This article has been
cited by other articles in ScienceCentral.
Abstract
Breast cancer is the second most common cancer and the most frequent cancer in women worldwide. Recent improvements in early detection and effective adjuvant chemotherapies have improved the survival of breast cancer patients. Even with initial disease remission, one-third of all breast cancer patients will relapse with distant metastasis. Breast cancer metastasis is largely an incurable disease and the main cause of death among breast cancer patients. Cancer metastasis is comprised of complex processes that are usually not controllable by intervention of a single molecular target. As a single microRNA (miRNA) can affect the aggressiveness of breast cancer cells by concurrently modulating multiple pathway effectors, a metastasis-regulating miRNA would represent a good disease target candidate. In this study, we evaluated the functional capacity of a newly defined human metastasis-related miRNA, miR-766, which was previously identified by comparing a patient-derived xenograft primary tumor model and a metastasis model. Compared to vector-transfected control cells, miR-766-overexpressed triple-negative breast cancer cells exhibited similar primary tumor growth in the orthotopic xenograft model. In contrast, tumor sphere formation and Matrigel invasion were significantly decreased in miR-766-overexpressed breast cancer cells compared with control cancer cells. In addition, lung metastasis was dramatically reduced in miR-766-overexpressed breast cancer cells compared with control cells. Thus, miR-766 affected the distant metastasis process to a greater extent than cancer cell proliferation and primary tumor growth, and may represent a future therapeutic target to effectively control fatal breast cancer metastasis.
Go to :

Keywords: Human triple-negative breast cancer, microRNA, metastasis
Breast cancer is the second most common cancer and the most frequent cancer in women worldwide [
1]. Breast cancer is divided into four subtypes depending on the expression patterns of surface receptors (i.e., estrogen receptor (ER), progesterone receptor (PR), and the erbB-2 receptor (Her2)). Luminal type breast cancers express ER and/or PR and, depending on the expression of Her2, the luminal type is subdivided into luminal type A, which expresses Her2, and luminal type B, which does not. Her2-overexpressing-type breast cancers express Her2 but not ER or PR. Triple-negative breast cancers do not express ER, PR, or Her2, and comprise approximately 20% of all breast cancer cases [
2]. Due to the development of targeted therapies using several types of estrogen antagonists for luminal-type breast cancers and an anti-Her2 antibody (Herceptin) for Her2-overexpressing-type breast cancer, the survival and prognosis of these subtypes have improved dramatically [
3]. In contrast, triple-negative breast cancers do not have a targeted therapy and are only treated by conventional chemotherapy. However, recent improvements in early detection and effective adjuvant chemotherapies have improved the survival of triple-negative breast cancer patients. Even with initial disease remission, over half of all triple-negative breast cancer patients will relapse with distant metastasis [
4].
Breast cancer metastasis is largely an incurable disease and the main cause of death among breast cancer patients [
5]. Cancer metastasis is comprised of complex processes, including dissociation of cells from the primary tumor, invasion into surrounding tissue, invasion into the blood or lymphatic vessels, transport through the vascular system, export out of the vascular system, implantation of disseminated cells at ectopic sites, and finally outgrowth of micrometastatic cancer cells to form a metastatic tumor mass [
6]. We previously reported that both cancer cell intrinsic and extrinsic factors and cells are involved in modulating breast cancer cell aggressiveness, thus affecting primary tumor growth, tissue invasion, and distant metastasis [
78]. The transglutaminase 2-nuclear factor kappa B (NF-κB)-interleukin 6 (IL-6) signaling axis is important in human triple-negative breast cancer cells, providing aggressiveness [
7]. Myeloid-derived suppressor cells (MDSCs), recruited by murine triple-negative breast cancer cells, cause cancer cells to prolong the expression of pSTAT3 and increase distant metastasis of these cells [
8].
MicroRNAs (miRNAs) are a type of non-coding RNA synthesized by RNA polymerase II and are sequentially processed by a specialized ribonuclease III enzyme, Drosha, in the nucleus and by a second RNase III enzyme, Dicer, in the cytoplasm and associate with Argonaute family proteins to form miRNA-induced silencing complexes [
9]. Post-translational silencing of complementary mRNA targets is accomplished through a combination of translational repression and mRNA destabilization depending on the seed pairing and/or 3 supplementary and compensatory pairings [
10]. As each miRNA has the potential to target hundreds of mRNAs and most mRNAs are conserved targets of miRNAs in mammals [
11], a single miRNA can affect a series of relevant signaling molecules in a specific signaling or biological pathway. A typical example is miR-205, which inhibits ZEB, Her3, and vascular endothelial growth factor (VEGF), each of which is involved in different processes of metastasis, such as epithelialmesenchymal transition (EMT), colonization in remote sites, and angiogenesis, respectively [
12]. Thus, a single miRNA can affect the aggressiveness of breast cancer cells by concurrently modulating multiple pathway effectors involved in cancer cell evolution and metastasis.
In this study, we evaluated the functional capacity of a newly defined human metastasis-related miRNA, miR-766, which was previously identified by comparing a patient-derived xenograft (PDX) primary tumor model and a metastasis model [
13]. Compared to vector-transfected control cells, miR-766-overexpressed MDA-MB-231 triple-negative breast cancer cells exhibited similar primary tumor growth in the orthotopic xenograft model in NOD/scid/IL-2Rγ
−/− (NSG) mice [
14]. In contrast, tumor sphere formation was significantly decreased in miR-766-overexpressed MDA-MB-231 cells compared with control cancer cells. In addition, miR-766-overexpressed MDA-MB-231 cells showed reduced invasion into the Matrigel in the invasion assay compared with control cells. The effector phase of hematogenous metastasis was evaluated via intravenous injection of breast cancer cells. Although similar primary tumor growth in the mammary fat pads was observed between cell types, lung metastasis was dramatically reduced in miR-766-overexpressed MDA-MB-231 cells compared with control cells. Thus, miR-766 affected the distant metastasis process to a greater extent than cancer cell proliferation and primary tumor growth.
Materials and Methods
Cell lines and miR-766 overexpression
MDA-MB-231 human triple-negative breast cancer cells were obtained from the American Type Culture Collection (Manassas, VA). Human miR-766-overexpressing MDA-MB-231 cells (MB231_miR-766) were established by stable transfection using the full sequence of human miR-766. Briefly, MDA-MB-231 cells were transfected with the pcDNA3.1 plus pSUPER plasmid containing full sequence of human miR-766 using Promofectin (PromoKine, Heidelberg, Germany), according to the manufacturer's instruction. Stable transfected clones were established by selection with G418 (Sigma-Aldrich, St. Louis, MO) at a concentration of 500 µg/mL for 3 weeks. Control cells were transfected with the pcDNA3.1 vector only (MB231_Vector).
Animals and xenografts
All mice were bred and maintained in specific pathogen-free conditions at the animal facility of Seoul National University College of Medicine. All animal experiments were performed with the approval of the institutional animal care and use committee of Seoul National University.
To assess tumorigenicity of the cancer cells, human breast cancer MDA-MB-231 cells (2×105 cells/each fatpad/each mouse), stably transfected with full sequence of human miR-766 or empty vector, were injected into the mammary fatpads into 8-week-old NOD/scid/IL-2Rγ −/− (NSG) mice (Jackson Laboratory, Bar Harbor, ME). Six weeks after the injection, the mice were euthanized and primary tumor masses in the fatpads, livers, and lungs were fixed in 4% paraformaldehyde for 24 h, and embedded in paraffin. Sections (4 µm) were stained with hematoxylin and eosin for histopathological analysis. The number of tumor masses in the liver and lung were counted under a dissecting microscope.
Invasion assay
Matrigel matrix solution (200 µg/mL, Matrigel™ Basement Membrane Matrix, BD Bioscience) was applied to each Transwell (FALCON). MDA-MB-231 cells (5×10
4) were seeded into the upper chamber of the Transwell and then the lower chamber was filled with collagen matrix (5 µg/mL). Invasion assays were carried out for 18 h. Non-invading cells on top of the matrix were removed by rubbing with a moistened cotton swab. Invaded cells on the lower surface of the Matrigel matrix were fixed with 4% PFA and stained with 0.2% crystal violet. Cells were counted using ImageJ software (version 1.46). (
http://rsb.info.nih.gov/ij/).
Sphere formation
Cells grown in an anchorage-independent condition were trypsinized, washed twice with PBS, and resuspended in serum-free medium consisting of a 1:1 mixture of F-12 Nutrient Mixture (Ham's F-12) and DMEM, supplemented with 20 ng/mL epithelial growth factor (Invitrogen, Carlsbad, CA), 20 ng/mL basic fibroblast growth factor (Millipore, Billerica, MA), 0.5 nM hydrocortisone (Sigma-Aldrich), B-27 supplement (GIBCO), 2 mM L-glutamine (GIBCO), and 1% antibiotics (100 U/mL penicillin and 100 mg/mL streptomycin). Cells were plated on a non-adherent 96-well plate (Corning, Corning, NY) at 10, 100, or 1000 cells/100 µL/well. The medium was replaced every 3 days. Visible spheres were counted under a microscope on day 8 post-plating.
Histopathology of lung tissue
Lung tissues were fixed in 4% paraformaldehyde, processed, and embedded in paraffin. Sections were stained with H&E for histopathological analysis.
Statistical analyses
The two-tailed Student's t-test was used to compare measurements for pairs of samples. Two-way analysis of variance (ANOVA) and Bonferroni post hoc testing were used to compare the tumor volumes of the two groups. The SPSS software (SPSS Inc.) was used for all statistical analyses.
Go to :

Results
Primary tumor growth was similar in the orthotopic mammary fat pads in miR-766-overexpressed breast cancer cells
To evaluate the role of miR-766 in breast cancer cell growth and metastasis, the metastatic human triple-negative breast cancer cell line MDA-MB-231 was used. MDA-MB-231 breast cancer cells were stably transfected with miR-766 and vector only-transfected cells were used as controls. Breast cancer cell behavior
in vivo was evaluated using highly immunocompromised NOD/scid/IL-2Rg chain-deficient (NSG) mice, which completely lack natural killer activity, as well as T and B cell activity, as recipients [
14].
Overexpression of miR-766 did not alter the primary tumor growth of MDA-MB-231 breast cancer cells. Both vector only-transfected control cells (MB231_Vector-GFP) and miR-766-overexpressed breast cancer cells (MB231_miR-766-GFP) grew similarly in the orthotopic mammary fat pads (
Figure 1A). We also conducted experiments using 4L breast cancer cells, which were obtained by serially grafting MDA-MB-231 cells in the mammary fat pads and collecting lung metastatic cells four times. 4L cells have the propensity to metastasize to the lung. When we compared 4L cells overexpressed with miR-766-(4L_miR-766) and vector-transfected (4L_Vector) cells, both breast cancer cells grew equivocally in the mammary fat pads (
Figure 1B). Thus, miR-766 did not affect the primary tumor growth of triple-negative breast cancer cells in the mammary fat pads.
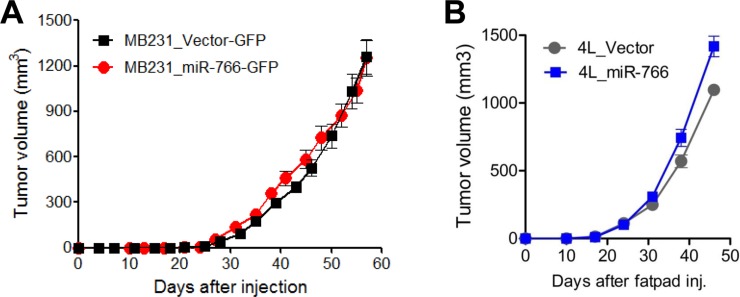 | Figure 1Primary tumor growth was similar in the orthotopic mammary fat pads in miR-766-overexpressed breast cancer cells. (A) Control and miR-766 overexpressed MDA-MB-231 triple-negative breast cancer cells (2×105 cells/each mouse) (MB231_Vector-GFP and MB231_miR-766-GFP, respectively) were injected in the fatpads of NSG mice. The growth of primary tumors was measured. (B) Control and miR-766 overexpressed 4L triple-negative breast cancer cells (2×105 cells/each mouse) (4L_Vector and 4L_miR-766, respectively) were injected in the fatpads of NSG mice. The growth of primary tumors was measured. (A,B) Data are given as mean±SD of 7 mice for each group from two independent experiments.
|
Reduced tumor sphere formation and invasion in miR-766-overexpressed breast cancer cells
For cancer cells to metastasize, they need to invade the surrounding tissue and blood or lymphatic vessels and circulate through the vascular system. During circulation, cancer cells must survive in anchorage-independent situations (i.e., anoikis-resistant), one of the key characteristics of stem cells. To evaluate whether the stem cell characteristics of MDA-MB-231 cells were altered by miR-766 overexpression, a tumor sphere formation assay was conducted. Tumor sphere formation was decreased in miR-766-overexpressed breast cancer cells (MB231_miR-766) compared with vector only control cells (MB231_Vector). Both the size and number of tumor spheres were decreased in miR-766-overexpressed cells (
Figure 2).
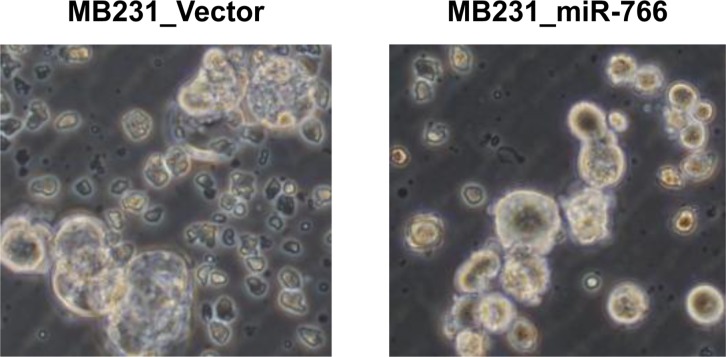 | Figure 2Reduced tumor sphere formation in miR-766-overexpressed breast cancer cells. Control and miR-766 overexpressed MDA-MB-231 cells (MB231_Vector and MB231_miR-766, respectively) were cultured in serum-free medium consisting of a 1:1 mixture of Ham's F-12 and DMEM, supplemented with growth factors. Phase-contrast microscopy of day 8 spheres generated from the MDA-MB-231 cell line. Data shown are representatives of three independent experiments.
|
To evaluate the movement of cancer cells in the extracellular matrix, a two-dimensional (2D) Matrigel invasion assay was performed. miR-766-overexpressed breast cancer cells (MB231_miR-766) exhibited reduced invasion into the Matrigel compared with vector only control breast cancer cells (MB231_Vector) (
Figure 3).
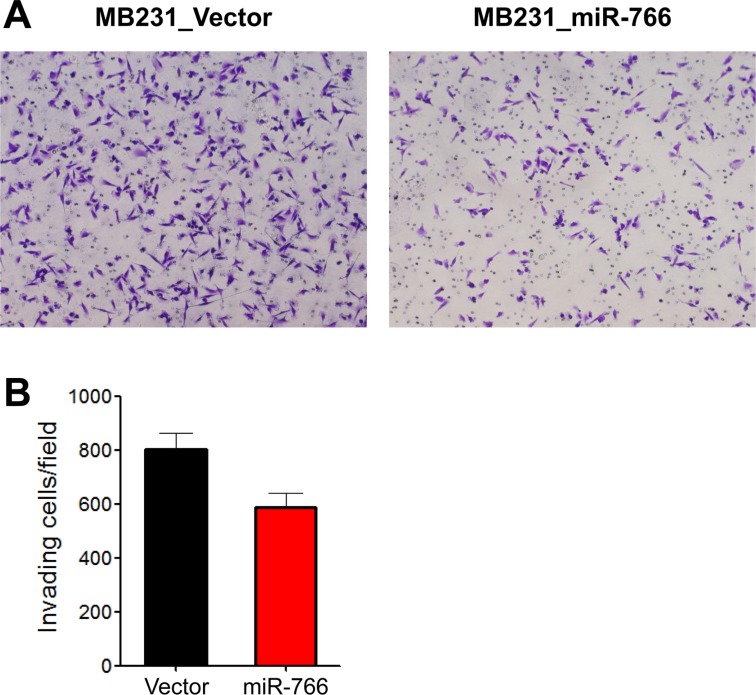 | Figure 3Reduced tumor cell invasion in miR-766-overexpressed breast cancer cells. A two-dimensional (2D) Matrigel invasion assay was performed. Control and miR-766 overexpressed MDA-MB-231 cells (MB231_Vector and MB231_miR-766, respectively) (5×104) were seeded into the upper chamber of the Transwell and then the lower chamber was filled with collagen matrix (5 µg/mL). Invasion assays were carried out for 18 h. Invaded cells were fixed with 4% PFA and stained with 0.2% crystal violet. (A) Photomicrographs of invasion assay. Data shown are representatives of each group. (B) Cell count. Data are given as mean±SD from three independent experiments.
|
Reduced lung metastasis in miR-766-overexpressed breast cancer cells
To evaluate the effect of miR-766 overexpression on the metastatic ability of triple-negative breast cancer cells, miR-766-overexpressed and vector only control breast cancer cells (2×10
5/ea) were intravenously injected into NSG mice and the lungs were analyzed five weeks later. Vector-only control breast cancer cells (MB231_Vector) formed multiple and large-sized metastatic nodules in the lung, which covered over half of the total lung area (
Figure 4). In contrast, miR-766-overexpressed breast cancer cells (MB231_miR-766) exhibited reduced lung metastasis compared with control cells (MB231_Vector), both in the metastatic nodule number and tumor area in the lung (
Figure 4).
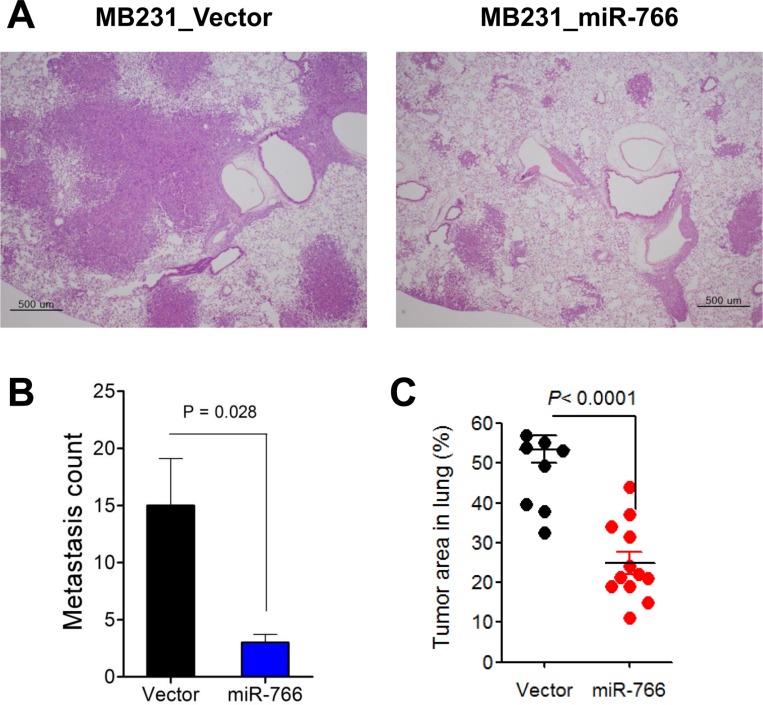 | Figure 4Reduced lung metastasis in miR-766-overexpressed breast cancer cells. Control and miR-766 overexpressed MDA-MB-231 triple-negative breast cancer cells (2×105 cells/each mouse) (MB231_Vector and MB231_miR-766, respectively) were intravenously injected into NSG mice. At five weeks after injection of cancer cells, the mice were sacrificed and distant metastases in the lung were analyzed. (A) Lung sections were obtained and stained with hematoxylin and eosin (original magnification, 200×). Data shown are representatives of each group. (B) Metastasis count in the lung. (C) Tumor area (%) in the lung. (B,C) Data are given as mean±SD of 8 and 12 mice for control and miR-766 overexpressed group, respectively.
|
Go to :

Discussion
Triple-negative breast cancer is the most aggressive subtype, comprises approximately 20% of all breast cancer cases, and is characterized by not expressing hormone receptors (e.g., ER and PR) or the Her2 receptor [
2]. Triple-negative breast cancer overlaps with basal-type breast cancers, which are classified according to their gene expression profile and characteristics of basal stem cells, which produce mammary glands and ducts [
15]. Improvements in neoadjuvant chemotherapeutic combinations and effective postsurgical radio- and chemotherapy have caused the initial remission rate and 5-year survival rate of triple-negative breast cancer patients to increase during the last 20 years. However, approximately two-fifths of patients do not respond to initial chemotherapy, and most patients who respond initially eventually recur with frequent metastasis [
16]. Compared with the other breast cancer subtypes, triple-negative breast cancers do not have a targeted therapy. In this aspect, therapeutic molecular targets specific for this breast cancer subtype need to be identified and validated.
Molecular targets affecting cancer cell proliferation, survival, invasion, drug resistance, and distant metastasis have been found based on various research approaches [
617]. Metastasis-initiating genes include those regulating cancer cell motility, angiogenesis, and local tissue invasion, are not valid targets for blocking metastasis unless they also play critical roles in the later stages of the metastatic cascade [
18]. However, genes that promote target tissue infiltration, survival, and colonization of distant organs, which are known as metastasis progression genes, are attractive targets that, if expressed in the primary tumor, may also be predictive markers of relapse with organ-specific metastasis [
18]. The process of metastasis is complex; many factors are involved and interconnected, and some are redundant thus reinforcing the circuit of the metastatic process. As such, single target therapy may not be sufficient to effectively control the process of ever-evolving breast cancer cells, which could lead to distant metastasis and drug sresistance [
19]. Thus, specific targets that are evolutionarily conserved and harmoniously affect several key and related points during breast cancer metastasis would be more ideal molecular targets. In this respect, miRNAs affecting putative biological pathways of cancer cells can be effective therapeutic targets [
20]. Several miRNAs have been characterized as metastasis-related. Metastasis-suppressive miRNAs, such as miR-34a, miR-200, and miR-335, decrease cancer metastasis by regulating metastasis-enhancing genes, such as CD44, Fra-1, SNAIL, ZEB1/2, SOX4, and Tenascin-C, and metastasis-promoting miRNAs, such as miR-10b, miR-21, and miR-103/107, which contribute to metastasis by regulating metastasis-inhibiting genes, such as HOXD10, PGCD4, TPM1, Maspin, and Dicer [
21].
We previously established and examined a PDX model in NSG mice using primary tumor tissue of breast cancer patients from Seoul National University Hospital [
13]. By serially resecting the primary tumor mass developed in NSG mice, we succeeded in constructing a metastatic PDX model with spontaneous metastasis to the lung and axillary lymph nodes. mRNA and miRNA microarrays were conducted, and the differentially expressed mRNAs and miRNAs were compared between the primary tumors and metastatic tumors from the same patients. As the mRNAs were inversely correlated with the regulating miRNAs, we identified mutually complementary mRNA-miRNA pairs enriched either in the primary tumors or metastatic tumors from the same patients. We empirically allocated miRNAs enriched in primary tumors relative to metastatic tumors and identified miR-766 as a metastasis-suppressive miRNA.
In this study, we evaluated the functional capacity of miR-766, which was discovered by comparing a primary and a metastatic PDX model of breast cancer [
13]. miR-766-overexpressed MDA-MB-231 triple-negative breast cancer cells exhibited similar primary tumor growth in highly immunocompromised NSG mice compared to vector-transfected control cells. In contrast, tumor sphere formation was significantly decreased in miR-766-overexpressed MDA-MB-231 cells compared with control cancer cells. In addition, miR-766-overexpressed MDA-MB-231 cells showed reduced invasion into the Matrigel in the invasion assay and decreased migration in the wound migration assay (data not shown) compared with control cells. The effector phase of hematogenous metastasis was evaluated via intravenous injection of breast cancer cells. Although primary tumor growth in the mammary fat pads was similar between the cell types, lung metastasis was dramatically reduced in miR-766-overexpressed MDA-MB-231 cells compared with control cells. Thus, miR-766 affected the distant metastasis process to a greater extent than cancer cell proliferation and primary tumor growth.
The evaluation of putative molecular targets of miR-766 would be more informative and subsequently predict the on- and off-target effects of miR-766-based therapies. In addition to putative molecular targets for metastasis control, cellular targets that could be affected by miR-766 need to be mentioned. The aggressiveness and behavior of metastatic cancer cells are affected continuously by their neighboring partners, such as endothelial cells and fibroblasts [
22]. In addition, a series of innate and adaptive inflammatory and immune cells, which are equipped with a variety of enzymes and toxic chemicals, can be co-opted by cancer cells in masquerading into the surrounding tissue and vessels, leading to distant metastasis [
2324]. Additionally, myeloid cells educated by cancer cells are strategically positioned in the putative metastatic organ as a pre-metastatic niche, making the local environment favorable for metastatic cancer cells [
25]. As miR-766 affects the distant lung metastasis of triple-negative MDA-MB-231 breast cancer cells, evaluating whether miR-766 affects key myeloid cell recruitment and activity in a remote organ, such as the lung, would be informative in dissecting the cellular mechanism of action.
The development of miRNA therapeutics includes small molecule inhibitors for key enzymes of miRNA processing. A more specific miRNA intervention includes specific miRNA-targeting technology. To this end, two key components are needed: chemical modification of miRNA to increase stability, such as the addition of a 2'-O-methyl group or a locked nucleic acid [
26]. In addition, several encapsulation methods have been developed to improve delivery to disease sites [
26]. By adopting up-to-date technology of an
in vivo cancer targeted delivery system, miR-766 may represent a future therapeutic target that effectively controls fatal breast cancer metastasis.
Go to :

Acknowledgments
This work was supported by the Seoul National University Hospital Research Fund (03-2014-0050 to D.S.L., 2014-1294), the Education and Research Encouragement Fund of Seoul National University Hospital and the National Research Foundation of Korea (NRF) grant funded by the Korea government (MSIP) (NRF-2014R1A2A1A11052904)
Go to :

Notes
Go to :

References
2. Polyak K. Breast cancer: origins and evolution. J Clin Invest. 2007; 117(11):3155–3163. PMID:
17975657.

3. Hornberger J, Alvarado MD, Rebecca C, Gutierrez HR, Yu TM, Gradishar WJ. Clinical validity/utility, change in practice patterns, and economic implications of risk stratifiers to predict outcomes for early-stage breast cancer: a systematic review. J Natl Cancer Inst. 2012; 104(14):1068–1079. PMID:
22767204.

4. Early Breast Cancer Trialists' Collaborative Group (EBCTCG). Effects of chemotherapy and hormonal therapy for early breast cancer on recurrence and 15-year survival: an overview of the randomised trials. Lancet. 2005; 365(9472):1687–1717. PMID:
15894097.
5. El Saghir NS, Tfayli A, Hatoum HA, Nachef Z, Dinh P, Awada A. Treatment of metastatic breast cancer: state-of-the-art, subtypes and perspectives. Crit Rev Oncol Hematol. 2011; 80(3):433–449. PMID:
21330148.

6. Eckhardt BL, Francis PA, Parker BS, Anderson RL. Strategies for the discovery and development of therapies for metastatic breast cancer. Nat Rev Drug Discov. 2012; 11(6):479–497. PMID:
22653217.

7. Oh K, Ko E, Kim HS, Park AK, Moon HG, Noh DY, Lee DS. Transglutaminase 2 facilitates the distant hematogenous metastasis of breast cancer by modulating interleukin-6 in cancer cells. Breast Cancer Res. 2011; 13(5):R96. PMID:
21967801.

8. Oh K, Lee OY, Shon SY, Nam O, Ryu PM, Seo MW, Lee DS. A mutual activation loop between breast cancer cells and myeloid-derived suppressor cells facilitates spontaneous metastasis through IL-6 trans-signaling in a murine model. Breast Cancer Res. 2013; 15(5):R79. PMID:
24021059.

9. Inui M, Martello G, Piccolo S. MicroRNA control of signal transduction. Nat Rev Mol Cell Biol. 2010; 11(4):252–263. PMID:
20216554.

10. Bartel DP. MicroRNAs: target recognition and regulatory functions. Cell. 2009; 136(2):215–233. PMID:
19167326.

11. Jonas S, Izaurralde E. Towards a molecular understanding of microRNA-mediated gene silencing. Nat Rev Genet. 2015; 16(7):421–433. PMID:
26077373.

12. Nicoloso MS, Spizzo R, Shimizu M, Rossi S, Calin GA. MicroRNAs--the micro steering wheel of tumour metastases. Nat Rev Cancer. 2009; 9(4):293–302. PMID:
19262572.
13. Moon HG, Oh K, Lee J, Lee M, Kim JY, Yoo TK, Seo MW, Park AK, Ryu HS, Jung EJ, Kim N, Jeong S, Han W, Lee DS, Noh DY. Prognostic and functional importance of the engraftment-associated genes in the patient-derived xenograft models of triple-negative breast cancers. Breast Cancer Res Treat. 2015; 154(1):13–22. PMID:
26438141.

14. Ito M, Hiramatsu H, Kobayashi K, Suzue K, Kawahata M, Hioki K, Ueyama Y, Koyanagi Y, Sugamura K, Tsuji K, Heike T, Nakahata T. NOD/SCID/gamma(c)(null) mouse: an excellent recipient mouse model for engraftment of human cells. Blood. 2002; 100(9):3175–3182. PMID:
12384415.
15. Perou CM, Sørlie T, Eisen MB, van de Rijn M, Jeffrey SS, Rees CA, Pollack JR, Ross DT, Johnsen H, Akslen LA, Fluge O, Pergamenschikov A, Williams C, Zhu SX, Lønning PE, Børresen-Dale AL, Brown PO, Botstein D. Molecular portraits of human breast tumours. Nature. 2000; 406(6797):747–752. PMID:
10963602.

16. Ahmad A. Pathways to breast cancer recurrence. ISRN Oncol. 2013; 2013:290568. PMID:
23533807.

17. Santos R, Ursu O, Gaulton A, Bento AP, Donadi RS, Bologa CG, Karlsson A, Al-Lazikani B, Hersey A, Oprea TI, Overington JP. A comprehensive map of molecular drug targets. Nat Rev Drug Discov. 2017; 16(1):19–34. PMID:
27910877.
18. Mimeault M, Batra SK. Altered gene products involved in the malignant reprogramming of cancer stem/progenitor cells and multitargeted therapies. Mol Aspects Med. 2014; 39:3–32. PMID:
23994756.

19. Gandellini P, Doldi V, Zaffaroni N. microRNAs as players and signals in the metastatic cascade: Implications for the development of novel anti-metastatic therapies. Semin Cancer Biol. 2017; 44:132–140. PMID:
28344166.

20. Rupaimoole R, Slack FJ. MicroRNA therapeutics: towards a new era for the management of cancer and other diseases. Nat Rev Drug Discov. 2017; 16(3):203–222. PMID:
28209991.

21. Teoh SL, Das S. The Role of MicroRNAs in Diagnosis, Prognosis, Metastasis and Resistant Cases in Breast Cancer. Curr Pharm Des. 2017; 23(12):1845–1859. PMID:
28231756.

22. Kalluri R. The biology and function of fibroblasts in cancer. Nat Rev Cancer. 2016; 16(9):582–598. PMID:
27550820.

23. Mantovani A, Marchesi F, Malesci A, Laghi L, Allavena P. Tumour-associated macrophages as treatment targets in oncology. Nat Rev Clin Oncol. 2017; 14(7):399–416. PMID:
28117416.

24. Gabrilovich DI, Ostrand-Rosenberg S, Bronte V. Coordinated regulation of myeloid cells by tumours. Nat Rev Immunol. 2012; 12(4):253–268. PMID:
22437938.

25. Peinado H, Zhang H, Matei IR, Costa-Silva B, Hoshino A, Rodrigues G, Psaila B, Kaplan RN, Bromberg JF, Kang Y, Bissell MJ, Cox TR, Giaccia AJ, Erler JT, Hiratsuka S, Ghajar CM, Lyden D. Pre-metastatic niches: organ-specific homes for metastases. Nat Rev Cancer. 2017; 17(5):302–317. PMID:
28303905.

26. Garzon R, Marcucci G, Croce CM. Targeting microRNAs in cancer: rationale, strategies and challenges. Nat Rev Drug Discov. 2010; 9(10):775–789. PMID:
20885409.

Go to :
