Abstract
Myelin degeneration is one of the characteristics of aging and degenerative diseases. This study investigated age-related alterations in expression of myelin basic protein (MBP) in the hippocampal subregions (dentate gyrus, CA2/3 and CA1 areas) of gerbils of various ages; young (1 month), adult (6 months) and aged (24 months), using western blot and immunohistochemistry. Western blot results showed tendencies of age-related reductions of MBP levels. MBP immunoreactivity was significantly decreased with age in synaptic sites of trisynaptic loops, perforant paths, mossy fibers, and Schaffer collaterals. In particular, MBP immunoreactive fibers in the dentate molecular cell layer (perforant path) was significantly reduced in adult and aged subjects. In addition, MBP immunoreactive mossy fibers in the dentate polymorphic layer and in the CA3 striatum radiatum was significantly decreased in the aged group. Furthermore, we observed similar age-related alterations in the CA1 stratum radiatum (Schaffer collaterals). However, the density of MBP immunoreactive fibers in the dentate granular cell layer and CA stratum pyramidale was decreased with aging. These findings indicate that expression of MBP is age-dependent and tissue specific according to hippocampal layers.
Myelin is a fatty white substance that is formed from oligodendrocytes and is an electrically insulating layer surrounding nerve axons in the central nervous system (CNS) [1]. Myelin basic protein (MBP) is one of key structural proteins of CNS myelin, accounting for 30% of total myelin protein [2]. MBP is considered a multifunctional protein that participates in scaffolding and signaling functions between oligodendrocyte and extracellular space as well as in forming and compacting myelin sheath in the CNS [34].
The hippocampus is a part of the limbic system and is critical for learning and memory [5]. Sequential neuronal circuitry within the ipsilateral hippocampus is classified as a trisynaptic loop. The circuit begins from neurons in the entorhinal cortex and projects to granule cells of the dentate gyrus through the perforant path, and then continues to the CA3 pyramidal cells through the mossy fibers, finally connecting with CA1 pyramidal cells through Schaffer collaterals [6]. In addition, CA3 and CA1 pyramidal cells are also connected with contralateral pyramidal cells through commissural connections [6].
It has been reported that aging contributes to a loss in hippocampal neurons and volume and that these changes are closely related to impairments of cognition [7]. However, investigations regarding effects of aging on myelin proteins in the hippocampus are limited. The majority of studies on myelin changes during aging have focused on alterations of subcortical white matter in normal brains [89], and brains with neurological diseases, such as Alzheimer's disease [1011] or schizophrenia [1213].
Therefore, the present study investigated the patterns in distribution and alteration of MBP immunoreactivity in the hippocampus during normal aging in gerbils which are good biological models for normal aging [14].
Male gerbils (Meriones unguiculatus) were used at postnatal month (PM) 1, PM 6 and PM 24 as the young, adult and aged, respectively. Gerbils (n=14 in each group) were handled and cared following the guidelines of current international laws and policies (NIH Guide for the Care and Use of Laboratory Animals, The National Academies Press, 8th Ed., 2011). Our experimental protocol was approved by Institutional Animal Care and Use Committee (IACUC) of Kangwon National University (approval no. KW-160802-2).
Animals (n=7 in each group) were used to examine change in MBP levels. Western blot analysis was performed according to our published method [15]. In short, hippocampal tissues were homogenized and centrifuged, the supernatants were subjected to western blot analysis. Rabbit anti-MBP (1:1000, Abcam, Cambridge, UK) was used as primary antibodies. Results of western blot analysis were scanned, and densitometric analysis for the quantification of the bands was done using Image J 1.46 (National Institutes of Health) to count relative optical density (ROD): A ratio of the ROD was calibrated as %, with young group designated as 100%.
To examine age-related changes in NeuN and MBP immunoreactivity in the hippocampus during normal aging, immunohistochemical staining and quantitative analysis of immunohistochemical data were performed according to our method [161715]. Shortly, animals (n=7 in each group) were anesthetized with pentobarbital sodium and perfused transcardially with 4% paraformaldehyde. Brain tissues were sectioned into at 30-µm thickness. Mouse anti-NeuN (1:800, Millipore, Ontario, Canada) and rabbit anti-MBP (1:1000, Abcam, Cambridge, UK) were used as primary antibodies. A negative control test was carried out using pre-immune serum instead of primary antibody to establish the specificity of the immunostaining. The negative control resulted in the absence of immunoreactivity in any structures.
To quantitatively analyze the immunoreactivities, digital images of the corresponding hippocampal areas from 6 sections per animal were captured with an AxioM1 light microscope (Carl Zeiss, Germany) equipped with a digital camera (Axiocam, Carl Zeiss) connected to a PC monitor. First, NeuN immunoreactive neurons were counted in a 250×250 µm square, applied approximately at the center of the CA1, CA2/3 region and DG using an image analyzing system (software: Optimas 6.5, CyberMetrics, Scottsdale, AZ). Cell counts were obtained by averaging the counts from each animal. Second, the densities of all MBP immunoreactive structures were evaluated based on optical density (OD), which was obtained after the transformation of the mean gray level using the formula: OD=log (256/mean gray level). After the background was subtracted, a ratio of the OD of image file was calibrated as % (relative optical density, ROD) using Adobe Photoshop version 8.0 and NIH Image J software (National Institutes of Health, Bethesda, MD). The mean value of the OD of the young group was designated as 100%, and the ROD of each group was calibrated and expressed as % of the young group.
The data shown here represent the means±SEM. Differences of the means among the groups were statistically analyzed by analysis of variance (ANOVA) with a post hoc Bonferroni's multiple comparison test to elucidate age-related differences among groups. Statistical significance was considered at P<0.05.
In all the groups, most of NeuN-immunoreactive neurons were distributed in the granular cell layer in the dentate gyrus, and in the striatum pyramidale in the hippocampus proper (CA1-3 areas) (Figure 1). The distribution pattern of NeuN-immunoreactive cells was not changed with age (Figure 1A-1C, 1A1-1C3). In addition, the number of NeuN-immunoreactive cells in the dentate gyrus and hippocampus proper was not significantly different among the groups (Figure 1D).
The western blot results showed that MBP level in the hippocampus was significantly declined with age during normal aging; the MBP level was significantly decreased in the aged group compared to that in the young group (Figure 2).
MBP immunoreactivity in the dentate gyrus was generally decreased with age; however, MBP immunoreactivity in the CA1-3 regions was different according to layers (Figure 3).
The density of MBP immunoreactive fibers was generally low in the molecular layer and high in the polymorphic layer in all the groups (Figure 3A1-C1). In the molecular layer, which contains perforant path, the density of MBP immunoreactive fibers was significantly decreased by 48 and 60% in the adult and aged, respectively, compared with that in the young group (Figure 3B1, 3C1, 3D). In the granular cell layer, MBP immunoreactive fibers were also decreased with age (Figure 3B1, 3C1, 3D). In the polymorphic layer, the density of MBP immunoreactive fibers in the young group was highest among all layers (Figure 3A1). The density in the adult group was significantly decreased by 56 and 67% in the adult and aged, respectively, compared with that in the young group (Figure 3B1, 3C1, 3D).
In the stratum oriens, the density of MBP immunoreactive myelinated fibers was not significantly different among all the groups (Figure 3A2-3D). In the stratum pyramidale, the density of MBP immunoreactive fibers was generally highest (181% of the young group) in the adult group (Figure 3B2, 3D), and slightly decreased in the aged group (Figure 3C2, 3D). In the stratum lucidum, the density of MBP immunoreactive fibers was generally low in all the groups (Figure 3A1-3C2). In the stratum radiatum, which contains mossy fibers, the density of MBP immunoreactive fibers in the adult and aged group was reduced by 13% and 39%, respectively, compared with that in the young group (Figure 3B2, 3C2, 3D).
The density of MBP immunoreactive myelinated fibers was generally high in the stratum pyramidale in all the groups (Figure 3A3-3C3). In the stratum oriens, the density of MBP immunoreactive fibers was significantly increased in the adult by 35% compared with that in the young group (Figure 3B3, 3D), and, in the aged group, the density was similar to that in the young group (Figure 3C3, 3D). In the stratum pyramidale, the density of MBP immunoreactive fibers was not significantly different among all the groups (Figure 3A3-3C3, 3D). In the stratum radiatum, which contains Schaffer collaterals, MBP immunoreactive myelinated fibers was abundantly found in the young group (Figure 3A3). The density of MBP immunoreactive fibers in the adult and aged was significantly decreased by 30% and 64%, respectively, compared with that in the young group (Figure 3B3, 3C3, 3D).
In the present study, we compared the changes in neuronal distribution and myelin expression in the hippocampus of young, adult, and aged gerbils using western blot and immunohistochemistry.
In this study, MBP protein levels were significantly reduced and correlated with normal aging. Also, MBP immunoreactivity was significantly reduced with age in specific layers; namely, the dentate molecular cell layer which carries axons of the perforant path, the dentate polymorphic layer and the CA3 stratum radiatum which contain the path of mossy fibers, and the CA1 stratum radiatum contains the Schaffer collaterals. Similar to the present study, Tanaka et al. (2005) reported that MBP immunoreactivity in the hippocampal CA1 subfield was significantly reduced in the senescence-accelerated mouse P8 [18], and MBP protein levels were significantly reduced in older rats [19]. On the other hand, the present study showed that no significant differences in MBP immunoreactivity were found in the CA3 stratum oriens which sends axons to contralateral CA1 pyramidal cells via the associational commissural pathway in all the groups. Based on our findings and previous studies, MBP immunoreactivity in the gerbil hippocampus progressively decreases within specific layers with age; however, MBP expression in the fibers going to the contralateral hippocampus were relatively more resistant to aging than fibers in the ipsilateral hippocampus.
Sloane et al. (2003) reported differences in age-related alterations of myelin proteins in the corpus callosum of aged rhesus monkey, a major white matter in the telencephalon but that MBP levels were not significantly different [20]. In addition, Xie et al. (2013) identified the overall age-related decline of MBP in the corpus callosum and in the dorsal column of the spinal cord in the aged rats [19]. Above findings may be associated with reports that show that myelinated nerve fibers are significantly lost and their myelin sheaths break down structurally with age in rhesus monkeys and humans, which displays the formation of myelin lamellae splits and myelin balloons in the gray and white matter [2122]. It is well known that myelin is relatively well preserved in the gray matter compared to that in the white matter; namely, age-dependent reduction of myelin sheaths of nerve fibers [2324] and myelin proteins [19] is much more obvious in the white matter. These results indicate that age-associated alterations of myelin proteins might occur differently according to specific brain regions and gray/white matter.
Finally, we show in the present study that neuronal morphology and distribution patterns were not significantly changed in the hippocampus in all the groups, although numbers of NeuN positive neurons were slightly decreased in the whole hippocampus with age, which coincides with previous studies [2526]. Despite the preservation of neuron numbers, age-related defects of myelin lead to reduction in the conduction velocity of nerve fibers and diminution in neural connectivity [2728], and there is a significant correlation between myelin degeneration and cognitive deficits [2923]. Results of previous studies support the present findings that showed that MBP expression was age-dependent and decreased over time without neuronal loss in the hippocampus, which underscore age-related cognitive declines with advanced age.
In summary, the present study identified age-related declines in MBP expression in the gerbil hippocampus as an indicator of aging; in particular, MBP immunoreactivity was significantly reduced in the trisynaptic loop.
Acknowledgments
The authors would like to thank Mr. Seung Uk Lee for their technical help. This research was supported by the Bio-Synergy Research Project (NRF-2015M3A9C 4076322) of the Ministry of Science, ICT and Future Planning through the National Research Foundation, and by a Priority Research Centers Program grant (NRF-2009-0093812) through the National Research Foundation of Korea funded by the Ministry of Science, ICT and Future Planning.
References
1. Baumann N, Pham-Dinh D. Biology of oligodendrocyte and myelin in the mammalian central nervous system. Physiol Rev. 2001; 81(2):871–927. PMID: 11274346.


2. Boggs JM. Myelin basic protein: a multifunctional protein. Cell Mol Life Sci. 2006; 63(17):1945–1961. PMID: 16794783.


3. Harauz G, Ladizhansky V, Boggs JM. Structural polymorphism and multifunctionality of myelin basic protein. Biochemistry. 2009; 48(34):8094–8104. PMID: 19642704.


4. Readhead C, Takasashi N, Shine HD, Saavedra R, Sidman R, Hood L. Role of myelin basic protein in the formation of central nervous system myelin. Ann N Y Acad Sci. 1990; 605:280–285. PMID: 1702601.


5. Neves G, Cooke SF, Bliss TV. Synaptic plasticity, memory and the hippocampus: a neural network approach to causality. Nat Rev Neurosci. 2008; 9(1):65–75. PMID: 18094707.


6. Neves G, Cooke SF, Bliss TV. Synaptic plasticity, memory and the hippocampus: a neural network approach to causality. Nat Rev Neurosci. 2008; 9(1):65–75. PMID: 18094707.


7. Schuff N, Amend DL, Knowlton R, Norman D, Fein G, Weiner MW. Age-related metabolite changes and volume loss in the hippocampus by magnetic resonance spectroscopy and imaging. Neurobiol Aging. 1999; 20(3):279–285. PMID: 10588575.


8. Feldman ML, Peters A. Ballooning of myelin sheaths in normally aged macaques. J Neurocytol. 1998; 27(8):605–614. PMID: 10405027.
9. Peters A. The effects of normal aging on myelin and nerve fibers: a review. J Neurocytol. 2002; 31(8-9):581–593. PMID: 14501200.
10. Bartzokis G. Age-related myelin breakdown: a developmental model of cognitive decline and Alzheimer's disease. Neurobiol Aging. 2004; 25(1):5–18. PMID: 14675724.


11. Wang DS, Bennett DA, Mufson EJ, Mattila P, Cochran E, Dickson DW. Contribution of changes in ubiquitin and myelin basic protein to age-related cognitive decline. Neurosci Res. 2004; 48(1):93–100. PMID: 14687885.


12. Chambers JS, Perrone-Bizzozero NI. Altered myelination of the hippocampal formation in subjects with schizophrenia and bipolar disorder. Neurochem Res. 2004; 29(12):2293–2302. PMID: 15672553.


13. Davis KL, Stewart DG, Friedman JI, Buchsbaum M, Harvey PD, Hof PR, Buxbaum J, Haroutunian V. White matter changes in schizophrenia: evidence for myelin-related dysfunction. Arch Gen Psychiatry. 2003; 60(5):443–456. PMID: 12742865.
14. Vanhooren V, Libert C. The mouse as a model organism in aging research: usefulness, pitfalls and possibilities. Ageing Res Rev. 2013; 12(1):8–21. PMID: 22543101.


15. Lee CH, Ahn JH, Park JH, Yan BC, Kim IH, Lee DH, Cho JH, Chen BH, Lee JC, Cho JH, Lee YL, Won MH, Kang IJ. Decreased insulin-like growth factor-I and its receptor expression in the hippocampus and somatosensory cortex of the aged mouse. Neurochem Res. 2014; 39(4):770–776. PMID: 24577837.
16. Ahn JH, Chen BH, Shin BN, Cho JH, Kim IH, Park JH, Lee JC, Tae HJ, Lee YL, Lee J, Byun K, Jeong GB, Lee B, Kim SU, Kim YM, Won MH, Choi SY. Intravenously Infused F3.Olig2 Improves Memory Deficits via Restoring Myelination in the Aged Hippocampus Following Experimental Ischemic Stroke. Cell Transplant. 2016; 25(12):2129–2144. PMID: 27442084.


17. Ahn JH, Choi JH, Park JH, Kim IH, Cho JH, Lee JC, Koo HM, Hwangbo G, Yoo KY, Lee CH, Hwang IK, Cho JH, Choi SY, Kwon YG, Kim YM, Kang IJ, Won MH. Long-Term Exercise Improves Memory Deficits via Restoration of Myelin and Microvessel Damage, and Enhancement of Neurogenesis in the Aged Gerbil Hippocampus After Ischemic Stroke. Neurorehabil Neural Repair. 2016; 30(9):894–905. PMID: 27026692.


18. Tanaka J, Okuma Y, Tomobe K, Nomura Y. The age-related degeneration of oligodendrocytes in the hippocampus of the senescence-accelerated mouse (SAM) P8: a quantitative immunohistochemical study. Biol Pharm Bull. 2005; 28(4):615–618. PMID: 15802797.


19. Xie F, Zhang JC, Fu H, Chen J. Age-related decline of myelin proteins is highly correlated with activation of astrocytes and microglia in the rat CNS. Int J Mol Med. 2013; 32(5):1021–1028. PMID: 24026164.


20. Sloane JA, Hinman JD, Lubonia M, Hollander W, Abraham CR. Age-dependent myelin degeneration and proteolysis of oligodendrocyte proteins is associated with the activation of calpain-1 in the rhesus monkey. J Neurochem. 2003; 84(1):157–168. PMID: 12485412.


21. Bowley MP, Cabral H, Rosene DL, Peters A. Age changes in myelinated nerve fibers of the cingulate bundle and corpus callosum in the rhesus monkey. J Comp Neurol. 2010; 518(15):3046–3064. PMID: 20533359.


22. Marner L, Nyengaard JR, Tang Y, Pakkenberg B. Marked loss of myelinated nerve fibers in the human brain with age. J Comp Neurol. 2003; 462(2):144–152. PMID: 12794739.


23. Peters A, Moss MB, Sethares C. Effects of aging on myelinated nerve fibers in monkey primary visual cortex. J Comp Neurol. 2000; 419(3):364–376. PMID: 10723011.


24. Peters A, Sethares C. Aging and the myelinated fibers in prefrontal cortex and corpus callosum of the monkey. J Comp Neurol. 2002; 442(3):277–291. PMID: 11774342.


25. Ahn JH, Chen BH, Shin BN, Lee TK, Cho JH, Kim IH, Park JH, Lee JC, Tae HJ, Lee CH, Won MH, Lee YL, Choi SY, Hong S. Comparison of catalase immunoreactivity in the hippocampus between young, adult and aged mice and rats. Mol Med Rep. 2016; 14(1):851–856. PMID: 27221506.


26. Hwang IK, Yoo KY, Jung BK, Cho JH, Kim DH, Kang TC, Kwon YG, Kim YS, Won MH. Correlations between neuronal loss, decrease of memory, and decrease expression of brain-derived neurotrophic factor in the gerbil hippocampus during normal aging. Exp Neurol. 2006; 201(1):75–83. PMID: 16678162.


27. Morales FR, Boxer PA, Fung SJ, Chase MH. Basic electrophysiological properties of spinal cord motoneurons during old age in the cat. J Neurophysiol. 1987; 58(1):180–194. PMID: 3612223.


28. Xi MC, Liu RH, Engelhardt JK, Morales FR, Chase MH. Changes in the axonal conduction velocity of pyramidal tract neurons in the aged cat. Neuroscience. 1999; 92(1):219–225. PMID: 10392844.


29. Bartzokis G, Lu PH, Geschwind DH, Edwards N, Mintz J, Cummings JL. Apolipoprotein E genotype and age-related myelin breakdown in healthy individuals: implications for cognitive decline and dementia. Arch Gen Psychiatry. 2006; 63(1):63–72. PMID: 16389198.
Figure 1
NeuN immunohistochemistry in the hippocampus of young (A), adult (B), and aged (C) gerbils. Numbers of NeuN immunoreactive neurons are not significantly different among all the groups. GCL, granular cell layer; MoL, molecular cell layer; PoL, polymorphic cell layer; SL, stratum lucidum; SO, stratum oriens; SP, stratum pyramidale. Scale bars=400 (A-C), 100 (A1-C3) µm. D: The relative number of NeuN immunoreactive neurons in the dentate gyrus, CA2/3, and CA1 region (n=7 per group). The bars indicate the means±SEM.
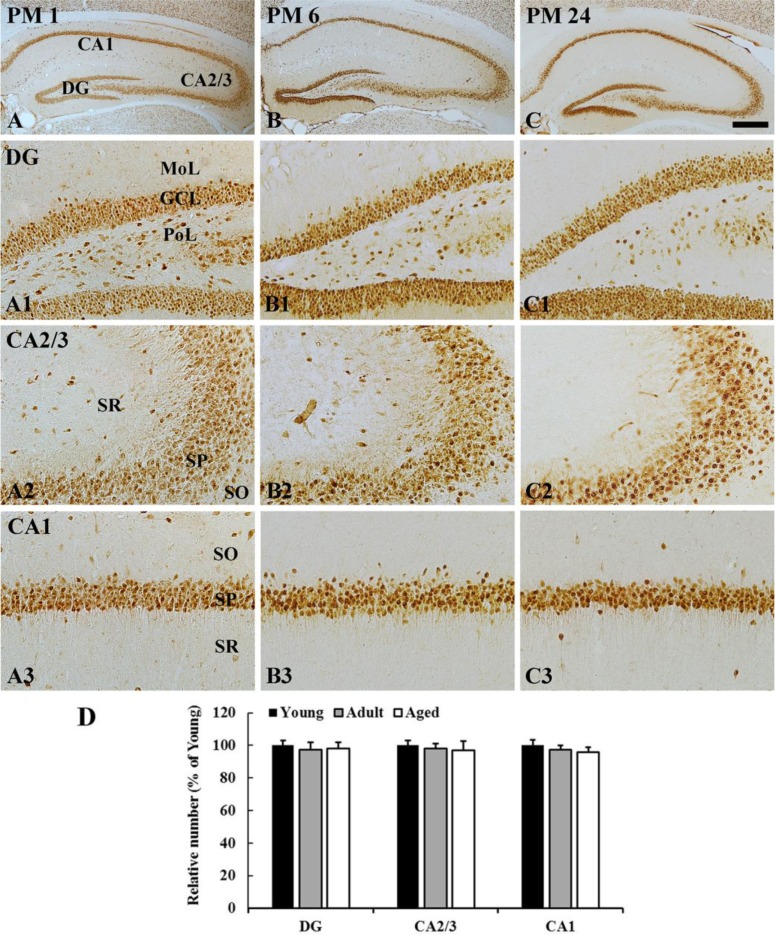
Figure 2
Western blot analysis of MBP in the hippocampus of young, adult and aged gerbils. The relative optical density (ROD) as percentage of immunoblot band is presented (n=7 per group; *P<0.05: significantly different from the young group, †P<0.05, significantly different from the adult group). The bars indicate mean±SEM.
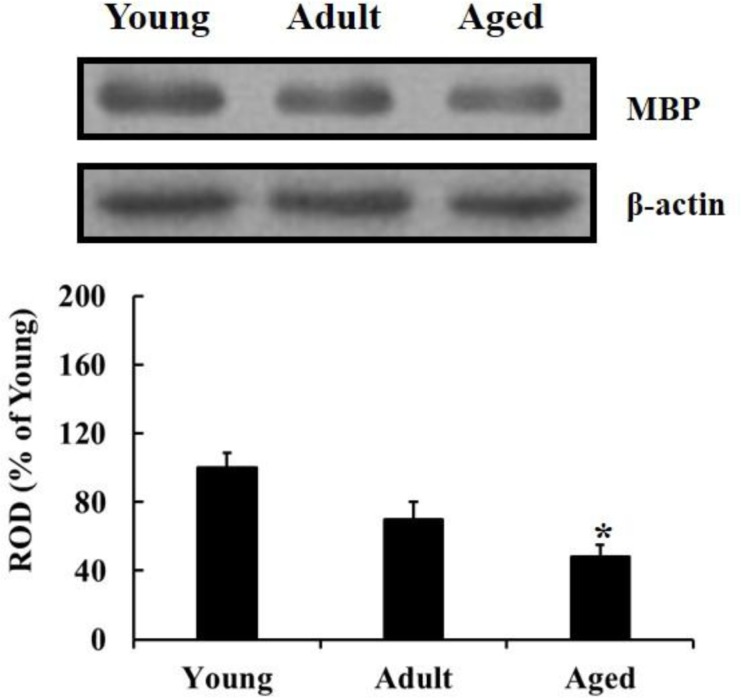
Figure 3
MBP immunohistochemistry in the hippocampus of young (A), adult (B), and aged (C) gerbils. In the dentate gyrus, the density of MBP immunoreactive fibers is decreased in all layers with age. In the CA2/3 and CA1 regions, the density of MBP immunoreactive fibers is significantly reduced only in the stratum radiatum (SR) with age. GCL, granular cell layer; MoL, molecular cell layer; PoL polymorphic layer; SL, stratum lucidum; SO, stratum oriens; SP, stratum pyramidale. Scale bars=400 (A-C), 50 (A1-C3) µm. D: The relative optical density (ROD) of MBP immunoreactive fibers in the hippocampal subregions (n=7 per group; *P<0.05, significantly different from the young group, †P<0.05, significantly different from the adult group). The bars indicate the means±SEM.
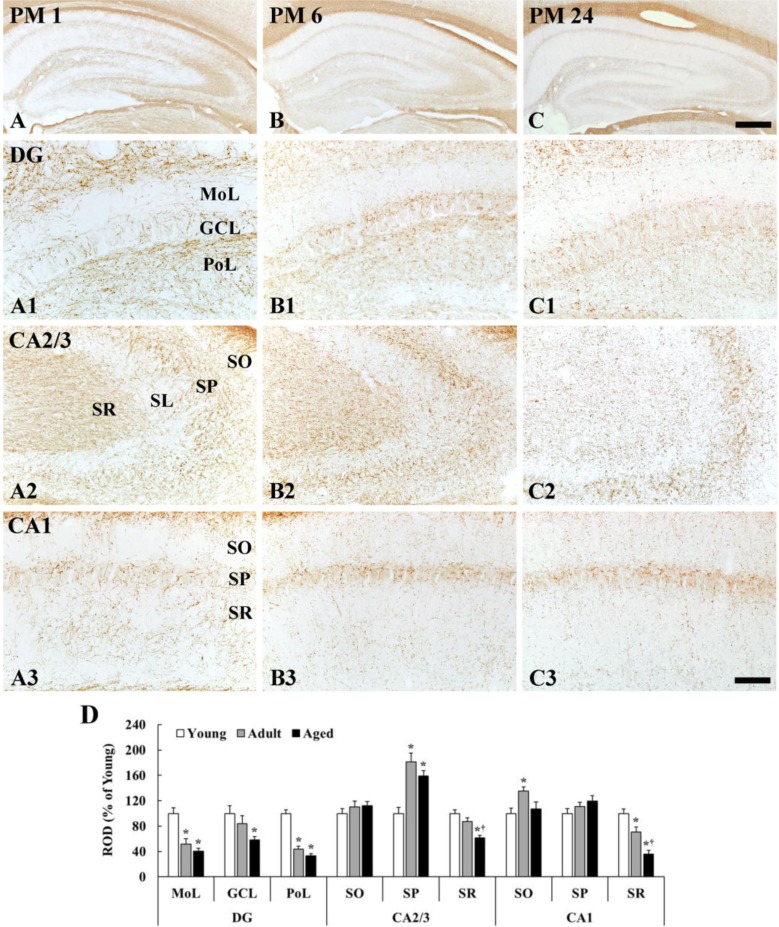