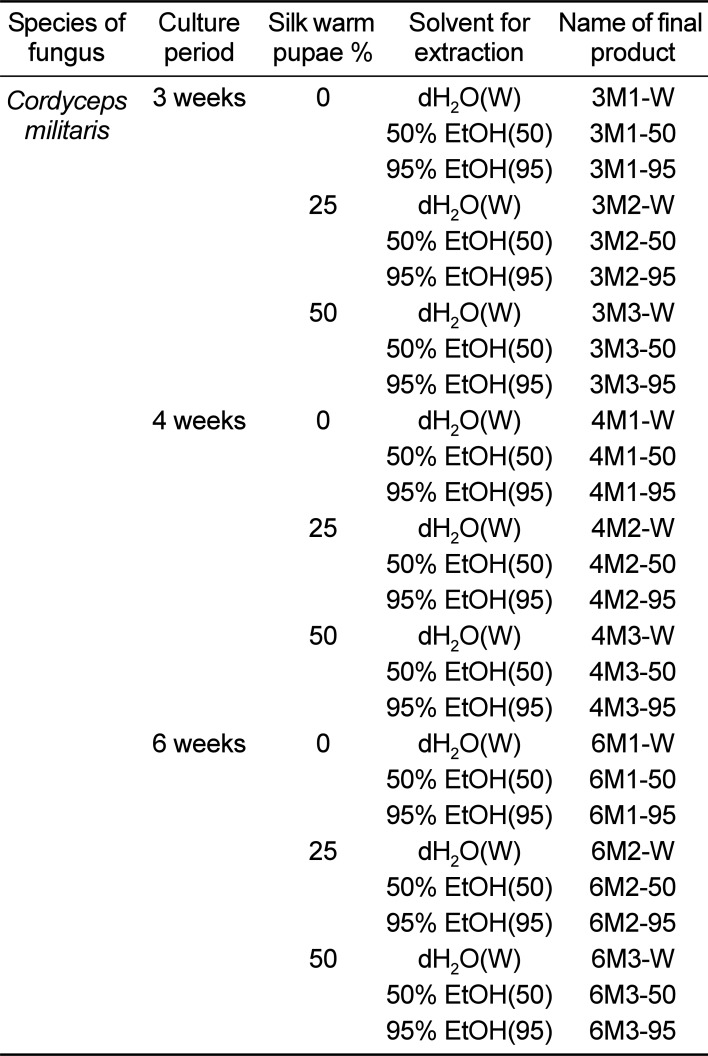
Table 2
Selection of candidate extracts based on the concentration of cAMP and toxicity
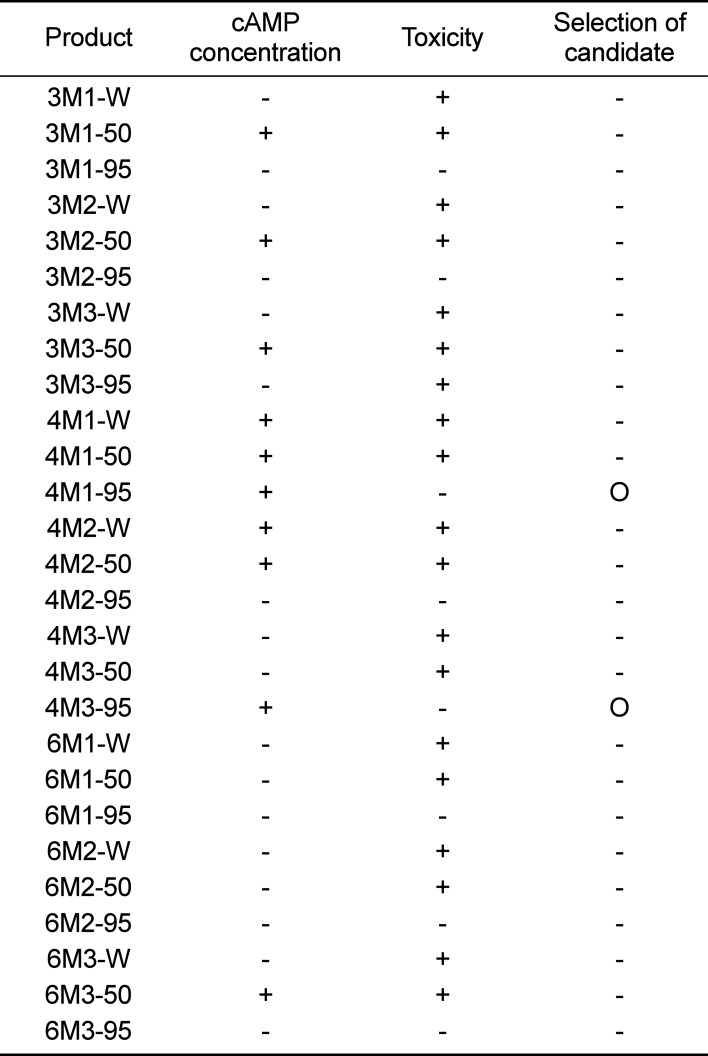