This article has been corrected. See "Erratum: Comparative study of fatty liver induced by methionine and choline-deficiency in C57BL/6N mice originating from three different sources" in Volume 33 on page 318.
Abstract
Non-alcoholic fatty liver disease (NAFLD) is believed to be the most prevalent liver disease worldwide and a major cause of chronic liver injury. It is characterized by lipid accumulation in the absence of significant alcohol consumption and frequently progresses to steatohepatitis, liver fibrosis, and hepatocellular carcinoma. Although many studies have been conducted to better understand NAFLD since it was first recognized, there are still many gaps in knowledge of etiology, prognosis, prevention and treatment. Methionine-choline deficient (MCD) diet, a well-established experimental model of NAFLD in rodents, rapidly and efficiently produces the clinical pathologies including macrovesicular steatosis and leads to disease progression. In this study, we measured the response to MCD diet in C57BL/6N mice obtained from three different sources; Korea NIFDS, USA, and Japan. We evaluated changes in body weight, food consumption, and relative weights of tissues such as liver, kidney, gonadal white adipose tissue, inguinal white adipose tissue, and brown adipose tissue. These basic parameters of mice with an MCD diet were not significantly different among the sources of mice tested. After 3 weeks on an MCD diet, histopathological analyses showed that the MCD diet induced clear fat vacuoles involving most area of the acinus in the liver of all mice. It was accompanied by increased serum activities of alanine aminotransferase and aspartate aminotransferase, and decreased levels of serum triglyceride and cholesterol. In conclusion, the response of C57BL6N mice originating from different sources to the MCD diet showed no significant differences as measured by physiological, biochemical, and histopathological parameters.
Non-alcoholic fatty liver disease (NAFLD) is believed to be the most prevalent liver disease worldwide and major reason for chronic liver injury [1]. It is characterized by lipid accumulation in the absence of significant alcohol consumption and frequently progresses to steatohepatitis, liver fibrosis, and hepatocellular carcinoma [2]. The main hypothesis of disease progression is considered to be the increased susceptibility to inflammatory cytokines and oxidative stress in fatty liver [345]. Although many studies have been done on NAFLD since the initial recognition of NAFLD, there are still many gaps related to our understanding of etiology, prognosis, prevention and treatment. Thus, well-established experimental animal models that mimic human pathology will provide a great opportunity to overcome genetic heterogeneity and various environmental factors influencing NAFLD.
There are several diet-induced models of NAFLD in small animals and, of them, mice are generally employed because of their short lifespan and the ease of gene manipulation. Currently, the C57BL/6 is the most widely used inbred mouse strain, and is commonly employed in the study of NAFLD [67]. The C57BL/6 inbred strain was established at the Jackson Laboratory as “subline 6” from the parental strain C57BL in 1948. One consequence of the popularity of the C57BL/6 strain has been the development of many substrains around the world. While all are referred to as C57BL/6, substrains are distinguished from one another by the series of letters (called lab codes) following the C57BL/6 designation. For example, C57BL/6 substrains established by commercial vendors such as Charles River, Taconic, Harlan and Jackson Labs are referred C57BL/6NCrl, C57BL/6NTac, C57BL/6NHsd and C57BL/6J, respectively. In the case of the Crl, Tac and Hsd substrains, the “N” indicates that these populations were originally raised at the National Institutes of Health (NIH). Thus, at the current time, C57BL/6J and C57BL/6N have been separated for around 220 generations. Although genetically very close, variations of the gene across generations will occur. In fact, these changes have resulted in phenotypic differences between C57BL/6 substrains [89101112].
To induce NAFLD, the methionine-choline deficient (MCD) diet is the most widely used in rodents. Although it does not induce characteristics of obesity due to weight loss, it is a very reproducible and consistently induces a spectrum of NAFLD depending on duration [1314]. Rodents fed an MCD diet rapidly develop the clinical pathologies from macrovesicular steatosis to hepatic fibrosis [15]. Choline is the substrate in the synthesis of phosphatidylcholine which is required for very low-density lipoprotein (VLDL) and its deficiency induce lipid accumulation in the liver [16]. Essential amino acids, methionine deficiency reduces the biosynthesis of glutathione (GSH) through transsulfuration pathway, followed by causing oxidative stress and contributing to disease progression [17].
The objective of this study was to compare the response to an MCD diet in the C57BL/6N mice obtained from three different sources; the National Institute of Food and Drug Safety Evaluation (NIFDS) in Korea, USA, and Japan. A study comparing the response to MCD diet could provide the utility of mice originated from Korea NIFDS in the preclinical test to develop new drug of NAFLD.
6-week-old male C57BL/6N mice were obtained from three different sources. C57BL/6NKorl were kindly provided by the Department of Laboratory Animal Resources in the National Institute of Food and Drug Safety Evaluation (NIFDS, Cheongju, Korea). The other two groups of C57BL/6N strain were purchased from different vendors located in the United States (referred A: C57BL/6N) and Japan (referred B: C57BL/6N). All animal protocols were approved by the Institutional Animal Care and Use Committees at Pusan National University (PNU-2015-0837). Mice were acclimated to temperature (22±2℃) and humidity (55±5%)-controlled rooms with a 12 h light/dark cycle for 1 week prior to use. They were randomly divided into 2 groups and fed a diet deficient in methionine and choline (MCD) or the control diet with methionine and choline for 3 weeks. The diets were obtained from Dyets Inc. (Bethlethem, PA, USA) and the composition of the diets was shown in Table 1. To assess the effect of MCD supplementation on various organs, liver and kidney as well as adipose tissue depots such as visceral (i.e., gonadal) and subcutaneous (i.e., inguinal) white adipose tissue (WAT), and inter-scapular brown adipose tissue (BAT) were harvested.
A blood sample was obtained from the abdominal aorta of each mouse and transferred into BD Microtainer Blood Collection Tube (BD Life Sciences, Franklin Lakes, NJ, USA). The samples were centrifuged at 3000 g for 15 min to separate the sera, which were stored at –80℃ for the biochemical analyses. Serum activities of alanine aminotransferase (ALT) and aspartate aminotransferase (AST) were measured using the protocol of Reitman and Frankel [18]. ALT and AST activities are proportional to the amount of pyruvate and oxaloacetate, respectively, formed over a definite period of time and are measured by a reaction with 2.4-dinitrophenylhydrazine in alkaline solution. They were quantified spectrophotometrically using an MULTISKAN GO reader (Thermo Scientific, Waltham, MA, USA). The serum levels of total cholesterol and triglycerides were determined by using an Automated Chemistry Analyzer (Prestige 24I, Tokyo Boeki Medical System, Tokyo, Japan).
To evaluate the levels of lipid accumulation in liver tissue, 5 µm cross sections of the left lateral lobe of the liver were sliced, immersed in propylene glycol for 5 min, and then stained with Oil red O (Sigma Aldrich). After washing with 85% propylene glycol and distilled water, the sections were counterstained with hematoxylin for 2 min before microscopic examination.
Daily observations for 3 weeks showed no change in dietary intake in the MCD diet-fed mice compared with control diet-fed mice (Figure 1A). However, mice fed the MCD diet lost body weight gradually compared with mice fed the control diet (Figure 1B). Otherwise, the general condition of MCD diet-fed mice remained good throughout the experimental period.
MCD-fed mice lost 31, 36, and 35% of their body weight in C57BL/6NKorl, A:C57BL/6N, and B:C57BL/6N, respectively, at the end of 3 weeks (Figure 2A). Although liver-to-body weight ratios in MCD diet-fed mice was slightly decreased with a similar degree in line with the previous finding [15], it showed significant reduction in both A:C57BL/6N and B:C57BL/6N, (Figure 2B). Metabolic interactions between liver and adipose tissue are known to be involved in the pathogenesis of NAFLD [1920]. In particular, hyper-lipolysis of WAT can contribute to triglyceride accumulation in livers in an MCD diet-induced NAFLD model [21]. In line with this, we examined the mass changes in adipose tissue depots in mice fed MCD diet. Although we only observed a significant decrease in the gonadal WAT of MCD-fed B:C57BL/6N mice, the relative weight of gonadal and inguinal WAT to body weight tended to decrease in all groups, suggesting lipolysis in WAT may be related to induction of steatosis in the mice treated MCD diet for 3 weeks. The weight ratio of kidney-to-body (Figure 2C) and BAT-to-body (Figure 2F) was not changed by MCD diet-treatment in all mice.
To evaluate the accumulation of triglycerides and lipids, liver sections were stained with Oil red O, a fat-soluble dye (Figure 3). As we expected, we observed that the supplementation of MCD diet induced dramatic lipid accumulation with mostly a macrovesicular type in the liver and there was no significant difference among the source of mice.
Serum activities of ALT (Figure 4A) and AST (Figure 4B), indicators of liver injury, were dramatically increased by the supplementation of MCD diet for 3 weeks. The MCD diet significantly decreased serum cholesterol (Figure 4C) and triglyceride (Figure 4D) levels in the mice of all sources, which was presumably attributable to a defect in hepatic secretion of triglycerides.
NAFLD, the hepatic manifestation of metabolic syndrome, is an emerging global public health issue due to its high prevalence and characteristic disease progression [22]. Clinical studies have showed that 10-20% of NAFLD patients have NASH, and 10-15% of NASH patients eventually progress into liver cirrhosis via hepatic fibrosis and even into hepatocellular carcinoma [222324]. Despite a growing understanding of the global NAFLD epidemic, there is no definite pharmacotherapy available and a better understanding of its pathophysiology will help in the development of potential therapies. Currently, various animal models of NAFLD such as genetic, dietary, and combination models are used in the laboratory [25]. Although it is necessary to reflect histopathology and pathophysiology of human disease in these models, they have limitations, and specific advantages and disadvantages.
MCD diets have been used for over 40 years to study NAFLD. Animals fed an MCD diet develop remarkable lipid accumulation in the liver by 2-4 weeks and further progress to inflammation and fibrosis shortly thereafter [2627]. The mechanism for steatosis on a MCD diet appears to be decreased mitochondrial oxidation of fatty acids and decreased export of fatty acids in the form of very low density lipoprotein due to lack of phosphatidylcholine synthesis [2829]. Importantly, unlike human or other diet-induced rodent models of NAFLD, MCD diet models lead to weight loss and no insulin resistance [630]. Although this contrasts to the typical human with NAFLD, who presents as obese and insulin resistant, MCD diet model has the advantage of being more efficient and reproducible for inducing NAFLD. Moreover, it is suggested that the MCD diet is a better model to study the pathobiological mechanisms resulting in human NAFLD compared with western diet models [31].
Inbred strains are an interesting and useful animal resource with relevance in several research fields. These animal models are commonly involved in biomedical and behavioral experiments, and they have contributed substantially to the knowledge and understanding of multiple biological mechanisms and metabolic pathways [323334]. Specifically, the C57BL/6 mouse is widely employed in metabolic research including diabetes, obesity, and NAFLD [353637] and it was proved that this strain developed the most inflammation and necrosis, and best approximated the histological features of NAFLD spectrum [6]. Recently, the National Institute of Food and Drug Safety Evaluation (NIFDS) in Korea has an established C57BL6/N mice named “Korl:C57BL6/N” in the Guidelines for Nomenclature of Mouse Strains [38].
The purpose of this study was to characterize C57BL6/NKorl in NAFLD and characterize its utility in the research of pathogenesis and preclinical drug development. It was performed using one of the most common dietary models, MCD diet-induced NAFLD, and the focus was on the general physiological, biochemical, and histopathological changes. We determined the changes in body weight, food consumption, and relative weights of tissues such as liver, kidney, gonadal white adipose tissue, inguinal white adipose tissue, and brown adipose tissue. These basic parameters of mice with an MCD diet for 3 weeks showed no significant difference between origins. Histopathological analysis showed that the MCD diet for 3 weeks induced clear fat vacuoles involving most area of the acinus in the liver of all mice. Severe lipid accumulation in the liver was accompanied by increased serum activities of alanine aminotransferase and aspartate aminotransferase, and decreased serum levels of triglyceride and cholesterol. In conclusion, the response of C57BL6N mice originated from different sources on MCD diet is no significant difference evidenced by physiological, biochemical, and histopathological parameters.
Figures and Tables
![]() | Figure 1Changes in body weight (A) and food consumption (B) of mice originating from different sources following 3 weeks of the MCD diet. “C57BL/6NKorl” from the National Institute of Food and Drug Safety Evaluation in Korea; “A:C57BL/6N” from vendor in the United States; “B:C57BL/6N” from vendor in Japan. |
![]() | Figure 2Effect of MCD diet for 3 weeks on the body weight (A) and relative weights of tissues including liver (B), kidney (C), gWAT (D), iWAT (E), and BAT (F) of mice originating from three different sources. *, **, *** Significantly different from the corresponding control mice (ANOVA followed by Newman-Keuls multiple range test, P<0.05, 0.01, 0.001, respectively). “C57BL/6NKorl” from the National Institute of Food and Drug Safety Evaluation in Korea; “A:C57BL/6N” from vendor in the United States; “B:C57BL/6N” from vendor in Japan. |
![]() | Figure 3Effect of MCD diet for 3 weeks on the lipid accumulation in the liver of mice originating from three different sources. It was accomplished by staining with Oil red O. 40X magnification. “C57BL/6NKorl” from the National Institute of Food and Drug Safety Evaluation in Korea; “A:C57BL/6N” from vendor in the United States; “B:C57BL/6N” from vendor in Japan. |
![]() | Figure 4Effect of MCD diet for 3 weeks on the activities of (A) alanine aminotransferase (ALT) and (B) aspartate aminotransferase (AST), and the levels of (C) total cholesterol and (D) triglycerides in the serum of mice originating from three different sources. **, *** Significantly different from the corresponding control mice (ANOVA followed by Newman-Keuls multiple range test, P<0.05, 0.01, 0.001, respectively). “C57BL/6NKorl” from the National Institute of Food and Drug Safety Evaluation in Korea; “A:C57BL/6N” from vendor in the United States; “B:C57BL/6N” from vendor in Japan. |
Table 1
Composition of the MCD diet
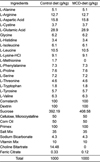
Acknowledgments
This project was supported by a grant of BIOREIN (Laboratory Animal Bio Resources Initiative) from Ministry of Food and Drug Safety in 2015.
References
1. Loomba R, Sanyal AJ. The global NAFLD epidemic. Nat Rev Gastroenterol Hepatol. 2013; 10(11):686–690.
2. Chalasani N, Younossi Z, Lavine JE, Diehl AM, Brunt EM, Cusi K, Charlton M, Sanyal AJ; American Gastroenterological Association; American Association for the Study of Liver Diseases; American College of Gastroenterologyh. The diagnosis and management of non-alcoholic fatty liver disease: practice guideline by the American Gastroenterological Association, American Association for the Study of Liver Diseases, and American College of Gastroenterology. Gastroenterology. 2012; 142(7):1592–1609.
3. Browning JD, Horton JD. Molecular mediators of hepatic steatosis and liver injury. J Clin Invest. 2004; 114(2):147–152.
4. Cohen JC, Horton JD, Hobbs HH. Human fatty liver disease: old questions and new insights. Science. 2011; 332(6037):1519–1523.
5. Day CP, James OF. Steatohepatitis: a tale of two “hits”? Gastroenterology. 1998; 114(4):842–845.
6. Kirsch R, Clarkson V, Shephard EG, Marais DA, Jaffer MA, Woodburne VE, Kirsch RE, Hall Pde L. Rodent nutritional model of non-alcoholic steatohepatitis: species, strain and sex difference studies. J Gastroenterol Hepatol. 2003; 18(11):1272–1282.
7. Leclercq IA, Farrell GC, Field J, Bell DR, Gonzalez FJ, Robertson GR. CYP2E1 and CYP4A as microsomal catalysts of lipid peroxides in murine nonalcoholic steatohepatitis. J Clin Invest. 2000; 105(8):1067–1075.
8. Bothe GW, Bolivar VJ, Vedder MJ, Geistfeld JG. Genetic and behavioral differences among five inbred mouse strains commonly used in the production of transgenic and knockout mice. Genes Brain Behav. 2004; 3(3):149–157.
9. Bryant CD, Zhang NN, Sokoloff G, Fanselow MS, Ennes HS, Palmer AA, McRoberts JA. Behavioral differences among C57BL/6 substrains: implications for transgenic and knockout studies. J Neurogenet. 2008; 22(4):315–331.
10. Mulligan MK, Ponomarev I, Boehm SL 2nd, Owen JA, Levin PS, Berman AE, Blednov YA, Crabbe JC, Williams RW, Miles MF, Bergeson SE. Alcohol trait and transcriptional genomic analysis of C57BL/6 substrains. Genes Brain Behav. 2008; 7(6):677–689.
11. Mekada K, Abe K, Murakami A, Nakamura S, Nakata H, Moriwaki K, Obata Y, Yoshiki A. Genetic differences among C57BL/6 substrains. Exp Anim. 2009; 58(2):141–149.
12. Zurita E, Chagoyen M, Cantero M, Alonso R, González-Neira A, López-Jiménez A, López-Moreno JA, Landel CP, Benítez J, Pazos F, Montoliu L. Genetic polymorphisms among C57BL/6 mouse inbred strains. Transgenic Res. 2011; 20(3):481–489.
13. Itagaki H, Shimizu K, Morikawa S, Ogawa K, Ezaki T. Morphological and functional characterization of non-alcoholic fatty liver disease induced by a methionine-choline-deficient diet in C57BL/6 mice. Int J Clin Exp Pathol. 2013; 6(12):2683–2696.
14. Machado MV, Cortez-Pinto H. Non-alcoholic fatty liver disease: what the clinician needs to know. World J Gastroenterol. 2014; 20(36):12956–12980.
15. Rinella ME, Elias MS, Smolak RR, Fu T, Borensztajn J, Green RM. Mechanisms of hepatic steatosis in mice fed a lipogenic methionine choline-deficient diet. J Lipid Res. 2008; 49(5):1068–1076.
16. Yao ZM, Vance DE. The active synthesis of phosphatidylcholine is required for very low density lipoprotein secretion from rat hepatocytes. J Biol Chem. 1988; 263(6):2998–3004.
17. Caballero F, Fernández A, Matías N, Martínez L, Fucho R, Elena M, Caballeria J, Morales A, Fernández-Checa JC, García-Ruiz C. Specific contribution of methionine and choline in nutritional nonalcoholic steatohepatitis: impact on mitochondrial S-adenosyl-L-methionine and glutathione. J Biol Chem. 2010; 285(24):18528–18536.
18. REITMAN S, FRANKEL S. A colorimetric method for the determination of serum glutamic oxalacetic and glutamic pyruvic transaminases. Am J Clin Pathol. 1957; 28(1):56–63.
19. Wei E, Ben Ali Y, Lyon J, Wang H, Nelson R, Dolinsky VW, Dyck JR, Mitchell G, Korbutt GS, Lehner R. Loss of TGH/Ces3 in mice decreases blood lipids, improves glucose tolerance, and increases energy expenditure. Cell Metab. 2010; 11(3):183–193.
20. Kim SN, Jung YS, Kwon HJ, Seong JK, Granneman JG, Lee YH. Sex differences in sympathetic innervation and browning of white adipose tissue of mice. Biol Sex Differ. 2016; 7:67.
21. Tanaka N, Takahashi S, Fang ZZ, Matsubara T, Krausz KW, Qu A, Gonzalez FJ. Role of white adipose lipolysis in the development of NASH induced by methionine- and cholinedeficient diet. Biochim Biophys Acta. 2014; 1841(11):1596–1607.
22. Day CP. Non-alcoholic fatty liver disease: a massive problem. Clin Med (Lond). 2011; 11(2):176–178.
23. Ekstedt M, Franzén LE, Mathiesen UL, Thorelius L, Holmqvist M, Bodemar G, Kechagias S. Long-term follow-up of patients with NAFLD and elevated liver enzymes. Hepatology. 2006; 44(4):865–873.
24. Sanyal AJ, Brunt EM, Kleiner DE, Kowdley KV, Chalasani N, Lavine JE, Ratziu V, McCullough A. Endpoints and clinical trial design for nonalcoholic steatohepatitis. Hepatology. 2011; 54(1):344–353.
25. Takahashi Y, Soejima Y, Fukusato T. Animal models of nonalcoholic fatty liver disease/nonalcoholic steatohepatitis. World J Gastroenterol. 2012; 18(19):2300–2308.
26. Sahai A, Malladi P, Melin-Aldana H, Green RM, Whitington PF. Upregulation of osteopontin expression is involved in the development of nonalcoholic steatohepatitis in a dietary murine model. Am J Physiol Gastrointest Liver Physiol. 2004; 287(1):G264–G273.
27. Weltman MD, Farrell GC, Liddle C. Increased hepatocyte CYP2E1 expression in a rat nutritional model of hepatic steatosis with inflammation. Gastroenterology. 1996; 111(6):1645–1653.
28. London RM, George J. Pathogenesis of NASH: animal models. Clin Liver Dis. 2007; 11(1):55–74. viii
29. Yao ZM, Vance DE. Reduction in VLDL, but not HDL, in plasma of rats deficient in choline. Biochem Cell Biol. 1990; 68(2):552–558.
30. Rinella ME, Green RM. The methionine-choline deficient dietary model of steatohepatitis does not exhibit insulin resistance. J Hepatol. 2004; 40(1):47–51.
31. Machado MV, Michelotti GA, Xie G, Almeida Pereira T, Boursier J, Bohnic B, Guy CD, Diehl AM. Mouse models of diet-induced nonalcoholic steatohepatitis reproduce the heterogeneity of the human disease. PLoS One. 2015; 10(5):e0127991.
32. Mashimo T, Serikawa T. Rat resources in biomedical research. Curr Pharm Biotechnol. 2009; 10(2):214–220.
33. Croy BA. The 1999 Reginald Thomson Lecture. Custom-built mice: unique discovery tools in biomedical research. Can Vet J. 2000; 41(3):201–206.
34. Ardaillou R. Transgenic mice: a major advance in biomedical research. Bull Acad Natl Med. 2009; 193(8):1773–1782.
35. Fontaine DA, Davis DB. Attention to Background Strain Is Essential for Metabolic Research: C57BL/6 and the International Knockout Mouse Consortium. Diabetes. 2016; 65(1):25–33.
36. Collins S, Martin TL, Surwit RS, Robidoux J. Genetic vulnerability to diet-induced obesity in the C57BL/6J mouse: physiological and molecular characteristics. Physiol Behav. 2004; 81(2):243–248.
37. Winzell MS, Ahrén B. The high-fat diet-fed mouse: a model for studying mechanisms and treatment of impaired glucose tolerance and type 2 diabetes. Diabetes. 2004; 53:Suppl 3. S215–S219.
38. Sundberg JP, Schofield PN. Commentary: mouse genetic nomenclature. Standardization of strain, gene, and protein symbols. Vet Pathol. 2010; 47(6):1100–1104.