This article has been corrected. See "Erratum: Comparison of doxorubicin-induced cardiotoxicity in the ICR mice of different sources" in Volume 33 on page 319.
Abstract
Doxorubicin is a widely used chemotherapeutic agents and is now part of standard therapeutic regimens for a variety of cancers (eg, hematopoietic malignancies and advanced solid tumors of the breast, ovary, thyroid, and bone). However, a potentially lethal and dose-dependent cardiotoxicity that appears within a short time after treatment limits the usage of doxorubicin in cancer patients. Although the mechanism of doxorubicin-induced cardiotoxicity is not completely understood, it is thought that free radical-induced oxidative stress and excessive production of reactive oxygen species are primary drivers of its toxicity. In this study, we compared the doxorubicin-induced cardiotoxicity of ICR mice obtained from three different sources and evaluated the utility of Korl:ICR stock established by the Korean FDA. Because doxorubicin-induced cardiotoxicity is thought to involve the excessive generation of ROS followed by oxidative stress, we determined the representative tissue index of oxidation, lipid peroxidation, and antioxidant, glutathione (GSH), as well as the parameters of heart injury. Doxorubicin treatment successfully induced cardiotoxicity as evidenced by histological examination and serum parameters (eg, levels of LDH and CK activities) in ICR mice. It was accompanied by increased lipid peroxidation and a decrease in both cysteine and GSH, further supporting previous reports that oxidative stress is a potential mechanism of doxorubicin-induced cardiotoxicity. Of interest, we did not observe a significant difference in doxorubicin-induced cardiotoxicity among mice of different origins. Collectively, our results suggest that Korl:ICR strain may be useful in the research of doxorubicin-induced cardiotoxicity.
The development of more effective anticancer drugs has greatly increased overall survival and has significantly reduced mortality rates that are accompanied by great benefits relating to patient prognosis. In many cases, a leading cause of morbidity and mortality in patients receiving chemotherapy has been cardiotoxicity. Patients who develop heart failure due to cancer chemotherapy have a 2-year mortality rate of 60% [1]. Consequently, cardiotoxicity caused by chemotherapies has been an area of interest to many clinical researchers [2]. Cardiotoxicity can be indicated by various pathological signs such as arrhythmia, changes in blood pressure, myocardial ischemia, or necrosis, but most importantly it can result in heart failure. In general, the onset of cardiac dysfunction is known to occur immediately after the administration of anticancer drugs for up to several years [34]. Therefore, animal studies in the preclinical evaluation are essential to assess cardiotoxicity induced by anticancer drugs such as doxorubicin.
Doxorubicin is a widely used chemotherapeutic agent and now part of standard therapeutic regimens for a variety of cancers (eg, hematopoietic malignancies and advanced solid tumors of the breast, ovary, thyroid, and bone) [5]. However, a potentially lethal and dose-dependent cardiotoxicity that appears within a short time after treatment limits the usage of doxorubicin in cancer patients [67]. Although the mechanism of doxorubicin-induced cardiotoxicity is not completely understood, it has been considered that free radical-induced oxidative stress and excessive production of reactive oxygen species are primary drivers of its toxicity [8910]. Recent findings have shown that markedly enhanced electron transfer in the heart following doxorubicin treatment leads to a profound increase in superoxide anion and hydrogen peroxide formation in mitochondria and sarcoplasmic reticulum-two major sites of cardiac injury from doxorubicin [10111213]. Moreover, its cytotoxic potential may be magnified by a high density of mitochondria and lower levels of enzymatic capacity to detoxify ROS [89].
Outbred stocks of mice and rats are commonly used in academic and industrial research. ICR mice specifically are a vigorously outbred stock. These mice are Swiss in origin and used as a general-purpose stock and in pharmaceutical research to study genetics, oncology, toxicology, and pharmacology. The ICR mice originated from the albino non-inbred mice in Switzerland, imported to the Rockefeller Institute by Clara J. Lynch in 1926 [14]. They were distributed worldwide and, currently, a number of vendors produce their own commercially available ICR stocks. Therefore, understanding the characteristics of these stocks and their advantages and disadvantages is important as it may help guide experimental design.
In this study, we compared the doxorubicin-induced cardiotoxicity of ICR mice obtained from three different sources and evaluated the utility of Korl:ICR stock established by the Korean FDA. Because doxorubicin-induced cardiotoxicity is suggested to involve the excessive generation of ROS followed by causing oxidative stress, we determined the representative tissue index of oxidation, lipid peroxidation, and antioxidant, glutathione (GSH), as well as the parameters of heart injury.
Male ICR mice were obtained from three different sources. Korl:ICR were kindly provided by the Department of Laboratory Animal Resources in the National Institute of Food and Drug Safety Evaluation (NIFDS, Cheongju, Korea). The other two groups of ICR stocks were purchased from different vendors located in the United States (referred A: ICR) and Japan (referred B: ICR). The use of animals was in compliance with the guidelines established and approved by the Institutional Animal Care and Use Committee in Pusan National University (PNU-2016-1236). Animals were acclimated to temperature (22±2℃) and humidity (55±5%) controlled rooms with a 12-h light/dark cycle for 1 week prior to use. All mice were provided with ad libitum access to standard irradiated chow diet (Samtako Inc., Osan, Korea) consisting of moisture (12.5%), crude protein (25.43%), crude fat (6.06%), crude fiber (3.9%), crude ash (5.31%), calcium (1.14%), and phosphorus (0.99%) and water. Alterations in body weight were measured using an electronic balance (Mettler Toledo, Greifensee, Switzerland) once a week according to the MFDS guideline. Doxorubicin-treated mice received a single intraperitoneal injection of 20 mg/kg of doxorubicin (Sigma Aldrich, St. Louis, MO, USA) and samples were collected 4 days after doxorubicin administration
A blood sample was collected from the abdominal aorta of each mouse and transferred into a BD Microtainer Blood Collection Tube (BD Life Sciences, Franklin Lakes, NJ, USA).
The samples were centrifuged at 3000 g for 15 min to separate the sera, then stored at −80℃ for biochemical analyses. Serum activity of LDH was examined according to the manufacturer's instructions using commercially available kit obtained from DOGEN (Seoul, Korea). The CK-MB assay kit was purchased from BioVision (San Francisco, CA, USA) and used according to the manufacturer's instructions. Activities of both LDH and CK-MB were quantified spectrophotometrically at 450 nm using an MULTISKAN GO reader (Thermo Scientific, Waltham, MA, USA).
Tissues were fixed with 4% paraformaldehyde, embedded in paraffin, and sectioned into 5 µm thick slices for histological examination. The paraffin sections were deparaffinized, and subjected to hydrophilic treatment by sequential immersion in a series of 100, 95, 80, and 70% ethanol followed by deionized water. In hematoxylin and eosin (H&E) staining, the nucleus was colored blue with Mayer's hematoxylin, while the cytoplasm was colored red with eosin (Sigma Aldrich). The stained sections were observed under an inverted microscope (Olympus, Tokyo, Japan).
Hearts were homogenized in a threefold volume of cold 1.15% KCl. An aliquot of lysate was mixed with 0.2% thiobarbituric acid in 2M sodium acetate buffer containing 5% butylated hydroxytoluene. The mixtures were incubated at 95℃ for 45 min. The supernatant was injected into HPLC equipped with a fluorescence detector (FLD-3100; Thermo Scientific, Sunnyvale, CA, USA) and a 5 mm Symmetry C18 reversed phase column (4.6 mm×150 mm; Eka Chemicals, Bohus, Sweden). The mobile phase was composed of 35% methanol and 65% sodium phosphate buffer. The complex of MDA-thiobarbituric acid was monitored by fluorescence detection with excitation at 515 nm and emission at 553 nm.
Total cysteine and GSH were analyzed using the sample-derivatization method by 7-benzo-2-oxa-1,3-diazole-4-sulfonic acid (SBD-F) [15]. Hearts were homogenized in a four-fold volume of ice-cold 1M perchloric acid. The denatured protein was removed by centrifugation at 10,000 g for 10 min and the supernatant was assayed for total cysteine and GSH concentrations using a HPLC separation/fluorometric detection method. 50 mL of N-(2-Mercaptopropionyl) glycine, as an internal standard, was added to 50 mL of samples and briefly mixed. Following the addition of 10 mL of a 10% (w/v) tris(2-carboxyethyl)phosphine hydrochloride solution. Subsequently, 90 mL of trichloroacetic acid solution with 1 mM EDTA was added to each sample and centrifuged (13,000×g, 10 min). The supernatant (50 mL) was then added to another tube containing 10 mL of 1.55 M NaOH, 125 mL of 0.125M borate buffer (pH 9.5) with 4 mM EDTA, and 50 mL of 0.1% (w/v) SBD-F in borate buffer (0.125 M with 4 mM EDTA). Samples were incubated at 60℃ for 1 h, and then, injected into the HPLC with a fluorescence detector (excitation 385 nm and emission 515 nm). The chromatographic separation was performed using a Hector M-C18 column (3 mm×4.6 mm×150 mm) (Chiral Technology Korea, Daejeon, Korea).
To quantify doxorubicin-induced cardiotoxicity, we examined the serum markers, LDH and CK-MB, for myocardial injury. Both LDH (Figure 1A) and CK-MB (Figure 1B) were significantly elevated in the doxorubicintreated mice as compared with corresponding control mice. The levels of LDH and CK in doxorubicin-treated mice were not significantly different between the three different sources of mice.
In line with serum parameters of doxorubicin-treated mice, examinations of heart sections using light microscopy 96 h after doxorubicin treatment revealed loss of myofibril and striations, as well as cytoplasmic vacuolization as shown by H&E staining in Figure 2B, 2D, 2F compared with corresponding control (Figure 2A, 2C, 2E). There was no significant difference in the comparison of the three different sources.
Oxidative stress is considered to be the major cause of cardiotoxicity induced by doxorubicin and the accumulation of doxorubicin-induced reactive oxygen species in the heart may explain the cardiac lipid membrane peroxidation [16]. Thus, we further assessed lipid peroxidation in the heart using MDA levels. Figure 3 shows the effect of the doxorubicin treatment on the levels of MDA in the heart homogenates. Acute treatment of doxorubicin in the mice of three different sources dramatically increased MDA levels with no significant difference among sources observed.
GSH is the most abundant endogenous antioxidant and has a potential role defending against oxidative stress through its electrophilic-scavenging ability [17]. Thus it is considered that GSH level is an important determinant of antioxidant capacity in the tissue. Its biosynthesis is tightly regulated by the availability of substrate, cysteine, as well as the activity of g-glutamylcysteine ligase, a rate-limiting enzyme in the synthesis of GSH [18].
It has been suggested that the generation of oxygen radicals by doxorubicin-related redox cycle could explain depleted GSH pools in the heart that have been found after doxorubicin administration [1920]. Figure 4 shows that doxorubicin treatment significantly reduced the cysteine concentration (Figure 4A) and was accompanied by a decrease in levels of GSH (Figure 4B) in the heart, suggesting that low levels of GSH contributed partially to decrease in the ability to defense oxidative stress followed by enhancement of lipid peroxidation.
Experimental animal models have been widely used to study pathogenesis of human disease or to test the efficacy of potential therapeutic interventions. Moreover, such models have been adopted to determine toxicity and to explore the underlying molecular mechanisms of various diseases and conditions.
There are several reasons why toxicologists have been reluctant to use non-classical animal models in drug safety assessments. Firstly, an ideal animal model is one that closely imitates a human disease mechanistically, etiologically and pathologically-conditions that are not always met. Furthermore, incorporating a new animal model into an existing test system might produce new and unexpected results that do not accurately reflect the human phenomenon. Preclinical safety studies aim to examine dose-dependent risks of potential drug candidates, and to identify target organ toxicity and type of toxicity. These studies are usually carried out in highly standardized and well-characterized laboratory animals. Although animal models do not imitate human disease conditions in all respects, they have proven to be of value because there are clear limits and simplifications that correspond to the nature of the model.
The ICR stock, named “Korl:ICR” has been established and used for the last 50 years in the National Institute of Food and Drug Safety Evaluation (NIFDS) in Korea. Recently, it was proved that Korl:ICR is a genetically independent stock compared with other ICR stocks originated for Japan and USA, however, no biological differences have been observed [21]. In line with this, the purpose of present study was to characterize the Korl:ICR stock in terms of cardiotoxicity and determine its utility in evaluating the safety of preclinical drug candidates.
Of outbred mouse stocks, ICR has been often employed in cardiotoxicity research and it has been considered a good model of doxorubicin-induced cardiotoxicity to investigate its toxic mechanism and test the preventive effect of some compounds [222324]. Moreover, Ma et al. [25] suggested that ICR mice are more appropriate than inbred strain C57BL/6J which is highly susceptible and variable to large doses of doxorubicin in acute cardiotoxicity induced by doxorubicin.
There is increasing experimental evidence suggesting that the life-threatening effects of doxorubicin on the heart result from drug-induced, oxidative tissue injury [2627]. Doxorubicin-related reactive oxygen metabolism may overwhelm the limited capacity of heart to detoxify free radicals, resulting in extensive oxidative damage to myocardial cells [28]. In this study, doxorubicin treatment successfully induced cardiotoxicity as evidenced by histological examination and serum parameters (ie, LDH and CK-MB activities) in ICR mice. These effects were accompanied by increased lipid peroxidation and decreases in both cysteine and GSH, supporting previous reports that oxidative stress is potential mechanism of doxorubicin-induced cardiotoxicity. Of interest, we did not observe differences in doxorubicin-induced cardiotoxicity depending on the origin of the mice. Collectively, our results suggest that Korl:ICR stock may at least be useful in the research of doxorubicin-induced cardiotoxicity.
Acknowledgments
This project was supported by a grant of BIOREIN (Laboratory Animal Bio Resources Initiative) from Ministry of Food and Drug Safety in 2016.
References
1. Thavendiranathan P, Poulin F, Lim KD, Plana JC, Woo A, Marwick TH. Use of myocardial strain imaging by echocardiography for the early detection of cardiotoxicity in patients during and after cancer chemotherapy: a systematic review. J Am Coll Cardiol. 2014; 63(25 Pt A):2751–2768. PMID: 24703918.
2. Minotti G, Menna P, Salvatorelli E, Cairo G, Gianni L. Anthracyclines: molecular advances and pharmacologic developments in antitumor activity and cardiotoxicity. Pharmacol Rev. 2004; 56(2):185–229. PMID: 15169927.


3. Perrino C, Schiattarella GG, Magliulo F, Ilardi F, Carotenuto G, Gargiulo G, Serino F, Ferrone M, Scudiero F, Carbone A, Trimarco B, Esposito G. Cardiac side effects of chemotherapy: state of art and strategies for a correct management. Curr Vasc Pharmacol. 2014; 12(1):106–116. PMID: 22563720.


4. Raschi E, Vasina V, Ursino MG, Boriani G, Martoni A, De Ponti F. Anticancer drugs and cardiotoxicity: Insights and perspectives in the era of targeted therapy. Pharmacol Ther. 2010; 125(2):196–218. PMID: 19874849.


5. Carter SK. Adriamycin-Review. J Natl Cancer Inst. 1975; 55(6):1265–1274. PMID: 1107570.
6. Bristow MR, Mason JW, Billingham ME, Daniels JR. Doxorubicin Cardiomyopathy-Evaluation by Phonocardiography, Endomyocardial Biopsy, and Cardiac-Catheterization. Ann Intern Med. 1978; 88(2):168–175. PMID: 626445.
7. Bristow MR, Thompson PD, Martin RP, Mason JW, Billingham ME, Harrison DC. Early Anthracycline Cardiotoxicity. Am J Med. 1978; 65(5):823–832. PMID: 707541.


8. Maksimenko AV, Vavaev AV. Antioxidant enzymes as potential targets in cardioprotection and treatment of cardiovascular diseases. Enzyme antioxidants: the next stage of pharmacological counterwork to the oxidative stress. Heart Int. 2012; 7(1):e3. PMID: 22690296.


9. Todorova VK, Beggs ML, Delongchamp RR, Dhakal I, Makhoul I, Wei JY, Klimberg VS. Transcriptome profiling of peripheral blood cells identifies potential biomarkers for doxorubicin cardiotoxicity in a rat model. PLoS One. 2012; 7(11):e48398. PMID: 23209553.


10. Sterba M, Popelova O, Vavrova A, Jirkovsky E, Kovarikova P, Gersl V, Simunek T. Oxidative stress, redox signaling, and metal chelation in anthracycline cardiotoxicity and pharmacological cardioprotection. Antioxid Redox Signal. 2013; 18(8):899–929. PMID: 22794198.
11. Thayer WS. Adriamycin Stimulated Superoxide Formation in Sub-Mitochondrial Particles. Chem Biol Interact. 1977; 19(3):265–278. PMID: 202411.


12. Doroshow JH, Reeves J. Anthracycline-Enhanced Oxygen Radical Formation in the Heart. Proc Am Assoc Cancer Res. 1980; 21:266.
13. Carvalho FS, Burgeiro A, Garcia R, Moreno AJ, Carvalho RA, Oliveira PJ. Doxorubicin-induced cardiotoxicity: from bioenergetic failure and cell death to cardiomyopathy. Med Res Rev. 2014; 34(1):106–135. PMID: 23494977.


14. Chia R, Achilli F, Festing MF, Fisher EM. The origins and uses of mouse outbred stocks. Nat Genet. 2005; 37(11):1181–1186. PMID: 16254564.


15. Nolin TD, McMenamin ME, Himmelfarb J. Simultaneous determination of total homocysteine, cysteine, cysteinylglycine, and glutathione in human plasma by high-performance liquid chromatography: application to studies of oxidative stress. J Chromatogr B Analyt Technol Biomed Life Sci. 2007; 852(1-2):554–561.


16. Myers CE, McGuire WP, Liss RH, Ifrim I, Grotzinger K, Young RC. Adriamycin: the role of lipid peroxidation in cardiac toxicity and tumor response. Science. 1977; 197(4299):165–167. PMID: 877547.


18. Jung YS, Kim SJ, Kwon DY, Kim YC. Comparison of the effects of buthioninesulfoximine and phorone on the metabolism of sulfur-containing amino acids in rat liver. Biochem Biophys Res Commun. 2008; 368(4):913–918. PMID: 18275846.


19. Doroshow JH, Locker GY, Baldinger J, Myers CE. The effect of doxorubicin on hepatic and cardiac glutathione. Res Commun Chem Pathol Pharmacol. 1979; 26(2):285–295. PMID: 574979.
20. Indu R, Azhar TS, Nair A, Nair CK. Amelioration of doxorubicin induced cardio-and hepato-toxicity by carotenoids. J Cancer Res Ther. 2014; 10(1):62–67. PMID: 24762488.
21. Shin HJ, Cho YM, Shin HJ, Kim HD, Choi KM, Kim MG, Shin HD, Chung MW. Comparison of commonly used ICR stocks and the characterization of Korl:ICR. Lab Anim Res. 2017; 33(1):8–14. PMID: 28400834.


22. Tan G, Lou Z, Liao W, Zhu Z, Dong X, Zhang W, Li W, Chai Y. Potential biomarkers in mouse myocardium of doxorubicin-induced cardiomyopathy: a metabonomic method and its application. PLoS One. 2011; 6(11):e27683. PMID: 22110719.


23. Liu X, Chen Z, Chua CC, Ma YS, Youngberg GA, Hamdy R, Chua BH. Melatonin as an effective protector against doxorubicin-induced cardiotoxicity. Am J Physiol Heart Circ Physiol. 2002; 283(1):H254–H263. PMID: 12063298.
24. Fisher PW, Salloum F, Das A, Hyder H, Kukreja RC. Phosphodiesterase-5 inhibition with sildenafil attenuates cardiomyocyte apoptosis and left ventricular dysfunction in a chronic model of doxorubicin cardiotoxicity. Circulation. 2005; 111(13):1601–1610. PMID: 15811867.


25. Ma Y, Zhang X, Bao H, Mi S, Cai W, Yan H, Wang Q, Wang Z, Yan J, Fan GC. Toll-like receptor (TLR) 2 and TLR4 differentially regulate doxorubicin induced cardiomyopathy in mice. PLoS One. 2012; 7(7):e40763. PMID: 22808256.


26. Thayer WS. Adriamycin stimulated superoxide formation in submitochondrial particles. Chem Biol Interact. 1977; 19(3):265–278. PMID: 202411.


27. Doroshow JH, Locker GY, Ifrim I, Myers CE. Prevention of doxorubicin cardiac toxicity in the mouse by N-acetylcysteine. J Clin Invest. 1981; 68(4):1053–1064. PMID: 7287901.


28. Doroshow JH, Locker GY, Myers CE. Enzymatic defenses of the mouse heart against reactive oxygen metabolites: alterations produced by doxorubicin. J Clin Invest. 1980; 65(1):128–135. PMID: 7350193.
Figure 1
Effects of doxorubicin (DOX) treatment on LDH (A) and CK-MB (B) activities in the heart of ICR mice. **, *** Significantly different from the corresponding control mice (ANOVA followed by Newman-Keuls multiple range test, P<0.01, 0.001, respectively).
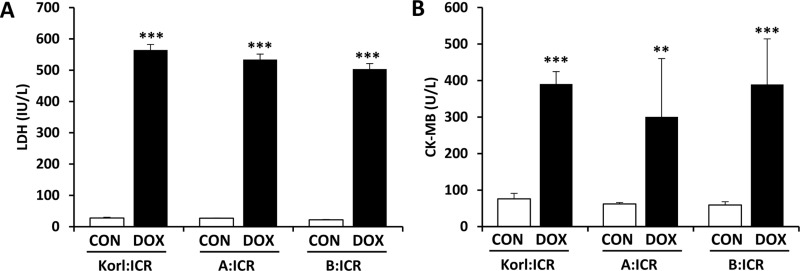
Figure 2
Histopathological changes in cardiac tissue following doxorubicin (DOX) treatment in ICR mice from three different sources. Pictures of heart sections from the control (A, C, and E) and DOX-treated (B, D, and F). Original magnification, ×100.
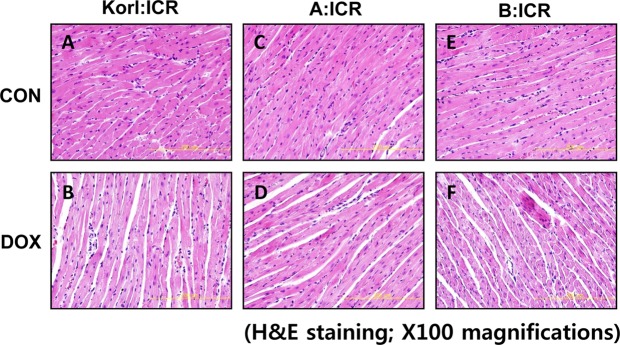